46 William L. Ledger School of Women’s and Children’s Health, University of New South Wales Medicine, New South Wales, Australia The human female is monotocous. Multiple pregnancy is a rare event in our species and frequently leads to complications that, in the days before modern medicine, would have led to maternal or fetal demise. Hence we have evolved a complex and highly regulated sequence of events that exists to ensure that only one oocyte is ovulated in any one cycle. Regular monthly menstruation is an obvious marker that the various levels of interaction between hypothalamus, pituitary, ovary and uterus are functional. Interruption of this axis at any point leads to disordered menses. Gynaecologists will frequently have to investigate and treat such disorders and a clear understanding of the regulation of the normal cycle is therefore necessary in order to guide rational management when things go wrong. The normal menstrual cycle is mostly a reflection of ovarian events. The selection and growth of the dominant follicle leads to increasing concentrations of oestrogens in the blood, stimulating endometrial growth in synchrony with the development of a competent oocyte. Later, following the luteinizing hormone (LH) surge, ovarian oestrogens and progesterone from the corpus luteum induce endometrial secretory changes, and the decline in luteal steroid production in the absence of pregnancy leads to the onset of menstruation. Hence a description of clinical relevance of the menstrual cycle should focus on ovarian physiology, while not overlooking events in the hypothalamus and pituitary and at the level of the uterus. The menstrual cycle is regulated at both endocrine and paracrine levels. Endocrinologically, there are classical feedback loops that modulate release of gonadotrophin hormones from the pituitary, with the ovarian steroids as the afferent arm. More recent studies have begun to elaborate a complex series of paracrine processes that operate within the tissues of the ovary and uterus to impose local regulation. At birth, the human ovaries contain approximately 1 million primordial follicles, arrested at prophase of the first meiotic division. This number already reflects considerable attrition from the maximum size of about 7 million in the follicle ‘pool’ at 5 months of fetal life [1]. Further depletion of the follicle pool will continue throughout reproductive life, with regular escape of follicles from the primordial ‘resting phase’ by re‐entry into meiosis. The process of ‘escape’ from the resting state is not dependent on extra‐ovarian influences: follicle depletion occurs before and after menarche, during use of the oral contraceptive pill and during pregnancy, and whether or not regular menstruation occurs. The majority of follicles will never develop beyond the pre‐antral stage, travelling instead towards atresia. Of the original pool of 7 million primordial follicles, only about 400 will ever acquire gonadotrophin receptors and the possibility of ovulation [2]. This dramatic attrition defines the female arm of natural selection, mirrored by the huge wastage of spermatogenesis in the male, in which millions of sperm are produced each day during fertile life, with only a tiny proportion ever fertilizing an oocyte. The early stages of follicle development in the human are independent of gonadotrophins. Studies using transgenic animal species have begun to elucidate the contribution of locally acting intra‐ovarian paracrine regulators of primordial follicle development, including bone morphogenetic proteins (BMPs), growth differentiation factor (GDF)‐9, anti‐Müllerian hormone (AMH) and the Bax family of regulators of apoptosis (Table 46.1). Table 46.1 Specific gene knockouts and their effects on ovarian function in the mouse. Such studies are of more than theoretical interest: understanding the mechanisms regulating rate of entry into the pool of growing follicles should help to explain such common clinical problems as ‘idiopathic’ premature ovarian failure and early onset of menopause, as well as suggesting means of prolonging the reproductive lifespan. For example, some patients with premature ovarian failure have been found to carry mutations in the BMP15 gene that lead to defective secretion of bioactive BMP15 dimer. BMPs are involved in the earlier stages of egress of follicles from the primordial pool, and such mutations may provide a diagnosis in cases of previously unexplained premature ovarian failure. Once a developing follicle reaches the pre‐antral stage of development (Fig. 46.1), further progression to the antral and preovulatory stages appears to be absolutely dependent on the presence of gonadotrophins. The temporary elevation