Introduction
The human microbiome consists of different microbial communities colonizing specific body sites ( Fig. 5.1 ) and fluctuating during several life stages. Because pediatrics is fundamentally oriented around the health and pathophysiology of the developing human, the rapid accumulation of new findings in microbiome science during the past decade provides a new frame of reference for human growth and development. Human microbes are part of the conversation regarding human physiology during fetal development even before birth, and these microbial communities continue to shift and develop during infancy and childhood. Beyond microbial composition, functional features of microbial metabolism are leading to the identification and rediscovery of potentially important bioactive compounds originating from the microbiome. Dietary changes, so prominent in early childhood, clearly impact the biology of the microbiome early in life and may have important consequences for disease risk.

Antibiotics may suppress and eliminate many commensal microbes, placing the host at risk for disorders of microbial ecology such as Clostridium difficile infection. Diet and antibiotics represent two major environmental factors affecting the biology of the microbiome, with potentially deleterious effects on microbial diversity and resilience of microbial communities. Commensal microbes produce a variety of antimicrobial compounds that modify microbial composition and may help the host defend against pathogens. Beneficial microbial species may induce the production of antimicrobial peptides, such as defensins, by the host. During infection, bacterial and other pathogens may have a profound impact on the composition and biology of the microbiome. Humans inhabiting this microbial world must coexist and thrive with indigenous microbial communities during growth and development. This intimate partnership between microbes and infant/child may affect susceptibility to pediatric infections and modulate the course and severity of infectious diseases.
Development in Early Life Through Childhood
The fetus and neonate are exposed to microbial communities during prenatal and early postnatal development. Descriptions of a placental microbiome highlight the presence of functionally dynamic microbial communities at the maternal-fetal interface. Even if microorganisms do not directly contact the fetus, microbial metabolites may have an impact on fetal development. The presence of microbes in the colostrum and amniotic fluid provides evidence that placental microbes may contact the fetus via the circulation. Mouse models indicate that bacterial species such as Fusobacterium spp. may “home” specifically to the placenta via the bloodstream. This finding emphasizes a key point that the placental microbiome is most similar to the oral microbiome, lending support to the concept that the oral microbiome may provide founder bacterial species for the placental microbiome in the early stages of pregnancy. The presence of oral pathogens may place the fetus at risk if oral pathogens can traverse the bloodstream and seed the placental microbiome. As we consider the nature of infections in utero in maternal-fetal medicine, this connection between the oral and placental microbiomes may point to new strategies to evaluate the oral microbiome before or during pregnancy and provide additional information regarding potential risks for the fetus. Additional concepts based on experimental data include differences in the placental microbiome in cases of preterm birth and whether differences in microbial composition or function may place the fetus at increased risk of preterm birth. Microbial metabolites such as microbe-derived calcium channel blockers may affect contractility of the uterine musculature and the tendency toward premature contractions prior to childbirth. Prior antimicrobial therapy has a fundamental impact on the composition of the placental microbiome, and antecedent antimicrobial exposure yields significant differences in the placental microbiome that could affect fetal development and preterm birth. All of these conceptual points highlight the rapidly emerging understanding of the impact of the microbiome on the health and disease susceptibility of the fetus.
Several studies have indicated that the neonatal and infant gut microbiome develops rapidly after birth and may influence susceptibility to enteric infections and acute disease. The neonatal microbiome matures rapidly in terms of microbial acquisition, and relatively complex microbial communities form in the intestine and other body sites by the end of postnatal week 1. Microbiome development continues at a rapid pace and with relatively large-scale fluctuations in composition and function during infancy ( Fig. 5.2 ). Immediately after birth, the neonate is colonized by various microbes belonging to different phyla at different body sites. The major phyla that are established in the human body by the end of infancy include Bacteroidetes, Firmicutes, Proteobacteria, and Actinobacteria. One of the hallmarks of the early neonatal microbiome is that microbial composition does not differ significantly by body site. It takes weeks or months during infancy to see evidence of “differentiation” of distinct microbial communities at each body site. Within days, different body sites are rapidly colonized, but these communities gradually change to reach a steady state of microbial communities that are highly adapted for a specific body site. This process of “microbiome differentiation” may have important consequences for infectious disease risk depending on the nature and timing of the infection.

