A constant supply of glucose to the brain is critical for normal cerebral metabolism. The dysglycemia of type 1 diabetes (T1D) can affect activity, survival, and function of neural cells. Clinical studies in T1D have shown impairments in brain morphology and function. The most neurotoxic milieu seems to be young age and/or diabetic ketoacidosis at onset, severe hypoglycemia under the age of 6 years followed by chronic hyperglycemia. Adverse cognitive outcomes seem to be associated with poorer mental health outcomes. It is imperative to improve outcomes by investigating the mechanisms of injury so that neuroprotective strategies independent of glycemia can be identified.
Key points
- •
Type 1 diabetes is associated with decrements in cognition during childhood and adolescence.
- •
The most neurotoxic milieu seems to be young age and/or diabetic ketoacidosis at onset, severe hypoglycemia under the age of 6, followed by chronic hyperglycemia.
- •
The observed cognitive changes are associated with observable changes in neuroimaging.
- •
Changes in cognitive performance are 0.3 to 0.8 standard deviations in full-scale IQ, affecting executive functioning, and functional and academic performance.
- •
There are anatomic and metabolic correlations between adverse cognitive and mental health outcomes.
Introduction
The primary metabolic fuel for the brain is glucose. In adults, brain energy consumption accounts for 25% of total body glucose utilization. In a developmental context, the pediatric brain dominates whole body metabolism. PET and MRI studies have shown that daily brain use of glucose peaks at 5.2 years at rates of 167 and 146 g/d in males and females, respectively. A constant supply of glucose to the brain is critical for normal cerebral metabolism. Thus, it is not surprising that developing brains in early childhood are more susceptible to metabolic insult, particularly those resulting from perturbations in blood glucose levels. Type 1 diabetes (T1D) is among the most common chronic diseases of childhood and the condition most likely to cause the greatest swings in amplitude in blood glucose on an hour-to-hour basis. Although changes in cognition among children with T1D have been documented for some time, it is only recently that sophisticated neuroimaging techniques have allowed direct measures of the impact of T1D on brain development.
Introduction
The primary metabolic fuel for the brain is glucose. In adults, brain energy consumption accounts for 25% of total body glucose utilization. In a developmental context, the pediatric brain dominates whole body metabolism. PET and MRI studies have shown that daily brain use of glucose peaks at 5.2 years at rates of 167 and 146 g/d in males and females, respectively. A constant supply of glucose to the brain is critical for normal cerebral metabolism. Thus, it is not surprising that developing brains in early childhood are more susceptible to metabolic insult, particularly those resulting from perturbations in blood glucose levels. Type 1 diabetes (T1D) is among the most common chronic diseases of childhood and the condition most likely to cause the greatest swings in amplitude in blood glucose on an hour-to-hour basis. Although changes in cognition among children with T1D have been documented for some time, it is only recently that sophisticated neuroimaging techniques have allowed direct measures of the impact of T1D on brain development.
The evidence for type 1 diabetes–related brain injury
Early Childhood
T1D is not uncommonly diagnosed in early life and in some surveys it is an increased prevalence in the under 5 year old age group that is most commonly seen. This is a particularly vulnerable age in terms of neurodevelopment. A study of preschool-aged children with an average of approximately 2 years’ diabetes duration showed them to have similar cognitive function when compared with healthy controls. However, when the group was subanalyzed by metabolic control, those children with higher hemoglobin A1C levels had lower general cognitive abilities, slower fine motor speed, and lower receptive language scores. A similar aged cohort was also found to have no differences overall in full-scale IQ compared with healthy controls; however, there were significant associations with poorer cognitive outcome and severe hypoglycemia or poorer metabolic control. MR studies in this preschool cohort showed lower than predicated white matter volume change with age and lower axial diffusivity values in the temporal and parietal lobes in the T1D group. Children who had a history of severe hypoglycemia had lesser gray and white matter volumes.
