Immediate Care
Before and during delivery, careful consideration must be given to several determinants of neonatal well-being including: (1) maternal health status; (2) prenatal complications, including any suspected fetal malformations; (3) gestational age; (4) labor complications; (5) duration of labor and ruptured membranes; (6) type and duration of anesthesia; (7) difficulty with delivery; and (8) medications given during labor and their dosages, administration routes, and timing relative to delivery.
Newborn Resuscitation
The International Liaison Committee on Resuscitation (ILCOR) updated its guidelines for neonatal resuscitation that are sanctioned by the American Academy of Pediatrics and the American Heart Association (Biban, 2011; Perlman, 2010). These substantially revised guidelines are incorporated into the following sections.
Approximately 10 percent of newborns require some degree of active resuscitation to stimulate breathing, and 1 percent require extensive resuscitation. It is perhaps not coincidental that there is a two- to threefold risk of death for newborns delivered at home compared with those delivered in hospitals (American College of Obstetricians and Gynecologists, 2013b). When deprived of oxygen, either before or after birth, neonates demonstrate a well-defined sequence of events leading to apnea (Fig. 32-1). With oxygen deprivation, there is a transient period of rapid breathing, and if it persists, breathing stops, which is termed primary apnea. This stage is accompanied by a fall in heart rate and loss of neuromuscular tone. Simple stimulation and exposure to oxygen will usually reverse primary apnea. If oxygen deprivation and asphyxia persist, however, the newborn will develop deep gasping respirations, followed by secondary apnea. This latter stage is associated with a further decline in heart rate, falling blood pressure, and loss of neuromuscular tone. Neonates in secondary apnea will not respond to stimulation and will not spontaneously resume respiratory efforts. Unless ventilation is assisted, death follows. Clinically, primary and secondary apneas are indistinguishable. Thus, secondary apnea must be assumed and resuscitation of the apneic newborn must be started immediately.
FIGURE 32-1 Physiological changes associated with primary and secondary apnea in the newborn. bpm = beats per minute; HR = heart rate; MAP = mean arterial pressure. (Adapted from Kattwinkel, 2006.)
Resuscitation Protocol
The updated algorithm for newborn resuscitation recommended by ILCOR and the International Consensus on Cardiopulmonary Resuscitation is shown in Figure 32-2. Many of its tenets follow below.
FIGURE 32-2 Algorithm for resuscitation of the newborn. CPAP = continuous positive airway pressure; HR = heart rate; IV = intravenous. (Adapted from Perlman, 2010.)
Basic Measures
The vigorous newborn is first placed in a warm environment to minimize heat loss, the airway is cleared, and the infant dried. Routine gastric aspiration has been shown to be nonbeneficial and even harmful (Kiremitci, 2011). And although previously recommended, there is no evidence that bulb suctioning for clear or meconium-stained fluid is beneficial, even if the newborn is depressed (Chap. 33, p. 638). With stimulation, the healthy newborn will take a breath within a few seconds of birth and cry within half a minute, after which routine supportive care is provided.
Assessment at 30 Seconds of Life. Apnea, gasping respirations, or heart rate < 100 bpm beyond 30 seconds after delivery should prompt administration of positive-pressure ventilation with room air (Fig. 32-3). Assisted ventilation rates of 30 to 60 breaths per minute are commonly employed, and the percent of oxygen saturation is monitored by pulse oximetry. At this point, supplemental oxygen can be given in graduated increasing percentages to maintain oxygen saturation (Spo2) values within a normal range (Vento, 2011). Adequate ventilation is indicated by improved heart rate.
FIGURE 32-3 Correct use of bag-and-mask ventilation. The head should be in a sniffing position with the tip of the nose pointing to the ceiling. The neck should not be hyperextended.
Assessment at 60 Seconds of Life. If the heart rate remains < 100 bpm, then ventilation is inadequate. The head position should be checked as shown in Figure 32-3, secretions cleared, and if necessary, inflation pressure increased. If the heart rate persists below 100 bpm beyond 60 seconds, tracheal intubation is considered. A number of conditions may be the cause of inadequate response, including the following:
• Hypoxemia or acidosis from any cause
• Drugs administered to the mother before delivery
• Immaturity
• Upper airway obstruction
• Pneumothorax
• Lung abnormalities
• Meconium aspiration
• Central nervous system developmental abnormality
• Sepsis syndrome.