At approximately 4 to 6 weeks age, the microbiomes at different body sites are clearly distinguishable, and the impact of mode of delivery on microbial composition is negligible. By 2 to 3 years of age, it appears that the intestinal microbiome is comparable to the adult microbiome in terms of complexity. The impact of diet on microbial composition during the first 3 years of life is dramatic, and breast milk to solid food transitions clearly change the composition and function of the intestinal microbiome. During childhood and prior to adolescence, the pediatric intestinal microbiome continues to differentiate in terms of microbial composition and function. The relative richness of the microbiome in childhood actually exceeds that of healthy young adults, so presumably bacterial species are culled during adolescence. Although the major phyla are established during infancy, the relative preponderance of different phyla differs between school-aged children and young adults. For example, children have a greater relative abundance of Firmicutes relative to Bacteroidetes, and genera such as Bifidobacterium and Faecalibacterium are enriched in children. Children also contain specific functional elements, such as pathways for vitamin B 12 and folate biosynthesis, which are enriched in the pediatric gut microbiome. These pathways may promote hematologic and central nervous system development. Firmicutes including clostridia may produce short-chain fatty acids (SCFAs) that can suppress enteric pathogenesis, and specific commensal species may produce various antimicrobial peptides and propionaldehyde compounds that suppress enteric pathogens.
Impact of Environmental Factors: Diet and Medications
Various environmental factors such as dietary food intake and consumption of medications may influence and modulate the composition and function of the human microbiome in different body sites. Breast milk drives predictable changes in microbial composition, and infants receiving breast milk clearly differ by intestinal microbial composition when compared to formula-fed infants. Human breast milk contains indigenous microbes including Bifidobacterium, Lactobacillus, and Streptococcus species, and these species colonize the gut microbiome of infants shortly after initiation of breastfeeding. The gastrointestinal microbiome rapidly expands in terms of richness and diversity during the first weeks of life if the infant is consuming breast milk and avoiding antimicrobial agents. Breastfeeding results in changes to the microbiome and host that result in reduced susceptibility to necrotizing enterocolitis (NEC). The addition of solid foods to the diet results in the next wave of profound changes to the gastrointestinal microbiome during the first 3 years of life, and these changes may affect relative susceptibility to enteric infections during childhood. Comparisons of children from western Africa (Burkina Faso) consuming a diet rich in cereals, vegetables, and plant fiber with children from Western Europe (Italy) on a diet enriched in animal protein, sugar, and fat yielded dramatic differences in gut bacterial composition at the phylum level. Children from Italy yielded gut microbiota dominated by the Firmicutes and Proteobacteria phyla, whereas children from Burkina Faso yielded microbiota more abundant in Actinobacteria and Bacteroidetes. A diet rich in plant polysaccharides and low in sugars and fats is predicted to increase microbial diversity and therefore confer protection from enteric pathogens. Although host genetics, sex, and geography are known to influence the composition of the gut microbiota, accumulating evidence suggests that diet has a relatively large impact on biodiversity within the gut ecosystem.