Mid Childhood
Group differences in overall cognitive performances between diabetic and nondiabetic cohorts are variably apparent by middle childhood with a history of either diabetic ketoacidosis (DKA) or severe hypoglycemia (especially in early life) being associated with poorer outcome. A cohort of T1D children with a mean age of 10 years performed significantly worse than healthy controls on 11 of 14 cognitive domains. Those children with a history of DKA had the worst outcomes on 8 of these domains. In a larger cohort of T1D children aged 6 to 18 years, children with a history of 3 or more episodes of severe hypoglycemia under the age of 5 years had significantly worse long-delay spatial delayed response performance compared with healthy controls and age- and duration-matched diabetes controls. Another group aged 4 to 10 years with median diabetes duration of 2.5 years did not show differences in IQ, although trends were apparent. MR studies in this age group are again already showing morphologic changes. In a large control study of T1D children with a mean age of 7 years, the T1D group showed gray matter volume change (decreased in the bilateral occipital and cerebellar regions and increased in the left prefrontal, insula, and temporal regions) with association between specific regional volumes and hyperglycemia. This same group also showed white matter change with reductions in white matter diffusivity observed throughout the brain, particularly in the frontal, temporal, parietal, and occipital lobes. White matter diffusivity was associated with hyperglycemia but not severe hypoglycemia. In these studies, the T1D cohort had significantly lesser full-scale IQ scores when compared with the matched healthy controls and this was associated with white matter structural change. Cross-sectional and longitudinal studies of older, peripubertal children with T1D compared with controls again showed changes in specific areas of brain volume, but only after a subanalysis was undertaken for those with a history of severe hypoglycemia and/or chronic hyperglycemia.
Adolescence and Early Adulthood
Late adolescence and early adulthood are informative life periods for cognitive study in T1D owing to duration of disease being usually longer and coincident with the time of neuromaturation. The 1 major study reported in this age group was a longitudinal control analysis of diabetic youth from the time of diagnosis to early adulthood. At baseline, both groups were matched for full-scale IQ and socioeconomic status. MR studies 12 years after diagnosis showed that relative to controls the T1D group had spectroscopy profiles consistent with lower neuronal density (reduced N -acetylaspartate) in the frontal lobes and basal ganglia and increase gliosis (increased myoinositol) and demyelination (increased choline) in the frontal, temporal, and parietal lobes. Regional gray and white matter volume changes were noted throughout multiple brain regions. Despite being matched for intelligence at baseline, after 12 years the T1D cohort had lost 0.3 standard deviations (SD) in full-scale IQ points and had twice the rate of school noncompletion. Similar spectroscopic changes suggestive of neuronal loss were reported in a case report of an adolescent with recurrent ketoacidosis who showed progressive decline of brain N -acetylaspartate.
Educational and Functional Outcomes
As discussed, measurable neurocognitive deficits have been described frequently in T1D. Metaanalyses of children and adults have shown mild (0.3–0.8 SD) decrements in full-scale IQ. Mild and specific deficits have been shown in the areas of attention, executive functions, psychomotor efficiency, and information processing speed. In children, memory and learning deficits were seen only in those with early onset disease and/or a history of severe hypoglycemia. In particular, cognitive outcomes have been found to be worse in those patients diagnosed in early life (<2 years of age). Whether these decrements should be seen as a consequence of disordered brain development or accelerated brain senescence is currently an area of debate. Functionally, however, there does seem to be a consequence of even mild decrements in cognition. Both Swedish and Finnish population registry–based studies have shown suboptimal academic secondary and primary school outcomes, respectively. A prospective Australian cohort study showed that older adolescents and young adults with T1DM had lower rates of school completion and work/study participation than healthy controls despite having similar IQ at diabetes onset 12 years previously. There is a highly plausible case that, within individuals, suboptimal metabolic control begets ongoing poor metabolic control through deficits in executive skills, such as goal setting, planning, organization, working memory and mental flexibility, reduced treatment adherence, and motivational issues secondary to impaired mental health.
Mental Health
Suboptimal mental health outcomes in children and adolescents with T1D have been well-documented with metaanalysis confirming that depression, anxiety, and eating disorders occur more commonly in both children and adults with T1D than in healthy controls. These affective disorders are often associated with poor metabolic control. Mental health disorders in T1D have been ascribed hitherto to the existential burden of living with the disease; however, there is an increasing body of evidence that their occurrence may also be linked to pathophysiologic brain changes that affect cognition as described. Corresponding brain regions are involved in cognitive and affective functions, including the hippocampus, prefrontal cortex, and limbic structure. Thus, neuronal injury in these areas is likely to have impacts on both cognition and mental well-being. A recent MR analysis of young adults with T1D and depression showed them to have elevations in prefrontal glutamate–glutamine–γ-aminobutyric acid, which were associated with degree of lifetime hyperglycemia. Finally, there is uncontrolled yet intriguing data showing short- and long-term improvements in behavior, mood, and cognition in children commencing insulin pump therapy.