in circulating concentration of follicle‐stimulating hormone (FSH) seen in the early follicular phase of the ovarian cycle allows a limited number of pre‐antral follicles to reach this stage of maturity, creating a cohort of practically synchronously developing follicles. However, only one ‘lead’ follicle will acquire significant aromatase enzyme activity within its granulosa cells, leading to increased synthesis and secretion of estradiol from androgenic precursors. The ‘two‐cell, two gonadotrophin’ hypothesis specifies the need for both LH, to stimulate production of precursor androgens, particularly androstenedione, by the theca cell layer, and FSH, to drive aromatization to estradiol within the adjacent granulosa cell layer [3]. FSH, LH and human chorionic gonadotrophin (hCG) are structurally similar, sharing an identical α‐subunit. Their specificity lies in structural differences in the β‐subunit (Fig. 46.2). Hence assays for these molecules use antibodies directed against β‐subunit epitopes. Fig. 46.1 The development of a follicle, from (a) primordial, (b) small antral and (c) preovulatory stages. (a) The primordial follicle is surrounded by a single layer of undifferentiated epithelial cells, and is insensitive to gonadotrophins. (b) The early antral follicle has well‐differentiated theca (immunostained brown) and granulosa cell layers surrounding the developing antral cavity with the oocyte. (c) The preovulatory follicle with the oocyte surrounded by the cumulus oophorus with well‐differentiated granulosa and theca cell layers. Fig. 46.2 Molecular structure of FSH, LH and hCG. The necessity for both LH and FSH at this stage of the cycle is demonstrated when exogenous gonadotrophin replacement is given to patients with Kallmann’s syndrome. These patients are unable to secrete gonadotrophins into the circulation, but have normal ovarian physiology. The results of a study of such a patient are shown in Fig. 46.3. The patient had Kallmann’s syndrome with anosmia, primary amenorrhoea and hypogonadotrophic hypogonadism. Ovulation induction was undertaken using two different preparations of gonadotrophin. Treatment with both FSH and LH in the form of human menopausal gonadotrophins (hMG) induces both normal follicle growth, monitored by transvaginal ultrasound (bottom panel), and estradiol secretion (top left panel), leading to high luteal phase progesterone concentrations after an artificial LH surge with hCG injection. This indicates that successful ovulation and luteinization occurred. In contrast, treatment with FSH in the absence of LH, using a recombinant FSH preparation, led to identical follicle development on ultrasound but little elevation in circulating estradiol concentration in the phase of follicular growth and no increase in progesterone after hCG injection. The genetic basis of hypogonadotrophic hypogonadism has recently been partially explained. Study of a patient with Kallman’s syndrome and a deletion on the X chromosome led to the identification of the KAL1 gene as a cause of X‐linked Kallman’s syndrome. More than 10 other gene defects have been identified in cases of hypogonadotrophic hypogonadism. Fig. 46.3 Effects of FSH alone, and FSH and LH in combination on follicle development in a hypogonadotrophic patient with Kallmann’s syndrome. The pituitary secretes the gonadotrophins LH and FSH in response to pulses of gonadotrophin‐releasing hormone (GnRH) from the hypothalamus, which travel to the anterior pituitary via the hypothalamo‐hypophyseal portal tract. LH secretion appears to be closely regulated by GnRH pulsatility, while secretion of FSH is coregulated by hypothalamic GnRH and other factors which act directly on the pituitary, possibly including the inhibins and activins. In the normal follicular phase, GnRH pulse frequency is approximately once per 90 min. GnRH pulses are less frequent in the luteal phase, occurring approximately once in 4 hours. Disorders that slow GnRH pulsatility, such as anorexia nervosa, result in failure of secretion of pituitary gonadotrophins and a state of hypogonadotrophic hypogonadism, with undetectable serum LH and FSH and amenorrhoea. The peptide kisspeptin acts as a potent stimulant to GnRH secretion in the human and mutations in the KISS1 gene leading to loss of kisspeptin signalling have been identified as rare causes of hypogonadotrophic hypogonadism [4]. Central kisspeptin release is also modulated by stress and nutritional status, providing a possible mechanism for ‘hypothalamic’ amenorrhoea in anorexia and in situations of excessive emotional stress. These changes may occur in response to alterations in leptin secretion from adipocytes, leading to kisspeptin‐mediated alterations in pulsatile GnRH