Dietary manipulation by the addition of beneficial microbes (probiotics) or creation of highly specialized diets may prevent infectious diseases or facilitate management of chronic intestinal disease phenotypes. Consumption of probiotics may dramatically alter the microbial composition and function of the pediatric intestinal microbiome, principally by changes in microbial gene expression and microbial metabolism. Ingestion of probiotics during infancy reduced the risk of enteric infections, and probiotics may protect children from acute gastroenteritis. On the other side of the spectrum, a restricted elemental diet used for treating pediatric patients with Crohn’s disease further reduced the biodiversity of the gastrointestinal microbiome while ameliorating symptoms. In addition to dietary manipulation, medications may have a profound impact on microbial composition and function in the intestine. Antimicrobial agents administered in the first few days of life to neonates may delay colonization of the intestine and could increase the risk of enteric infections by reducing colonization resistance in the host ( Fig. 5.3 ). Different classes of antimicrobial agents may have different effects on the intestinal microbiome, and we are just beginning to appreciate the different risks associated with exposure to different antimicrobial agents. A classic example is recurrent C. difficile infection and antimicrobial-associated diarrhea due to treatment with antimicrobial agents such as cephalosporins and fluoroquinolones.

Body Metabolism and Immunity
SCFAs are well-known examples of microbial metabolites affecting the intestinal epithelium. Three main SCFAs produced in the human colon are acetate, propionate, and butyrate. Propionate and acetate affect adipogenesis and leptin production. By modulating gut- and adipocyte-derived hormones, SCFAs may affect the gut-brain axis and brain function, including appetite and satiety. Acetate is able to directly affect neuronal function in the brain, especially regions associated with appetite. With respect to enteric infections, Shiga toxin–producing strains of Escherichia coli can produce serious, life-threatening infections in children, resulting in hemolytic uremic syndrome and renal injury. Studies in mouse models indicate that Bifidobacterium species can suppress translocation of Shiga toxin across the intestinal epithelium and that the organic acid acetate contributes to this effect. B-complex vitamins including vitamin B 12 are also produced by bacterial members of the intestinal microbiome, and vitamin B 12 may suppress Shiga toxin by E. coli. Bioactive compounds produced by the commensal intestinal microbiota may regulate toxin production by enteric pathogens and reduce susceptibility to enteric infections.
Beneficial microbes may convert tryptophan into bioactive indole compounds. Indole derivatives act as endogenous ligands for the arylhydrocarbon receptor (AhR) and are generated from dietary tryptophan by commensal intestinal microbes. By binding AhR, the microbiota may stimulate mammalian immune cells to produce the cytokine interleukin (IL)-22, thereby enhancing protective mucus production, suppressing IL-17 production, and stimulating production of antimicrobial peptides via signal transducer and activator of transcription 3 (STAT3) activation. The lesson in Lactobacillus -mediated indole-3-aldehyde (one example of an indole derivative) production from tryptophan is that bacterial metabolites generated by the microbiome may have a profound impact on immunity. The metabiotic concept refers to metabolites produced by the microbiome or probiotic species that may confer specific benefits on mammalian physiology or immunity. The mammalian tryptophan catabolic enzyme indoleamine 2,3-dioxygenase 1 (IDO1) plays a key role in the conversion of dietary or microbial tryptophan into kynurenines and promotes tolerance to commensal microbes. The capacity of IDO1-expressing dendritic cells, epithelial cells, and kynurenines to induce regulatory T cells (Tregs) and inhibit T-helper 17 cells (T H 17) has revealed their unexpected potential to control inflammation, allergy, and T H 17-driven inflammation in fungal infections.
