- •
Traditional biomarkers are not very useful in bronchopulmonary dysplasia (BPD) as the pathogenesis of BPD is multifactorial and the clinical phenotype is variable.
- •
Novel systems biology–based “-omic” approaches (genomics, proteomics, metabolomics, and microbiomics) may help determine biomarkers associated with abnormal lung development in BPD.
- •
Identification of the genomic determinants of BPD has proven difficult, despite genomewide analyses of single-nucleotide polymorphisms and whole-exome sequencing as well as analyses of the epigenome, perhaps owing to clinical heterogeneity as well as differences in ancestry.
- •
Analyses of transcriptomic differences associated with BPD require evaluation of differences in messenger ribonucleic acid (RNA), microRNA, and long noncoding RNA, although changes observed in the peripheral blood may not necessarily reflect those in tracheal aspirates or lung tissue.
- •
Proteomic differences in the tracheal aspirate and urine have been found to be associated with gestational age at birth and severity of BPD.
- •
Metabolomic differences in the amniotic fluid and tracheal aspirates have been reported among infants who did or did not develop BPD. Metabolomic differences in exhaled breath condensates of long-term survivors of BPD, compared with healthy individuals, suggest persistence of metabolic abnormalities.
- •
All infants, whether born at term or extremely preterm, have a diverse airway microbiome at birth that is dysregulated (e.g., reduced lactobacilli) in infants who later develop BPD.
- •
Detailed data collection of clinical variables for improved disease phenotyping, in addition to careful determination of unbiased, specific, temporal, “-omic” biomarkers may be necessary for a personalized medicine approach to BPD.
Acknowledgments
Supported in part by funding from the National Institutes of Health (NIH) (grant numbers U01 HL122626; R01 HD067126; R01 HD066982; and U10 HD34216).
Since the original description of bronchopulmonary dysplasia (BPD) in late preterm infants by Northway et al., advances in neonatal care have occurred and BPD is now mostly seen in extremely premature infants. Although the definition of BPD has evolved over the past few decades, it remains an operational definition, with some functional indication of the actual magnitude of lung disease, but with limited correlation to the underlying structural or molecular cardiopulmonary pathophysiology. For example, severe BPD markedly differs from that of mild or moderate disease, both in terms of clinical phenotype as well as in terms of genetic predisposition, rather than just being on the extreme end of the spectrum of the clinical operational definition.
A focus on specific genes, proteins, or metabolites does not necessarily enable a deeper understanding of the “holistic” nature of normal lung development in which many different cell types have specific patterns of expression and regulation of multiple genes, proteins, and metabolites, nor does it indicate how these processes are impaired during BPD. The use of newer systems biology approaches is warranted, as they allow an understanding of the phenotypes (the type of “forest”) emerging from various components (trees, and so on) and interactions (of the biological community with the environment) that constitute the (eco)system. This chapter discusses the various new “-omic” system biology approaches that may be available in the future for evaluating the diagnosis, prognosis, and treatment responses of BPD ( Fig. 4.1 ).

-Omics of Disease Processes
Newer technologies have facilitated the gathering of large data sets, often through high-throughput assays, with subsequent analyses of these data sets through bioinformatic methods. In these studies, the researcher obtains a very highly detailed dataset of the changes that occur in response to a defined perturbation, such as the induction of a normal or diseased state. These large-scale data sets on the genome, proteome, metabolome, microbiome, and so forth are often referred to as -omic data sets. An advantage of these technologies is that they can be used with samples (blood, tracheal aspirates [TA], urine, and so on) that can be collected using minimally invasive or noninvasive methods, which enables serial sampling for longitudinal analyses for evaluation of normal or abnormal growth and development. The analytical approaches involved are not driven by any a priori hypotheses, thus permitting unbiased -omic pattern characteristics of a given pathologic condition to be identified, followed by recognition of potential targets in the disease profile, and eventually to formulate hypothesis for diagnostics or therapy development. We have described the utility of these newer -omic methods in relation to BPD in some of our previous publications. A better understanding of the -omic data related to BPD may provide insight not only into the predisposition to BPD (e.g., from genomic data) but also into the pathogenesis of disease (e.g., gene expression, proteomic, or metabolomic data from TA, blood, or lung tissue from individuals with and without BPD), and prediction of therapeutic response (e.g., change in -omic biomarkers with initiation of specific therapies).