secretion. Recently, peripheral administration of synthetic kisspeptin has been shown to be effective in inducing ovulation in hypothalamic amenorrhoea and other therapeutic roles in treatment of infertility are being explored. A second neuropeptide, neurokinin B, is also expressed on GnRH neurones and stimulates GnRH secretion and both kisspeptin and neurokinin B expression are downregulated by oestrogen. Hence it appears likely that kisspeptin and neurokinin B may participate in the relay of feedback from oestrogens, acting at the level of the hypothalamus, to GnRH production, regulating follicle growth and the onset of the LH surge (Fig. 46.4). Fig. 46.4 Hypothalamic–pituitary–gonadal axis. Source: Dr May Wong. Reproduced with permission of Dr May Wong. Once the concentration of serum estradiol begins to rise in the mid‐follicular phase, there is rapid suppression of pituitary FSH production by negative feedback (Fig. 46.5). Recent studies have suggested that suppression of pituitary FSH secretion in the follicular phase might be co‐mediated by rising serum concentrations of inhibin B, a glycoprotein secreted by the granulosa cells of the developing dominant follicle. It is perhaps not surprising that a dual mechanism to control follicular‐phase FSH secretion has evolved, since mono‐ovulation is so essential to healthy reproduction in humans. The resulting decrease in the circulating concentration of FSH withdraws gonadotrophin ‘drive’ from the remainder of the growing cohort of follicles. The result is progression to atresia for all but the dominant follicle, leading to mono‐ovulation [5]. Fig. 46.5 Synchronization of the menstrual and ovarian cycles. Source: Dr May Wong. Reproduced with permission of Dr May Wong. The mechanism by which this selection of a single dominant follicle occurs has been described by the ‘threshold’ concept, in which the rising concentration of FSH exceeds the threshold and thereby opens a ‘window’ that allows one follicle to continue growth and development. Suppression of FSH concentration then closes the window, preventing growth of multiple mature follicles (Fig. 46.6). Fig. 46.6 The ‘threshold concept’ illustrating dependence of advanced follicle growth and maturation on a rise in circulating FSH concentration above an arbitrary threshold, with subsequent suppression of FSH preventing multiple follicle development. Source: Macklon NS, Fauser BC. Follicle‐stimulating hormone and advanced follicle development in the human. Arch Med Res 2001;32:595–600. Reproduced with permission of Elsevier. The threshold concept is useful in understanding the pitfalls of superovulation, in which daily injections of high doses of FSH are given as part of in vitro fertilization (IVF). The aim is to produce a cohort of eight or more mature follicles suitable for ultrasound‐guided oocyte retrieval. However, if the follicle pool is small (e.g. if the patient is nearing menopause), then the yield of mature follicles will be disappointing, while if the follicle pool is large (e.g. if the patient has polycystic ovary syndrome), then there is a danger of over‐response with ovarian hyperstimulation syndrome. The recent introduction of rapid and reliable automated assays for AMH has allowed measurement of this dimeric glycoprotein before starting exogenous gonadotrophin superovulation. Serum AMH is high in patients with polycystic ovary syndrome who are in danger of over‐response and hyperstimulation, and low in patients who are closer to menopause with low ovarian reserve. Hence those women with high AMH are given low doses of gonadotrophins, with careful monitoring, whilst those with low AMH can safely be given higher doses in an attempt to improve oocyte yield. Physiologically, AMH is secreted by small antral follicles and is not seen in large preovulatory follicles. Hence measurement gives a direct assessment of the number of small antral follicles in the developing pool, which in turn depends on the size of the primordial follicle pool remaining in the ovaries. Measurement of AMH can therefore provide an estimate of the size of the remaining pool of follicles in the ovaries. Recent studies have suggested that this can provide a reasonably accurate assessment of a woman’s time to menopause, with clear clinical implications for management of infertility. The serum concentration of AMH varies little across the menstrual cycle and is not significantly affected by use of combined oral contraceptives, facilitating easy measurement in clinical practice. Final maturation of the oocyte only occurs after initiation of the LH surge. This ensures that the oocyte is mature and ready for fertilization only after release from the follicle into the ampulla of the