Amino acid decarboxylation systems, such as the glutamate decarboxylase (GAD) or histidine decarboxylase (HDC) systems, enable bacterial species to cope with acid stress in the gastrointestinal tract. These systems are providing insights into infectious diseases and intestinal inflammation as it pertains to childhood. The bacterial GAD system is enriched in the intestinal microbiome relative to other body sites of healthy adults, and γ-aminobutyric acid (GABA) is enriched in the intestines of patients with recurrent C. difficile –associated disease (CDAD). Most intestinal GABA measured in stool is microbial in origin, and this metabolite appears to potentiate the risk of C. difficile infection. Individuals consuming the GABA A receptor agonist zolpidem (Ambien) have increased risk of C. difficile infection, adding support to the potentially pathogenic role of GABA in recurrent CDAD in children. Microbial species in the human gut can also convert l -histidine to histamine. This biogenic amine may promote allergic inflammation, suppress intestinal inflammation, or function as a neurotransmitter in the central nervous system by binding to any of the four different histamine receptors found on human cells. Antihistamines function by blocking signaling through the histamine 1 or histamine 4 receptors (H 1 R and H 4 R), and these receptors are considered proinflammatory. In contrast, histamine may promote gastric acid production and suppress intestinal inflammation via H 2 R. Although H 2 R blockers have been useful as adjunct medications in the treatment of Helicobacter pylori infection, they may also promote mucosal or systemic inflammation. Studies in the pediatric literature have documented increased risk of and mortality from NEC in newborns exposed to H 2 R blockers. Gut microbes may generate histamine in the small or large intestine and serve as a checkpoint for immunoregulation in the gastrointestinal tract. These amino acid metabolites may have opposing effects in terms of mucosal immunity, and microbe-derived compounds may help modulate susceptibility to pediatric infections.
Microbiome at Different Body Sites
Airway and Respiratory Tract Microbiome
The fetal airway is likely exposed to microbial influences in utero due to the direct contact between the respiratory tract and the surrounding environment. However, bacterial niches in the airway are vastly different after birth as the respiratory tract transitions from a fluid-filled cavity to a functional organ involved in gas exchange. Upon exposure to the external environment, the human respiratory tract is rapidly colonized by a community of commensal bacteria that gradually matures and diversifies during the first year of life. Skin-associated bacterial genera including Staphylococcus and Corynebacterium species are detectable in the upper respiratory tract on the first day of life and are dominant up to 3 months of age. The prevalence of these species steadily declines from birth to 6 months, and these microorganisms are succeeded by other bacterial species such as Streptococcus pneumoniae , Moraxella catarrhalis , and Haemophilus influenzae. Geography, season of birth, environment, social interactions, and presence of older siblings are primary determinants of colonization rate and sources of bacterial acquisition. The infant lung has distinct compartments with different growth conditions allowing enhanced growth of Bacteroidetes, unlike the upper respiratory tract, which is dominated by Firmicutes and Proteobacteria. Microbiota traverse the upper and lower respiratory tracts via mucosal extension, microaspiration, and ciliary transport. These factors along with local growth conditions in human airways, immunity of the respiratory tract, and microbial crosstalk contribute to the community structure at specific locations within airways. Interactions between the intestinal and respiratory microbiota have been suggested, and early nutritional exposures may also influence the developmental trajectory of the respiratory microbiome.
Dynamic changes in the composition of the respiratory tract microbiome during the first year of life may affect the development of the respiratory mucosal immune system. The pattern of immune responses in the respiratory tract modulates relative susceptibilities to allergic inflammation or respiratory tract infections. A diverse, homeostatic microbiome is necessary for immune priming and defense against pathogen overgrowth and invasion. Microbial exposure in the lung during the first 2 weeks of life induces a programmed cell death ligand 1 (PDL1)-mediated tolerance to aeroallergens. Relatively limited biodiversity and bacterial load in the airways are associated with improper development of regulatory immune cells, leaving the host susceptible to allergic inflammation lasting into adulthood. The composition of the microbial community in the upper respiratory tract also influences the susceptibility of the lower airways to viral infections and affects the severity of the resulting inflammatory response. Antibiotic use and inflammation resulting from viral infections can also lead to dysbiosis and the development of early allergic sensitization.
Early colonization with a diverse respiratory microbiota is associated with a decreased risk of developing asthma, and overall microbial community composition appears to be a main determinant for asthma. Relative overgrowth of specific bacterial species may exacerbate the risk of developing asthma in genetically predisposed newborns. Beyond allergic inflammation, physicochemical alterations in chronic lung disease may alter the airway microbiota and susceptibility to respiratory tract infections. Disruption of the microbial ecosystem can be a consequence of host factors such as cystic fibrosis in which increased mucus production and impaired mucociliary clearance result in altered local growth conditions and reduced microbial clearance favoring the growth of specific respiratory pathogens.