Genomics
Advances in the understanding of the human genome sequence and the large-scale generation of data sets have added a new dimension to biomedical research. The role of genetics in BPD was initially proposed by Parker et al., who found a high concordance in 108 twin pairs with birth weights less than 1500 g. This first study confirming and quantifying the genetic contribution to BPD has been independently ratified. We recently published a detailed review summarizing the studies on genetic predisposition to BPD. Genomic variants predisposing to BPD may be single-nucleotide polymorphisms (SNPs), which may increase susceptibility to the disease. Hadchouel et al. conducted a genome-wide analysis and identified SPOCK2 as a new possible candidate susceptibility gene for BPD, but this target was not confirmed in a recent study by Wang et al. or Ambalavanan et al. Multivariate logistic regression showed that the polymorphism vascular endothelial growth factor (VEGF)-634G>C allele was an independent risk factor for BPD in a Japanese cohort of infants. However, polymorphisms in the VEGF and VEGF receptor 2 (VEGFR2) genes have not been consistently associated with BPD in other populations.
Because single-marker approaches might not explain more than a small fraction of heritability of BPD, an integrated genomic analysis was recently conducted by the Eunice Kennedy Shriver National Institutes of Child Health and Human Development Neonatal Research Network (NICHD NRN). Using a DNA repository of extremely low-birth-weight infants, a genome-wide scan was conducted of 1.2 million genotyped SNPs and an additional 7 million imputed SNPs followed by genome-wide association and gene set analysis for BPD or death, severe BPD or death, and severe BPD in survivors. Known pathways of lung development and repair (the cell-surface glycoprotein CD44, phosphorus oxygen lyase activity) and novel molecules and pathways (adenosine deaminase, targets of micro–ribonucleic acid [RNA; miR-219] were found to be involved in the genetic predisposition to BPD. The racial and ethnic differences found in the pathways involved in BPD further highlighted the underlying genetic predisposition of the disease. Other studies using gene expression profiling, such as those by Bhattacharya et al. and Pietrzyk et al. also identified different pathways associated with the genetic origins of BPD. In a recent study, whole-exome sequencing was performed to identify uncommon variants in patients with BPD. The top candidate genes highlighted in this study were nitric oxide synthase 2, matrix metalloproteinase 1, C-reactive protein, lipopolysaccharide-binding protein, and the toll-like receptor family. In another study, authors performed exome sequencing on 50 BPD-affected and unaffected twin pairs using DNA isolated from neonatal blood spots and identified genes affected by extremely rare nonsynonymous mutations. The genes identified were highly enriched for processes involved in pulmonary structure and function, including collagen fibril organization, morphogenesis of embryonic epithelium, and regulation of the Wnt signaling pathway.
In addition to the genome, major epigenetic mechanisms such as DNA methylation may regulate coordinated temporal expression of multiple genes during lung development. Cuna et al. recently conducted a study to identify genes regulated by methylation during normal septation in mice and during disordered septation in BPD. Microarray analysis of gene expression and immunoprecipitation of methylated DNA followed by sequencing was done in mouse lungs, and microarray gene expression data were integrated with genome-wide DNA methylation data from human lungs (BPD vs. preterm or term lung). The authors found that changes in methylation corresponded to altered expression of several genes associated with lung development, suggesting that DNA methylation of these genes may regulate normal and abnormal alveolar septation. Genes known to be important in lung development ( Wnt signaling, Angpt2 , Sox9 , and so on) and its extracellular matrix ( Tnc , Eln , and so on), and genes involved with immune and antioxidant defense ( Stat4 , Sod3 , Prdx6 , and so on) were observed to be differentially methylated in mice. In humans, genes including detoxifying enzymes ( Gstm3 ) and transforming growth factor beta signaling (bone morphogenetic protein 7 [ Bmp7 ]) were differentially methylated with reciprocal changes in expression in BPD compared with preterm or term lung. Significant overlap in genes methylated during mouse and human lung development and with development of BPD strongly suggests the role of DNA methylation in normal and abnormal alveolar septation.
