Background
Maternal diabetes increases the risk of neural tube defects in offspring. Our previous study demonstrated that the green tea polyphenol, Epigallocatechin gallate, inhibits high glucose-induced neural tube defects in cultured embryos. However, the therapeutic effect of Epigallocatechin gallate on maternal diabetes–induced neural tube defects is still unclear.
Objective
We aimed to examine whether Epigallocatechin gallate treatment can reduce maternal diabetes–induced DNA methylation and neural tube defects.
Study Design
Nondiabetic and diabetic pregnant mice at embryonic day 5.5 were given drinking water with or without 1 or 10 μM Epigallocatechin gallate. At embryonic day 8.75, embryos were dissected from the visceral yolk sac for the measurement of the levels and activity of DNA methyltransferases, the levels of global DNA methylation, and methylation in the CpG islands of neural tube closure essential gene promoters. embryonic day 10.5 embryos were examined for neural tube defect incidence.
Results
Epigallocatechin gallate treatment did not affect embryonic development because embryos from nondiabetic dams treated with Epigallocatechin gallate did not exhibit any neural tube defects. Treatment with 1 μM Epigallocatechin gallate did not reduce maternal diabetes–induced neural tube defects significantly. Embryos from diabetic dams treated with 10 μM Epigallocatechin gallate had a significantly lower neural tube defect incidence compared with that of embryos without Epigallocatechin gallate treatment. Epigallocatechin gallate reduced neural tube defect rates from 29.5% to 2%, an incidence that is comparable with that of embryos from nondiabetic dams. Ten micromoles of Epigallocatechin gallate treatment blocked maternal diabetes–increased DNA methyltransferases 3a and 3b expression and their activities, leading to the suppression of global DNA hypermethylation. Additionally, 10 μM Epigallocatechin gallate abrogated maternal diabetes–increased DNA methylation in the CpG islands of neural tube closure essential genes, including Grhl3 , Pax3 , and Tulp3 .
Conclusion
Epigallocatechin gallate reduces maternal diabetes–induced neural tube defects formation and blocks the enhanced expression and activity of DNA methyltransferases, leading to the suppression of DNA hypermethylation and the restoration of neural tube closure essential gene expression. These observations suggest that Epigallocatechin gallate supplements could mitigate the teratogenic effects of hyperglycemia on the developing embryo and prevent diabetes–induced neural tube defects.
Currently nearly 60 million women of reproductive age (18–44 years old) worldwide have diabetes, and this number has been estimated to double by 2030. Clinical studies and animal model investigations have revealed that maternal diabetes increases the risk of neural tube defects in offspring and that hyperglycemia is a teratogen.
Although strict glycemic control by lifestyle and pharmacological treatment can decrease the incidence of hyperglycemia-induced embryonic malformations in pregnancies affected by preexisting maternal diabetes, euglycemia is difficult to achieve and maintain, and even transient exposure to high glucose could lead to abnormal embryonic development. Thus, diabetes-induced birth defects are significant public health problems, and there is an urgent need for new therapeutic approaches against diabetic embryopathy.
Neural tube defects are common complex congenital malformations of the central nervous system that form during embryogenesis. There are approximately 5 times more neural tube defects in offspring from diabetic mothers than in those from nondiabetic mothers, despite modern preconception care. Studies from our group and others have demonstrated that maternal diabetes induces cellular stress, including oxidative stress and endoplasmic reticulum stress, and that those cellular stresses cause apoptosis in the embryonic neural tissue, leading to neural tube defect formation. Recently several studies have suggested that altered DNA methylation disrupts the folate metabolic pathway and causes neural tube defects. Therefore, we hypothesize that altered DNA methylation is involved in neural tube defect formation in diabetic pregnancies.
Our previous studies have revealed that naturally occurring polyphenols exert protective effects against high glucose–induced neural tube defects in vitro. Epigallocatechin gallate is the major polyphenol in green tea ( Camellia sinensis ) and makes up to approximately 30% of the solids in green tea.