Tracheal Intubation
If bag-and-mask ventilation is ineffective or prolonged, tracheal intubation is then performed. Other indications include the need for chest compressions or tracheal administration of medications, or special circumstances such as extremely low birthweight or a congenital diaphragmatic hernia. A laryngoscope with a straight blade—size 0 for a preterm infant and size 1 for a term neonate—is introduced at the side of the mouth and then directed posteriorly toward the oropharynx as shown in Figure 32-4. The laryngoscope is next moved gently into the vallecula—the space between the base of the tongue and the epiglottis. Gentle elevation of the laryngoscope tip will raise the epiglottis and expose the glottis and the vocal cords. The tube is then introduced through the vocal cords. Gentle cricoid pressure may be useful. Tube sizes vary from 3.5 to 4.0 mm for term infants down to 2.5 mm for those < 28 weeks or < 1000 g.
FIGURE 32-4 Sagittal view of laryngoscope positioning during intubation. The laryngoscope blade is inserted between the tongue base and epiglottis. Upward tilting of the tongue also lifts the epiglottis. The endotracheal tube is then threaded below the epiglottis and between the vocal cords (inset) to enter the trachea.
Several steps are taken to ensure that the tube is positioned in the trachea and not the esophagus: observation for symmetrical chest wall motion; auscultation for equal breath sounds, especially in the axillae; and auscultation for the absence of breath sounds or gurgling over the stomach. Tracheal suctioning is no longer recommended or discouraged. Using an appropriate ventilation bag attached to the tracheal tube, puffs of air are delivered into the tube at 1- to 2-second intervals with a force adequate to gently lift the chest wall. In term infants, pressures of 30 to 40 cm H2O typically will expand the alveoli without causing barotrauma. For preterm infants, pressures are 20 to 25 cm H2O. An increase in heart rate and Spo2 levels within acceptable ranges reflect a positive response.
Chest Compressions
If the heart rate remains < 60 bpm despite adequate ventilation for 30 seconds, chest compressions are initiated. These are delivered on the lower third of the sternum at a depth sufficient to generate a palpable pulse. A 3:1 compressions-to-ventilation ratio is recommended, with 90 compressions and 30 breaths to achieve approximately 120 events each minute. The heart rate is reassessed every 30 seconds, and chest compressions are continued until the spontaneous heart rate is at least 60 bpm.
Epinephrine and Volume Expansion
Intravenously administered epinephrine is indicated when the heart rate remains < 60 bpm after adequate ventilation and chest compressions. Epinephrine may be given through the endotracheal tube if venous access has not been estabished. The recommended intravenous dose is 0.01 to 0.03 mg/kg. If given through the tracheal tube, higher doses are employed—0.05 to 0.1 mg/kg.
For infants with blood loss, early volume replacement with crystalloid or packed red cells is indicated if they do not respond to resuscitation.
Discontinuation of Resuscitation
As expected, newborns with cardiopulmonary arrest who do not respond promptly to resuscitation are at great risk for death, and if they survive, for severe morbidity (Haddad, 2000). The International Consensus Committee concluded that discontinuation of resuscitative efforts may be appropriate in a neonate without a heartbeat for 10 minutes of continuous and adequate resuscitative efforts (Perlman, 2010). This committee cites lack of data for more specific recommendations regarding the infant whose heart rate remains < 60 bpm.
EVALUATION OF NEWBORN CONDITION
Apgar Score
The scoring system described in 1953 by Dr. Virginia Apgar remains a useful clinical tool to identify those neonates who require resuscitation and to assess the effectiveness of any resuscitative measures. As shown in Table 32-1, each of the five easily identifiable characteristics—heart rate, respiratory effort, muscle tone, reflex irritability, and color—is assessed and assigned a value of 0 to 2. The total score, based on the sum of the five components, is determined in all neonates at 1 and 5 minutes after delivery. In depressed infants, the score may be calculated at further 5-minute intervals until a 20-minute Apgar score is assessed.