It still remains to be ascertained how different patient characteristics and clinical practices modulate the genetic or epigenetic influences on BPD across different populations. More multicenter human studies are needed to account for clinical heterogeneity, as well as center differences in the incidence of BPD.
Transcriptomics
Transcriptomics is the study of the transcriptome, the complete set of RNA transcripts produced by the genome, using high-throughput methods. High-throughput transcriptomics became possible with microarrays, which are particularly useful for analyzing large mammalian transcriptomes, but they detect only known sequences included on the array. In recent years, RNA sequencing methods have been developed that can evaluate protein-coding mRNA, long noncoding RNA (lncRNA), transcripts of pseudogenes, and miRNA. Bhattacharya et al. conducted an RNA sequencing study using the rodent model of BPD in which expression patterns for selected genes were validated by quantitative polymerase chain reaction followed by mechanistic testing. In this study, the canonical pathways dysregulated in hyperoxia included nuclear factor (erythryoid-derived 2)-like 2 (Nrf2)-mediated oxidative stress signaling, p53 signaling, endothelial nitric oxide synthase signaling, and aryl hydrocarbon receptor (Ahr) pathways. Further cluster analysis identified cyclin D1, cyclin-dependent kinase inhibitor 1A, and Ahr as critical regulatory nodes in the response to hyperoxia, with Ahr serving as the major effector node. Other investigators have used hyperoxia-exposed Nrf2-null mutant newborn mice, along with transcriptomic analyses, to identify the effector molecules of lung injury. Salaets et al. conducted RNA sequencing of preterm rabbit lungs after 7 days of hyperoxia exposure and identified changes in inflammatory, oxidative stress, and lung developmental pathways. In a recent study using human lung tissue, Kho et al. analyzed whole-lung transcriptome profiles of 61 females and 78 males at 54 to 127 days after conception from nonsmoking mothers, using unsupervised principal component analysis and supervised linear regression models. This study using banked human fetal lung tissue found postmenstrual age to be a more dominant factor than sex on the effects of early fetal lung development or disease risk. A limitation of this study was the inability to adjust for differences in race and potential confounders such as in utero exposures owing to limited availability of phenotypic information.
Recently investigators, using a model of mouse lung injury that mimicked human BPD, noted that 882 lncRNAs were upregulated and 887 lncRNAs were downregulated in BPD lung tissues. Further analyses revealed that a downregulated lncRNA—namely, AK033210—associated with tenascin C may be involved in the pathogenesis of BPD.
MicroRNAs have been seen to be dysregulated in multiple disorders and are now being studied in lung biology. Several miRs have been shown to play a role in branching morphogenesis, a key step in early lung development. The current evidence of the role of miRs in late lung development and BPD has been summarized by Nardiello and Morty. We recently highlighted the role of miR-489 in guiding alveolar septation by modulation of its target genes insulin-like growth factor and tenascin C. As mentioned earlier, in the integrated genomic analyses conducted by the NICHD NRN, the pathway with the lowest false discovery rate for BPD/death was the one targeting miR-219. Efforts are needed to develop sophisticated computational methods for the integration of microRNA, microRNA targets, transcription factors, and other important components of the transcriptome into biologically relevant networks, in the context of BPD.