Adulthood
Despite a high frequency of severe hypoglycemia, the Diabetes Control and Complications Trial failed to find any evidence of cognitive decline in young adults and adolescents followed prospectively over an 18-year period. Although this was initially reassuring, the baseline line assessment occurred relatively late in the course of neurodevelopment and was not reflective of premorbid cognitive ability. Another study similar to the adolescent cohort of the Diabetes Control and Complications Trial showed that, although there was no evidence of a significant loss in IQ in the overall cohort, episodes of severe hypoglycemia were again prognostic of cognitive outcome. This again was age dependent with severe hypoglycemia under 6 years of age having the most pronounced effect (a loss of 1.3 SD in IQ compared with controls) and episodes between 6 and 10 years of age (a loss of 0.7 SD). A cross-sectional MR study of a slightly older adult cohort showed no differences between T1D patients and controls in white matter; however, the T1D cohort showed reduced gray matter volumes in numerous regions, on this occasion showing association both with exposure to severe hypoglycemia and chronic hyperglycemia. In adults, an accelerated aging effect on brain function seems to be also mediated by long-term exposure to hyperglycemia, hypertension, and microvascular disease, including nephropathy, retinopathy, and neuropathy, which are all associated with cognitive deficits. There are increasing similarities noted between the brain injury of T1D in adults and Alzheimer’s disease, with several shared cerebrospinal fluid biomarkers.
Mechanisms of injury
Glucose is essential for neuronal metabolism and function. At the cellular level, glucose undergoes glycolysis (a sequence of 10 catalyzed reactions) to generate adenosine triphosphate, and the monocarboxylic acids pyruvate and lactate. Pyruvate and lactate in turn fuel the tricarboxylic acid cycle (generating more adenosine triphosphate). At the neuronal level, adenosine triphosphate is required for transmembrane ion pump activity, which in turn allows for maintenance of transmembrane voltage gradients, action potentials, and subsequent neurotransmission. Pyruvate and lactate are also chemical precursors to the neurotransmitters acetylcholine, glutamate and γ-aminobutyric acid. Pyruvate and lactate, along with ketone bodies (acetoacetate and β-hydroxybutyrate) and free fatty acids (FFAs), are themselves also secondary fuel sources for neurons.
Glucose is taken up by neurons via the glucose transporter proteins (GLUTs 1–12) and the H-myo-inositol transporter. The dominant GLUTs in the central nervous system are GLUT1 and GLUT3, maximally expressed in glial cells and neurons respectively. These 2 transporters are upregulated in response to hypoglycemia and hypoxia in an insulin-independent manner. Both transporters act in a bidirectional fashion that allows for equilibration rather than accumulation of glucose. Secondary fuel sources, pyruvate, lactate, and ketone bodies are transported across neuronal membranes via monocarboxylate transporters. Glucose is presented to the brain from the circulation across the blood–brain barrier, where endothelial junctions are opposed to perivascular astrocytic processes. Brain extracellular fluid levels of glucose are approximately 30% of circulating glucose levels with 20 to 30 minutes’ equilibration times during periods of variation in blood glucose. Not all glucose is metabolized immediately, with the main reserve existing in the form of cerebral glycogen. The bulk of this is held within astrocytes at levels several fold greater than cerebral glucose levels under physiologic conditions. In vitro studies have shown that stable glucose levels are essential for neuronal function and activity. Periods of low or high glucose levels, analogous to hypoglycemia and hyperglycemia, respectively, result in decreased neuronal activity and survival. Periods of rapid glucose fluctuation, analogous to the glycemic variation of diabetic dysglycemia, also result in neuronal injury, perhaps more profoundly so than in a unidimensional glycemic insult.
In periods of sustained insulinopenia or energy restriction, ketone bodies can serve as the primary cerebral energy source, providing up to 60% of metabolic requirements. Ketone bodies can also have direct influences on brain activity by causing increased levels of vascular permeability factor and the vasoconstrictor endothelin-1. In vivo animal studies have shown both benefits and injury to the brain from excessive ketosis, which are again related to context. Although exogenously administered ketones have been shown to reduce infarction and edema in response to hypoxic brain injury, ketones generated in a model of endogenous ketoacidosis resulted in reduced cerebral blood flow and production of high-energy phosphate metabolites.
Alternative cerebral energy resources include triglycerides, FFAs, and amino acids. FFAs are released from the metabolism of triglycerides. Amino acids are both important substrates for gluconeogenesis and neurotransmitters in their own right, and there are several active transport systems for them across the blood–brain barrier. Among the amino acids, glutamate is noteworthy. Glutamate is the most abundant free amino acid in the brain and acts as an excitatory neurotransmitter in the mammalian nervous system. Unlike other amino acids and alternative energy substrates, glutamate does not cross the blood–brain readily and it seems to be present mostly as a consequent rather than precursor metabolite of the tricarboxylic acid cycle. Glutamate, however, is also the precursor metabolite for the dominant inhibitory neurotransmitter γ-aminobutyric acid. Finally, both lactate and pyruvate, the intermediary metabolites between glucose and the tricarboxylic acid pathway, can also act as a reserve energy supply with brain extracellular fluid lactate levels being 3-fold greater than circulating lactate levels under physiologic conditions. FFAs, amino acids, lactate, and pyruvate are efficient secondary metabolic fuel reserves with exogenous administration of each causing cognitive recovery in an insulin clamp model of induced hypoglycemia.