Gastrointestinal Microbiome
The intestinal microbiome develops rapidly after birth in both premature and full-term infants. In premature infants the succession of bacterial classes demonstrates a temporal sequence shifting from a relative abundance of Bacilli (gram-positive aerobes) to Gammaproteobacteria (gram-negative facultative anaerobes). Both Clostridia and Gammaproteobacteria increase in relative abundance with increasing postconceptional age. Since the class Gammaproteobacteria contains several potential enteric pathogens, perhaps these microbes promote complete maturation of the intestinal immune system. Alternatively the gut milieu accommodates a greater variety of microbes with time during infancy, including potential pathogens. Potential pathogens lurking in the intestinal microbiome early in life may invade the bloodstream of premature infants. Pathogenic bacteria such as E. coli , group B streptococcus (GBS), and Serratia marcescens were documented in the intestinal microbiomes of premature infants prior to late-onset sepsis by the same microbes (verified by molecular epidemiology/sequencing). Additionally, ciprofloxacin-resistant E. coli were isolated from children and their mothers, and maternal colonization by drug-resistant bacteria was correlated with childhood carriage of the same organisms. Therefore, the presence of potential pathogens and drug-resistant microbes in the intestine may have important implications for familial transmission and infection risk.
A classic example of infectious disease risk associated with assaults on the intact microbiome is the category of antimicrobial-associated diarrhea (AAD) or colitis. Human patients and animal models with intestinal microbiomes of limited diversity following antimicrobial agent challenge clearly seem to be at increased risk for CDAD or related disorders. Antimicrobial agents of various classes (cephalosporins, fluoroquinolones) are associated with development of AAD in children and adults and highlight the importance of an intact gut microbiome in resistance to infection by intestinal pathogens. In addition to therapy by specific antimicrobial agents (e.g., metronidazole, vancomycin), microbiome supplementation or replacement by fecal microbiota transplantation (FMT) emphasizes the importance of the intestinal microbial community in resolution of CDAD. A potential confounder is co-infection with C. difficile and other pathogens in cases of AAD. Enteric infections result in widespread perturbations of the intestinal microbiome highlighted by the phylum Proteobacteria, a phylum that contains many enteric pathogens including Escherichia. Resolution of infection is accompanied by distinct changes in the microbiome, with a greater abundance of the genus Bacteroides , as expected. Possibly rapid sequencing of the intestinal microbiome may provide valuable information regarding the condition of the microbiome in the context of undiagnosed enteric infections.
Skin and Vaginal Microbiomes
The structure of the vaginal microbiome varies in pregnancy by gestational age and is characterized by decreased bacterial diversity and species richness. However, the genus Lactobacillus persistently maintains prevalence, and certain species including L. iners, L. crispatus, L. jensenii, and L. johnsonii have been demonstrated to be enriched during pregnancy. Consequently, the dominant skin microbiota observed on vaginally delivered babies includes Lactobacillus spp., as well as Prevotella , Atopobium , and Sneathia species. The transmission of the vaginal microbiota to the baby may provide protection against pathogens by occupying niches prior to the development of site-specific communities. In babies delivered via C-section, the lack of exposure to vaginal microbes results in an initial microbial community similar to the mother’s skin microbiota, with an abundance of Staphylococcus species. Premature infants are also at risk of disturbed initial bacterial colonization, and recent studies suggest that room and isolator surfaces in neonatal intensive care units are a reservoir of colonizing microbes for these infants. This care environment may be detrimental to the health of these preterm newborns because they have an underdeveloped skin barrier that makes them more vulnerable to chemical damage and microbial infections. However, the infant skin microbiome is dynamic, and the initial differences arising from mode of delivery do not persist as site-specific niches develop in the initial months after birth.