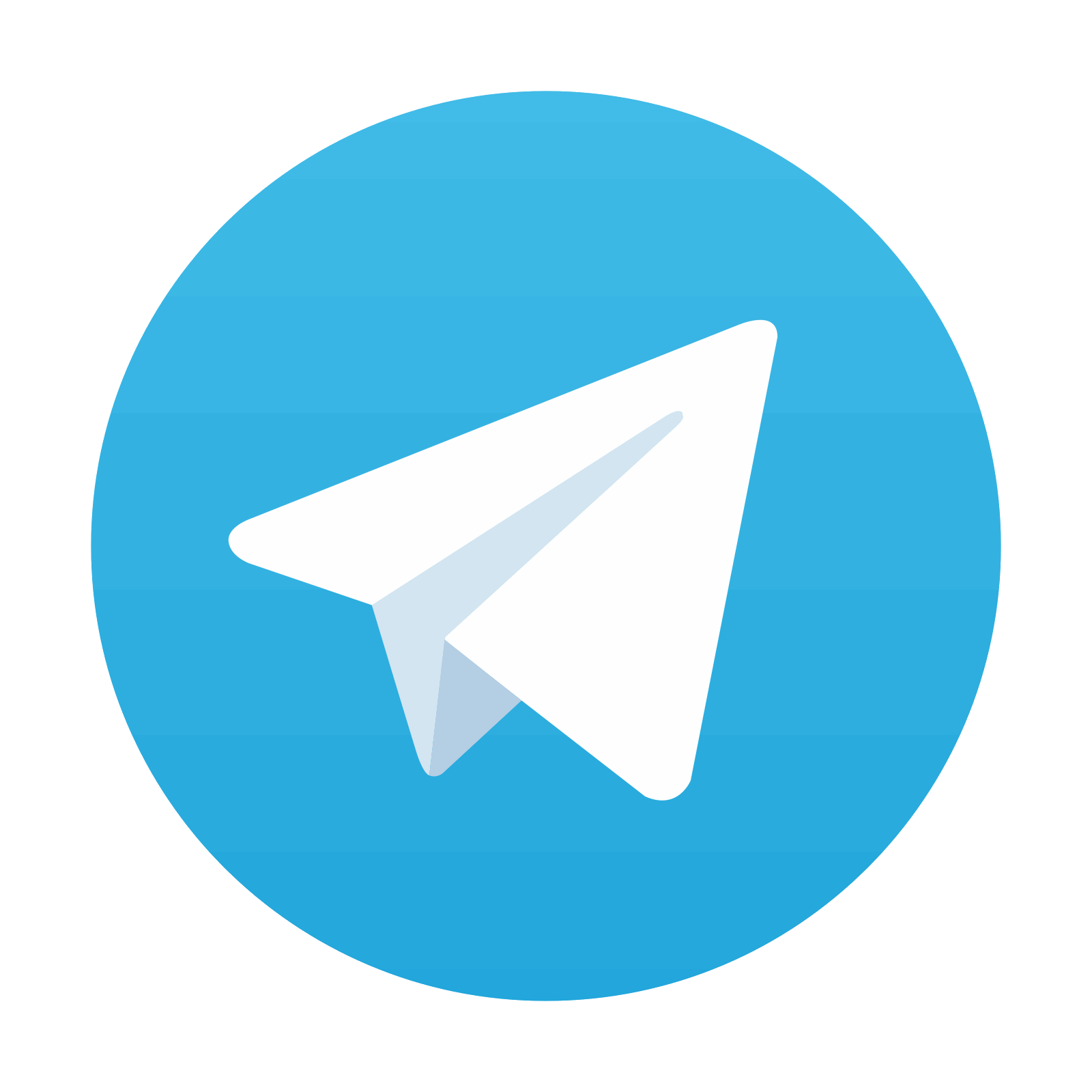
Stay updated, free articles. Join our Telegram channel

Full access? Get Clinical Tree
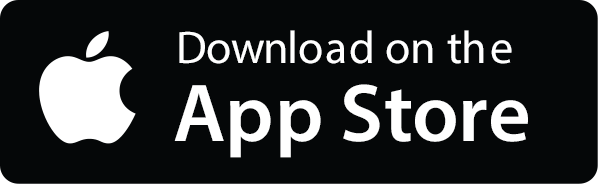
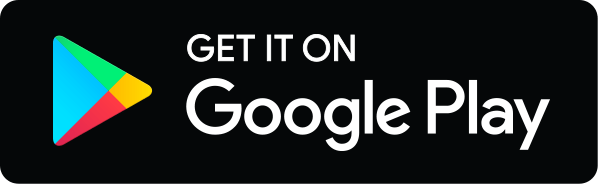