TABLE 32-1. Apgar Scoring System
The 1-minute Apgar score reflects the need for immediate resuscitation. The 5-minute score, and particularly the change in score between 1 and 5 minutes, is a useful index of the effectiveness of resuscitative efforts. The 5-minute Apgar score also has prognostic significance for neonatal survival because survival is related closely to the condition of the neonate in the delivery room (Apgar, 1958). In an analysis of more than 150,000 infants delivered at Parkland Hospital, Casey and associates (2001b) assessed the significance of the 5-minute score for predicting survival during the first 28 days of life. They found that in term neonates, the risk of neonatal death was approximately 1 in 5000 for those with Apgar scores of 7 to 10. This risk compares with a mortality rate of 1 in 4 for term infants with 5-minute scores of 3 or less. Low 5-minute scores were comparably predictive of neonatal death in preterm infants. These investigators concluded that the Apgar scoring system is as relevant for the prediction of neonatal survival today as it was more than 50 years ago.
There have been attempts to use Apgar scores to define asphyxial injury and to predict subsequent neurological outcome—uses for which the Apgar score was never intended. Such associations are difficult to measure with reliability given that both asphyxial injury and low Apgar scores are infrequent outcomes. For example, according to United States birth certificate records for 2010, only 1.8 percent of newborns had a 5-minute score below 7 (Martin, 2012). Similarly, in a population-based study of more than 1 million term infants born in Sweden between 1988 and 1997, the incidence of 5-minute Apgar scores of 3 or less was approximately 2 per 1000 (Thorngren-Jerneck, 2001).
Despite the methodological challenges, erroneous definitions of asphyxia by many groups were established based solely on low Apgar scores. These prompted the American College of Obstetricians and Gynecologists and the American Academy of Pediatrics (2010) to issue a series of joint opinions with important caveats regarding limitations of use of the Apgar score. One is that because certain elements of the Apgar score are partially dependent on the physiological maturity of the newborn, a healthy preterm infant may receive a low score only because of immaturity (Catlin, 1986). Apgar scores may be influenced by a variety of factors including, but not limited to, fetal malformations, maternal medications, and infection. Therefore, it is inappropriate to use an Apgar score alone to diagnose asphyxia. A 5-minute Apgar score of 3 correlates poorly with adverse future neurological outcomes, and thus scores are measured also at 10, 15, and 20 minutes when the score remains 3 or less (Freeman, 1988; Nelson, 1981).
Importantly, the Apgar score alone cannot establish hypoxia as the cause of cerebral palsy. As discussed in Chapter 33 (p. 638), a neonate who has had an asphyxial insult proximate to delivery that is severe enough to result in acute neurological injury will demonstrate most of the following: (1) profound acidemia with cord artery blood pH < 7 and acid-base deficit ≥ 12 mmol/L; (2) Apgar score of 0–3 persisting for 10 minutes or longer; (3) neurological manifestations such as seizures, coma, or hypotonia; and (4) multisystem organ dysfunction—cardiovascular, gastrointestinal, hematological, pulmonary, or renal (American College of Obstetricians and Gynecologists and the American Academy of Pediatrics, 2003).
Umbilical Cord blood Acid–Base Studies
Blood taken from umbilical vessels may be used for acid-base studies to assess the metabolic status of the neonate. Blood collection is performed following delivery by immediately isolating a 10- to 20-cm segment of cord with two clamps placed near the neonate and another two clamps nearer the placenta. The importance of clamping the cord is underscored by the fact that delays of 20 to 30 seconds can alter both the Pco2 and pH (Valero, 2012; White, 2012). The cord is then cut between the two proximal clamps and then the two distal clamps.
Arterial blood is drawn from the isolated cord segment into a 1- to 2-mL commercially prepared plastic syringe containing lyophilized heparin or a similar syringe that has been flushed with a heparin solution containing 1000 U/mL. Once sampling is completed, the needle is capped and the syringe transported, on ice, to the laboratory. Although efforts should be made for prompt transport, neither the pH nor Pco2 values change significantly in blood kept at room temperature for up to 60 minutes (Duerbeck, 1992). Mathematical models have been developed that allow reasonable prediction of birth acid–base status in properly collected cord blood samples analyzed as late as 60 hours after delivery (Chauhan, 1994). Also, Swedish investigators reported significant variances in acid-base measurements with the use of different analyzers (Mokarami, 2012).
Fetal Acid-Base Physiology
The fetus produces both carbonic and organic acids. Carbonic acid (H2CO3) is formed by oxidative metabolism of CO2. The fetus usually rapidly clears CO2 through the placental circulation. If CO2 clearance is lowered, then carbonic acid levels rise. When H2CO3 accumulates in fetal blood and there is no concurrent increase in organic acids, the result is termed respiratory acidemia. This often follows impaired placental exchange.