Apart from energy metabolites, glucose-regulating hormones also have direct effects on the central nervous system. Both insulin and C-peptide cross the blood–brain barrier. Insulin receptors are widely distributed throughout the brain and, although insulin is not required for glucose uptake by neurons and astrocytes, insulin seems to play an important role in energy homeostasis and cognitive function within the central nervous system. Insulin also increases synapse density and dendritic plasticity in visual pathways as well as glucose utilization of neuronal networks. Decreased brain levels of insulin and C-peptide deficiencies may impact on neural survival through withdrawal of trophic factors (such as insulin-like growth factor-1 and nerve growth factor), leading to gray matter atrophy, and increased oxidative stress leading to neural apoptosis and white matter atrophy. Counterregulatory hormones are also relevant, affecting either directly or indirectly cognitive processes within the brain.
Notwithstanding the pleotropic array of potential mechanisms for neuronal injury in T1D, unstable blood glucose levels outside the physiologic range (dysglycemia) are an inevitable consequence of nonphysiologic insulin replacement and the primary insult. Dysglycemia can be characterized as extremes of glycemia—hypoglycemia and hyperglycemia—or rapid variations in glycemia—glycemic variation. Diabetic dysglycemia can be further subcategorized into mild to severe hypoglycemia (with altered conscious state and or seizure), prolonged hyperglycemia with or without ketoacidosis, and intraday or interday glycemic variation.
Hypoglycemia
Hypoglycemia is the form of dysglycemia that is associated most readily with neuronal insult. The cognitive effects of mild hypoglycemia induced by insulin clamp studies have been known for some time. Progressive cognitive dysfunction seems to occur below a blood glucose level of 3.0 to 3.5 mmol/L. The cognitive tests affected in these clamp studies included reaction time, trail making, and the Stroop test ( Fig. 1 ). These effects seem to be transitory, with rapid recovery occurring upon reestablishment of euglycemia.

Severe hypoglycemia can cause altered conscious state, progressing to seizures or coma and ultimately death. The Diabetes Control and Complications Trial showed that increased rates severe hypoglycemia were an inevitable consequence of lower hemoglobin A1C levels; however, with the advent of greater therapeutic insight, more predictable analog insulins and continuous subcutaneous insulin infusion therapy this paradigm seems to no longer be inevitable. Nonetheless, data from Germany and the United States indicate that severe hypoglycemia still occurs at frequency rates of 2% to 3% per year in pediatric populations under 6 years of age with T1D. An opportunistic study of 4 individuals who had hypoglycemic seizures while wearing continuous glucose monitoring devices has shown that profound hypoglycemia must be continually present for several hours before a seizure occurs ( Fig. 2 ). Rat studies have shown that just 1 episode of severe hypoglycemia followed by recovery in nondiabetic Wistar rats results in neuronal apoptosis and gliosis and a marked upregulation in neuronal GLUTs ( Fig. 3 ). It is unethical to induce hypoglycemia causing loss of consciousness and/or seizure in humans; thus, the associated acute brain changes have been poorly studied. Severe hypoglycemia seems to cause injury within the cortex, particularly the temporal and hippocampal regions, basal ganglia, and substantia nigra. In addition to cortical neuronal injury, there also seems to be subcortical white matter axonal injury involving major white matter tracts, such as the corpus callosum and internal capsule. Very limited studies have shown that these changes may be reversible in part with 1 adult case showing reversal of changes diffusivity. Another series of 3 prepubertal patients who underwent MR brain examination and cognitive assessment within 24 hours of hypoglycemic seizures initially showed altered spectroscopy. Reduced N -acetylaspartate in the frontal lobe was seen in all 3 patients and increased levels of trimethylamines in the temporal lobe were seen in 2 of the 3 patients. These metabolites are markers of neuronal density/activity and membrane turnover, respectively, and they improved over the 6-month follow-up period. Although there was some variation in cognitive function, all 3 patients showed significantly reduced selective attention, which also improved over the follow-up period.