fallopian tube, where fertilization may occur. The LH surge represents a coordinated discharge of LH from the gonadotroph cells of the anterior pituitary. This occurs in response to the rapid rise in estradiol during the latter days of the follicular phase of the ovarian cycle. Pulses of GnRH from the hypothalamus increase in both magnitude and frequency, triggering the LH surge with a rapid outpouring of LH and, to a lesser extent, FSH from the anterior pituitary (see Fig. 46.5). The LH surge is also preceded by a rise in serum concentration of progesterone. The contribution of this rise to the periovulatory phase of the cycle is unclear, but prevention of the preovulatory rise in serum progesterone concentration using progesterone receptor antagonists such as mifepristone prevents efficient ovulation. Compounds with effects similar to mifepristone are being tested as possible contraceptive agents, possibly acting by inhibiting both ovulation and implantation. The LH surge initiates final maturation of the oocyte with completion of meiosis and extrusion of the first polar body, which contains one of the two haploid sets of chromosomes, from the oocyte. The LH surge also induces an inflammatory‐type reaction at the apex of the follicle adjacent to the outer surface of the ovarian cortex. A process of new blood vessel formation, with associated release of prostaglandins and cytokines, leads to rupture of the follicle wall and ovulation about 38 hours after the initiation of the LH surge. A chemotactic effect of ovarian cytokines draws the fimbria of the fallopian tube to within close proximity of the rupturing follicle. A thin mucus strand seems to join the mouth of the fallopian tube to the ovular follicle, forming a bridge for transit of the oocyte into the tube. The ‘empty’ follicle rapidly fills with blood and the theca and granulosa cell layers of the follicle wall luteinize, with formation of the corpus luteum (see Fig. 46.6). A rapid synthesis of progesterone, along with estradiol, follows. Concentrations of progesterone in serum rise rapidly to above 25 nmol/L, one of the highest concentrations seen for any hormone in the circulation. These concentrations rise still further if pregnancy follows. The follicular phase of the ovarian cycle is characterized by the appearance in the circulation of increasing amounts of estradiol. Estradiol acts on the basalis layer of endometrium, which persists from cycle to cycle in contrast to the monthly shedding of the more superficial layers of endometrium. The follicular phase of the ovarian cycle therefore equates to the proliferative phase of the endometrial cycle as proliferative endometrium develops in synchrony with the growth and maturation of the oocyte and its follicle. An organized endometrial architecture appears, with glandular and stromal compartments. The LH surge is followed by luteinization of the ruptured follicle and formation of the corpus luteum, with secretion of large amounts of progesterone. This in turn stimulates the onset of secretion from the endometrial glands, initiating the secretory phase of the endometrial cycle in concert with the luteal phase of the ovarian cycle (Fig. 46.7). Fig. 46.7 The histological appearances of the endometrial cycle showing the menstrual, proliferative and secretory phases. A key element in growth of healthy endometrium is formation of new blood vessels (endometrial angiogenesis), which seems to occur by elongation and expansion from pre‐existing small vessels in the basalis. Endometrial angiogenesis can be divided into three stages: first, during menstruation, to re‐form the vascular bed; second, during the proliferative phase, to develop endometrial vascular supply; and, finally, with spiral arteriole growth and coiling during the secretory phase, with the aim of providing an adequately vascularized site for implantation [6]. Therefore, in contrast to most vascular beds, which keep a persistent structure throughout life, the endometrial vascular network grows and regresses during each menstrual cycle. Numerous angiogenic and angiostatic factors have been identified in the human endometrium. Most of these studies have focused on vascular endothelial growth factor (VEGF) and interleukins, which may be directly responsive to changing concentrations of ovarian steroids. The development of a healthy secretory endometrium is essential for implantation and successful development of pregnancy. In the human, the oocyte is fertilized in the ampullary portion of the fallopian tube and then travels to enter the uterus on day 3, at the morula stage of development. The blastocyst, with distinct trophectoderm and inner cell mass, forms on day 4. The blastocyst sheds the zona