The skin is a continuously self-renewing organ that undergoes rapid turnover and expansion during the first years of life. As a result, the infant skin microbiome continuously matures during this time frame, increasing in diversity and abundance. The skin microenvironment varies greatly between infants and adults due to this rapid skin expansion and the distinct composition of infant skin microstructure, which varies in epidermal thickness and cell size. Around 3 months of age, site-specific communities begin to emerge. The skin microbiota consists of both commensal microbes that reestablish after perturbation and transient microbes from the surrounding environment. Distinct niches form on the body based on levels of moisture and sebum, and glands and hair follicles have their own distinct microbiota. The diversity and composition of the bacterial community at these body sites is also affected by intrinsic factors such as age and genetics, as well as by extrinsic factors such as climate and hygiene, leading to large intrapersonal variations in community composition. The most abundant bacterial phylum colonizing the infant skin is Firmicutes whereas, in contrast, adults are dominated by Actinobacteria. Staphylococci (Firmicutes) are abundant in infants under 1 year of age, and certain skin commensals such as S. epidermidis may play a protective role against pathogens. S. epidermidis has been demonstrated to inhibit colonization and biofilm formation of the opportunistic pathogen Staphylococcus aureus by competition and expression of a serine protease. S. epidermidis also secretes small molecules that can activate Toll-like receptor TLR2 signaling and induce antimicrobial peptide expression by keratinocytes, thus enhancing the skin’s defense against infection. Staphylococcus species may promote early immune development, but their effect is predicted to decline because their abundance decreases steadily during the first year of life. However, skin barrier function and immune development are significantly affected by early microbial colonization, and microbial exposure may also affect the maturation of the systemic immune system.
Shifts in microbial communities can drive some commensals to become pathogenic under certain circumstances. Although it is considered a commensal organism, S. epidermidis is a primary cause of nosocomial infections and can cause disease if barrier integrity is compromised and it is allowed to invade other sites. Several skin disorders have also been associated with certain members of the microbiota. Atopic dermatitis (AD), or eczema, is a chronic disorder that affects approximately 15% of children in the United States. AD is characterized by S. aureus colonization on both lesional and nonlesional skin, and it is associated with worsened disease severity in pediatric patients. Propionibacterium acnes is well known for its role in acne vulgaris in teenagers because this species secretes enzymes that injure the tissue lining of the already inflamed pilosebaceous unit. Although infants have limited sebum production, infantile acne begins to appear at the same time that an increase in Propionibacterium occurs at age 4 to 6 months. Psoriasis is another chronic skin condition that can occur in children, and lesional skin has been demonstrated to exhibit increased bacterial diversity concurrent with a decrease in prevalence of healthy skin bacteria such as Actinobacteria. These examples highlight how the skin barrier and the dynamic microbial communities that inhabit it are maintained in a delicate balance, the disturbance of which can predispose the host to cutaneous infections and other inflammatory conditions.