Epigallocatechin gallate is the subject of increasing research interest because it has demonstrated beneficial effects in studies of diabetes, Parkinson’s disease, Alzheimer’s disease, stroke, and obesity. The cancer-preventive effects of Epigallocatechin gallate have been widely reported in epidemiological, cell culture, animal, and clinical studies. One of the mechanisms by which Epigallocatechin gallate exerts effects on cancer cells is through the inhibition of DNA methyltransferases and reactivation of DNA methylation–silenced gene expression. Thus, in the present study, we investigated whether Epigallocatechin gallate could reduce or prevent neural tube defect formation in embryos from diabetic dams and inhibit maternal diabetes–increased DNA methylation.
Methods and Materials
Animals and reagents
All animal procedures were approved by the University of Maryland School of Medicine Institutional Animal Care and Use Committee. Wild-type C57BL/6J mice were purchased from The Jackson Laboratory (Bar harbor, ME). Streptozotocin from Sigma (St Louis, MO) was dissolved in 0.1 M citrate buffer (pH 4.5). Sustained-release insulin pellets were purchased from Linplant (Linshin, Canada).
The mouse model of diabetic embryopathy
Our mouse model of diabetic embryopathy was described previously. Briefly, female mice were intravenously injected daily with 75 mg/kg streptozotocin over 2 days to induce diabetes. Nondiabetic wild-type mice with vehicle injection served as controls. Diabetes was defined as 12 hour fasting blood glucose levels of ≥250 mg/dL, which normally occurred at 3–5 days after streptozotocin injections. Once the level of hyperglycemia indicative of diabetes (≥250 mg/dL) was achieved, insulin pellets were subcutaneously implanted in these diabetic mice to restore euglycemia prior to mating. The mice were then mated with wild-type male mice at 3:00 pm .
We designated that the morning when a vaginal plug was present as embryonic day 0.5. On embryonic day 5.5, insulin pellets were removed to permit frank hyperglycemia (>250 mg/dL glucose level), so the developing conceptuses would be exposed to hyperglycemic conditions. Wild-type, nondiabetic female mice with vehicle injections and sham operation of insulin pellet implants served as nondiabetic controls. On embryonic day 8.75, the mice were euthanized, and conceptuses were dissected out of the uteri for analysis. Embryos were harvested at embryonic day 8.75 for analysis and at embryonic day 10.5 for neural tube defect examination.
At embryonic day 10.5, embryos were examined under a Leica MZ16F stereomicroscope (Leica, Wetzlar, Germany) to identify neural tube defects. Images of embryos were captured by a DFC420 5-megapixel digital camera with software (Leica). Normal embryos were classified as having completely closed neural tube and no evidence of other malformations. Malformed embryos were classified as showing evidence of failed closure of the anterior neural tubes, resulting in exencephaly, a major type of neural tube defect.
Epigallocatechin gallate treatment
Epigallocatechin gallate treatment was performed as described previously. Concentrations of either 1 or 10 μM Epigallocatechin gallate (Sigma) were given to wild-type nondiabetic and diabetic pregnant mice at embryonic day 5.5 in drinking water.
Real-time polymerase chain reaction
Using the Trizol (Invitrogen, Carlsbad, CA), messenger RNA was isolated from embryonic day 8.5 embryos and then reversed transcribed using the high-capacity complementary DNA archive kit (Applied Biosystems, Grand Island, NY). Real-time polymerase chain reaction for Dnmt1 , Dnmt3a , Dnmt3b , Grhl3 (grainyhead-like-3), Pax3 (paired box gene 3), Tulp3 (tubby-like-3), and β-actin was performed using the Maxima SYBR Green/ROX quantitative polymerase chain reaction master mix assay (Thermo Scientific, Rockford, IL) in the StepOnePlus system (Applied Biosystems, Grand Island, NY). Primer sequences are listed in Table 1 .