In contrast, organic acids primarily include lactic and β-hydroxybutyric acids. Levels of these increase with persistent placental exchange impairment and result from anaerobic glycolysis. These organic acids are cleared slowly from fetal blood, and when they accumulate without a concurrent increase in H2CO3, the result is termed metabolic acidemia. With the development of metabolic acidemia, bicarbonate (HCO3−) decreases because it is used to buffer the organic acid. An increase in H2CO3 accompanied by an increase in organic acid reflected by decreased HCO3− causes mixed respiratory-metabolic acidemia.
In the fetus, respiratory and metabolic acidemia and ultimately tissue acidosis are most likely part of a progressively worsening continuum. This is different from the adult pathophysiology, in which distinct conditions result in either respiratory acidosis—for example, pulmonary disease, or metabolic acidosis—for example, diabetes. In the fetus, the placenta serves as both the lungs and to a certain degree, the kidneys. One principal cause of fetal acidemia is a decrease in uteroplacental perfusion. This results in the retention of CO2, that is, respiratory acidemia, and if protracted and severe enough, a mixed or metabolic acidemia.
Assuming that maternal pH and blood gases are normal, the actual pH of fetal blood is dependent on the proportion of carbonic and organic acids and the amount of bicarbonate, which is the major buffer in blood. This can best be illustrated by the Henderson–Hasselbalch equation:
For clinical purposes, HCO3− represents the metabolic component and is reported in mEq/L. The H2CO3 concentration represents the respiratory component and is reported as the Pco2 in mm Hg. Thus:
The result of this equation is a pH value. Because pH is a logarithmic term, it does not give a linear measure of acid accumulation. For example, a change in hydrogen ion concentration associated with a fall in pH from 7.0 to 6.9 is almost twice that which is associated with a fall in pH from 7.3 to 7.2. For this reason, the change in base, termed delta base, offers a more linear measure of the degree of accumulation of metabolic acid (Armstrong, 2007). The delta base is a calculated number used as a measure of the change in buffering capacity of bicarbonate (HCO3−). The formula for calculating the base excess (BE) is as follows:
BE = 0.02786 × Pco2 × 10(pH – 6.1) × 13.77 × pH – 124.58
Shown in Figure 32-5 is a nomogram developed from which these can be calculated if only two parameters are known. For example, HCO3− concentration will be decreased with a metabolic acidemia as it is consumed to maintain a normal pH. A base deficit occurs when HCO3− concentration decreases to below normal levels, and a base excess occurs when HCO3− values are above normal. Importantly, a mixed respiratory–metabolic acidemia with a large base deficit and a low HCO3−, for example 12 mmol/L, is more often associated with a depressed neonate than is a mixed acidemia with a minimal base deficit and a more nearly normal HCO3− level.
FIGURE 32-5 Nomogram for determining the delta base. (Adapted from Siggaard-Andersen, 1963).
Clinical Significance of Acidemia
Fetal oxygenation and pH generally decline during the course of normal labor. Normal umbilical cord blood pH and blood gas values at delivery in term newborns are summarized in Table 32-2. Similar values have been reported for preterm infants (Dickinson, 1992; Ramin, 1989; Riley, 1993). The lower limits of normal pH in the newborn have been found to range from 7.04 to 7.10 (Boylan, 1994). Thus, these values should be considered to define neonatal acidemia. Even so, most fetuses will tolerate intrapartum acidemia with a pH as low as 7.00 without incurring neurological impairment (Freeman, 1988; Gilstrap, 1989). However, in a study of newborns with a pH < 7.0 from Parkland Hospital, there were inordinate proportions of neonatal deaths—8 percent, intensive-care admission—39 percent, intubations—14 percent, and seizures—13 percent (Goldaber, 1991). And in a study from Oxford of more than 51,000 term infants, the incidence of neonatal encephalopathy with the pH < 7.0 was 3 percent (Yeh, 2012).