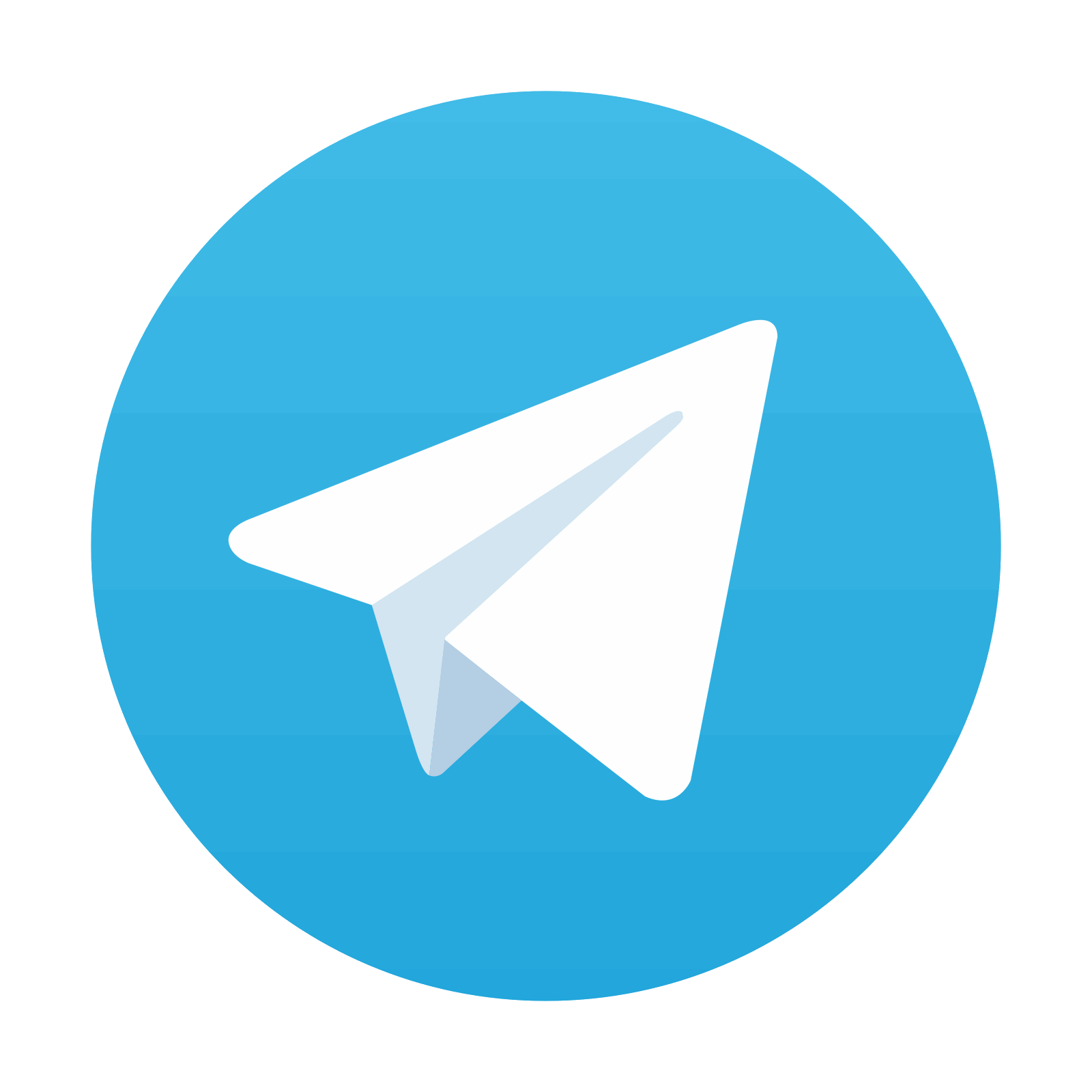
Stay updated, free articles. Join our Telegram channel

Full access? Get Clinical Tree
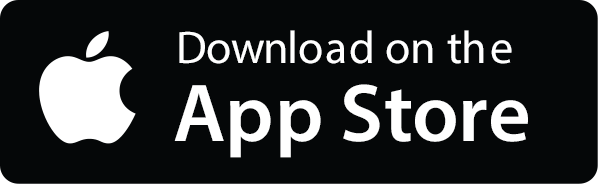
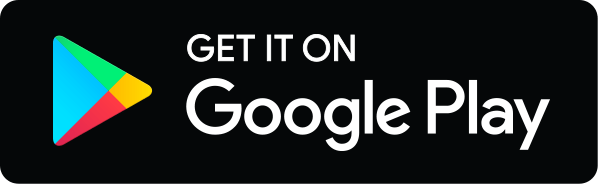