pellucida and then adheres to the endometrial epithelium, beginning the process of implantation. Implantation is the first step in the interaction between the cells of the blastocyst and endometrium, i.e. between the mother and the fetus. Hence this interaction is critical to successful pregnancy, and a number of endometrial proteins have been identified as potential regulators of blastocyst development and implantation. These include endometrial integrins, glycosylated cell adhesion molecule (GlyCAM)‐1 and osteopontin [7]. Continuous exposure of the endometrium to progesterone in early pregnancy downregulates progesterone receptors in the epithelia, a process associated with loss of the cell‐surface mucin MUC1 and induction of secreted adhesion proteins. High circulating concentrations of progesterone are essential for the continuation of pregnancy. hCG secretion by the trophoblast of the developing pregnancy ‘rescues’ the corpus luteum, providing an LH‐like stimulus to maintain luteal progesterone secretion. Interruption of luteal progesterone synthesis and secretion, for example using the progesterone receptor antagonist mifepristone, is used in clinical practice to induce termination of early pregnancy. In contrast, luteal‐phase support in the form of hCG injection or injected or vaginal progestogens is used to maintain IVF pregnancies, since normal luteinization is interrupted by the GnRH agonist drugs used to prevent premature LH surges and unwanted ovulation. Menstruation refers to the shedding of the superficial layers of the endometrium with subsequent repair in preparation for regrowth from the basalis layer. Menstruation is initiated by the fall in circulating progesterone that follows luteal regression, i.e. failure of ‘rescue’ of the corpus luteum by an implanted early pregnancy (Fig 46.8). During luteolysis, progesterone secretion falls despite maintained serum concentrations of LH, since the corpus luteum becomes less sensitive to gonadotrophic support and becomes increasingly unable to maintain production of progesterone. In contrast, in a conception cycle, the increasing block to progesterone synthesis is overcome by the rapidly increasing concentrations of hCG which act on the corpus luteum through its LH receptors. Fig. 46.8 The histological appearances of the corpus luteum, showing (a) an active corpus luteum and (b) regression of the corpus luteum with histiocyte infiltration. In the immediate premenstrual phase, progesterone withdrawal initiates a complex series of intrauterine signals, including expression of chemotactic factors that draw leucocytes into the uterus and expression of matrix metalloproteinase enzymes, prostaglandins and other compounds that act on the uterine vessels and smooth muscle. The ‘invasion’ of leucocytes and subsequent expression of inflammatory mediators has led to menstruation being likened to an inflammatory event [8,9]. Prostaglandins of the E and F series are present in high concentrations in the endometrium, and their synthesis is regulated by the ovarian steroids. Increased production of PGF2α produces the myometrial contractions and vasoconstriction seen at menstruation, while E series prostaglandins increase pain and oedema, and are vasodilatory. PGE2 also appears to induce synthesis of the cytokine interleukin (IL)‐8, another key inflammatory and chemotactic mediator [10]. Pronounced vasoconstriction in turn leads to localized tissue hypoxia, further reinforcing release of inflammatory mediators. The end result of this cascade of events is constriction of the spiral arterioles with contraction of the uterine muscle, leading to expulsion of the shed tissue (Fig. 46.9). Fig. 46.9 Histological appearance of (a) late secretory and (b) menstrual endometrium. Source: Professor M. Wells, University of Sheffield. Reproduced with permission of Professor M. Wells. These studies have clear relevance to clinical management of menorrhagia and other menstrual disorders. Inhibitors of prostaglandin synthesis are widely used in these conditions, with good scientific basis. However, prostaglandin synthesis is also an important component of ovulation, and the use of powerful inhibitors of prostaglandin synthesis, such as non‐steroidal anti‐inflammatory agents, can lead to anovular cycles and involuntary infertility. Although complex, the endocrine and paracrine events that regulate the normal ovarian and uterine cycles are well understood. This chapter illustrates several examples by which understanding of the basic physiology of the cycle has led to scientifically based therapeutics. Further exploration of these regulatory mechanisms will produce new approaches to diagnosis and treatment for gynaecologists and their patients.