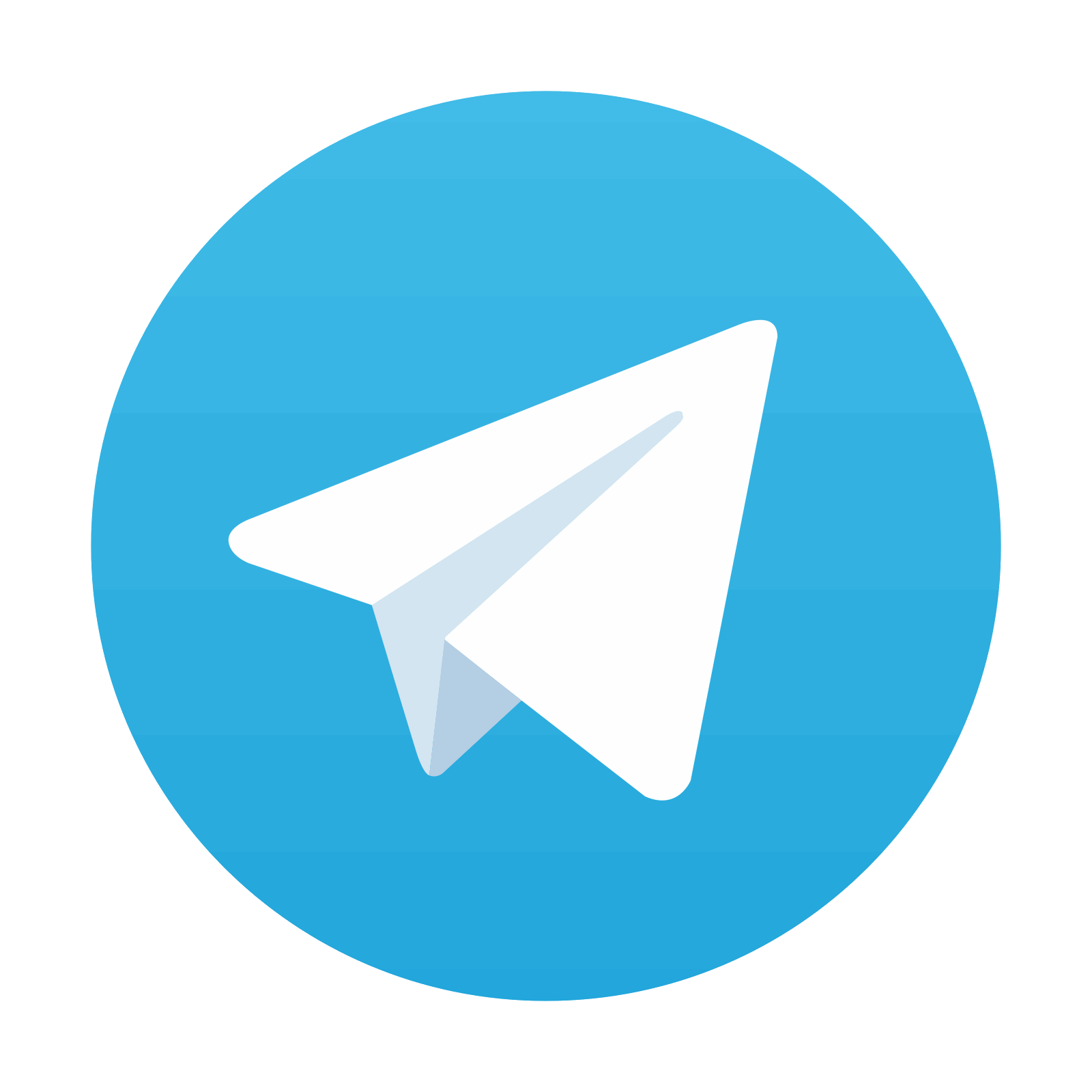
Stay updated, free articles. Join our Telegram channel

Full access? Get Clinical Tree
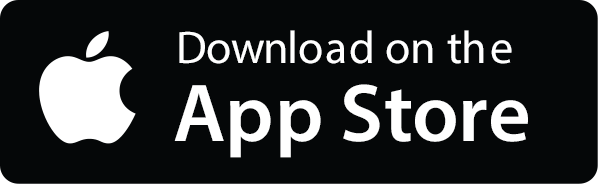
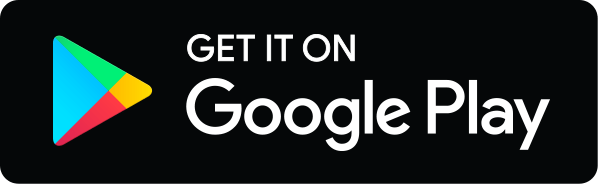