TABLE 32-2. Umbilical Cord blood pH and blood Gas Values in Normal Term Newborns
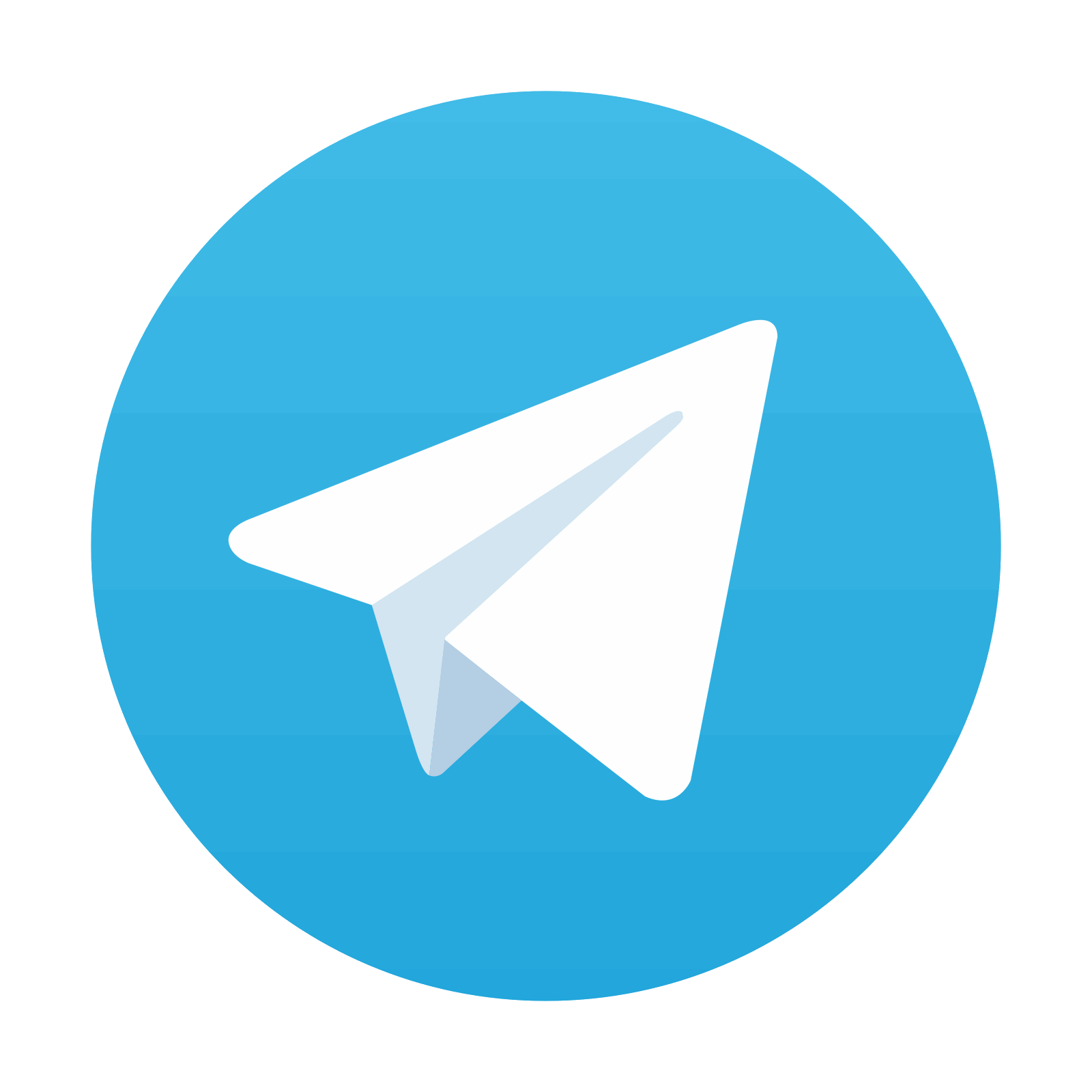
Stay updated, free articles. Join our Telegram channel

Full access? Get Clinical Tree
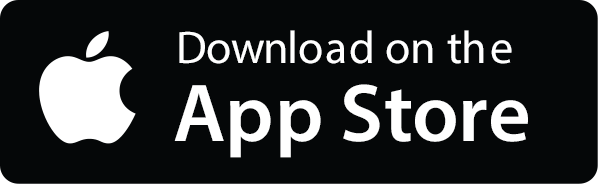
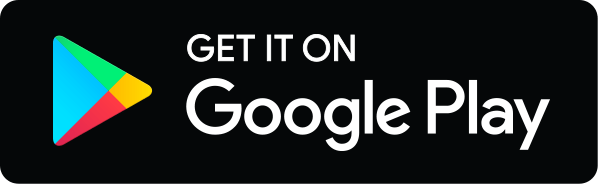