The Menstrual Cycle
Step one: ensuring mono‐ovulation
Folliculogenesis and the ‘follicular phase’
Transgenic/mutant mouse
Ovarian phenotype
C‐Kit deficiency, Kit ligand deficiency
Loss of germ cells (migration/proliferation failure)
WT1 knockout
Failure of gonadal development
BMP15/GDF9 knockout
Folliculogenesis arrest (primary stage)
IGF1 knockout
Folliculogenesis arrest (before antral follicle stage)
Kisspeptin/GPPSU knockout
Folliculogenesis alternation of LU stage
Oestrogen receptor gene knockout
Failure to ovulate
WNT4 knockout
Reduced germ cell number, masculinization
Step two: ensuring maintenance of very early pregnancy
The LH surge and ovulation
Endometrial development during the menstrual cycle and early pregnancy
Menstruation
Conclusion
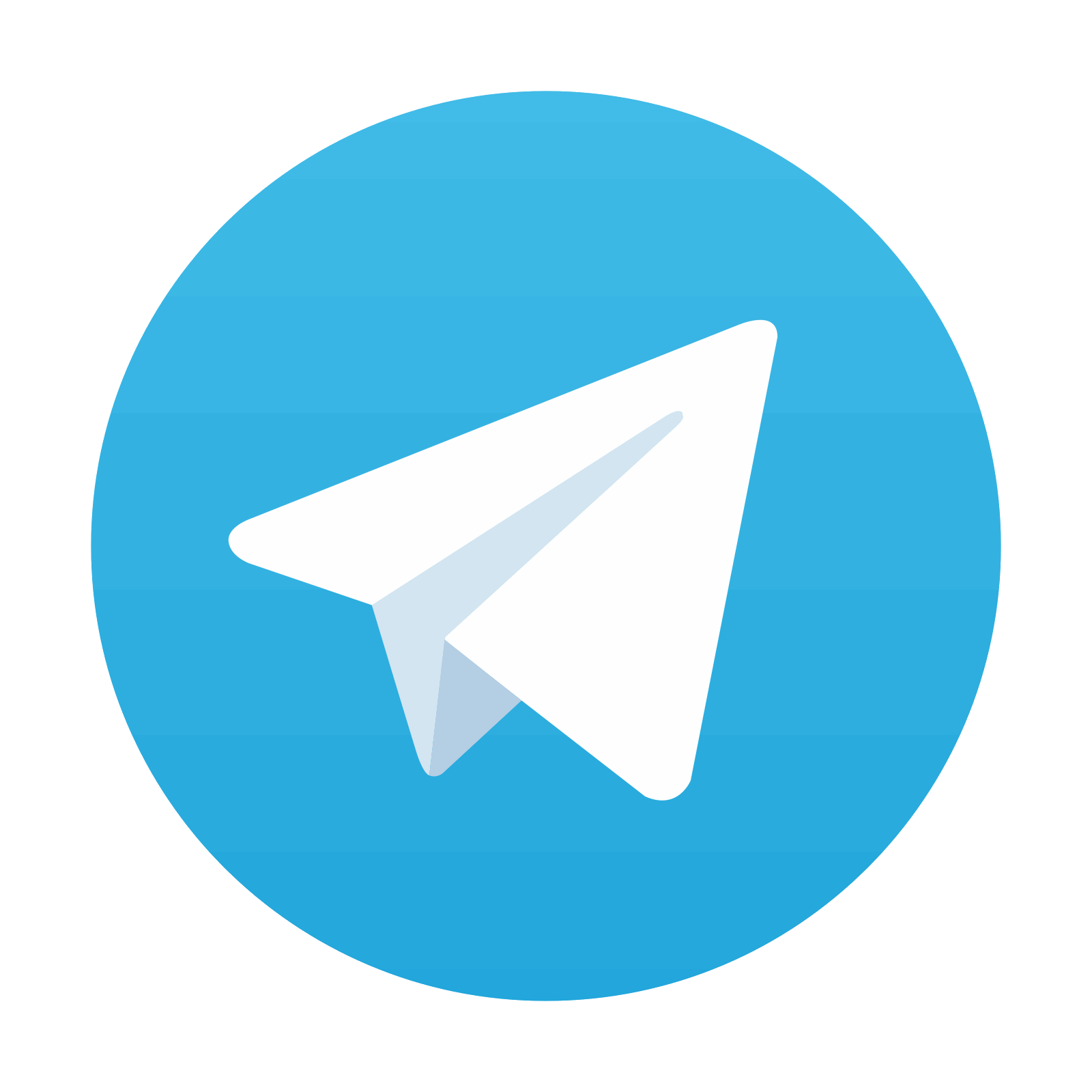
Stay updated, free articles. Join our Telegram channel

Full access? Get Clinical Tree
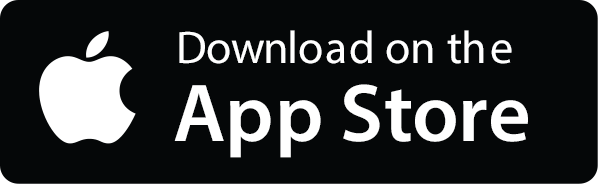
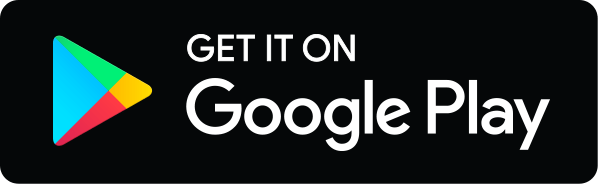