Primer name | Primer sequences |
---|---|
Dnmt1 | Forward primer, 5′- AAGAATGGTGTTGTCTACCGAC -3′ |
Reverse primer, 5′- CATCCAGGTTGCTCCCCTTG -3′ | |
Dnmt3a | Forward primer, 5′- GATGAGCCTGAGTATGAGGATGG -3′ |
Reverse primer, 5′- CAAGACACAATTCGGCCTGG -3′ | |
Dnmt3b | Forward primer, 5′- CGTTAATGGGAACTTCAGTGACC -3′ |
Reverse primer, 5′- GGGAGCATCCTTCGTGTCTG -3′ | |
Grhl3 | Forward primer, 5′- CCCGGCAAGACCAATACCG -3′ |
Reverse primer, 5′- AACCCCATGAATGCTCTCAAAT -3′ | |
Pax3 | Forward primer, 5′- TTTCACCTCAGGTAATGGGACT -3′ |
Reverse primer, 5′- GAACGTCCAAGGCTTACTTTGT -3′ | |
Tulp3 | Forward primer, 5′- CCAAAAACACGGCATCTTGAG -3′ |
Reverse primer, 5′- GGGCTATACGCAAAGTCCTCTAA -3′ | |
β-Actin | Forward primer, 5′- GTGACGTTGACATCCGTAAAGA -3′ |
Reverse primer, 5′- GCCGGACTCATCGTACTCC -3′ |
Western blotting
Western blotting was performed as described previously. To extract proteins, embryos were sonicated in ice-cold lysis buffer (Cell Signaling Technology, Beverly, MA) with protease inhibitor cocktail (Sigma). Equal amounts of protein from different experimental groups and the Precision Plus protein standards (Bio-Rad Laboratories, Hercules, CA) were resolved by sodium dodecyl sulfate-polyacrylamide gel electrophoresis, transferred onto polyvinyl difluoride membranes, and then immunoblotted by primary antibodies at 1:1000 dilutions in 5% nonfat milk. Antibodies to protein DNA methyltransferase-1, DNA methyltransferase-3a, and DNA methyltransferase-3b were purchased from Cell Signaling Technology.
Horseradish peroxidase–conjugated goat antirabbit, goat antimouse (Jackson ImmunoResearch Laboratories, West Grove, PA) or goat antirat (Chemicon, Temecula, CA) secondary antibodies at 1:1000 were used. The intensity of the target protein bands were identified by densitometry and normalized by the densities of β-actin (Abcam, Cambridge, United Kingdom). Signals were detected by SuperSignal West Femto maximum sensitivity substrate kit (Thermo Scientific), and chemiluminescence emitted from bands was captured by a UVP Bioimage EC3 system (Upland, CA). All experiments were repeated 3 times with the use of independently prepared tissue lysates.
Measurement of DNA methyltransferase activities
DNA methyltransferase activities were measured by an EpiQuik DNA methyltransferase activity/inhibition assay kit (Epigentek, Farmingdale, NY) according to the manufacturer’s instructions. Briefly, we first extracted nuclear from embryonic day 8.5 embryos and then incubated nuclear with substrate and assay buffer for 1 hour, later added capture antibody for wash, after the wash added detection antibody, and finally added fluorodeveloping solution for fluorescence development and measurement.
Detection of global DNA methylation level
The global DNA methylation level was detected by a MethylFlash methylated DNA quantification kit (Colorimetric; Epigentek, Farmingdale, NY) according to the manufacturer’s instructions. In brief, we extracted genomic DNA from embryonic day 8.5 embryos and then bound DNA to the assay well, washed the well and added the capture antibody, washed the well again and added detection antibody and enhancer solution, and finally added color developing solution for color development and measurement.
Methylation-specific PCR
DNA methylation patterns in the CpG islands of Grhl3 , Pax3 , and Tulp3 genes were determined by methylation-specific polymerase chain reaction. Methylation-specific polymerase chain reaction distinguishes unmethylated from methylated alleles in a given gene based on sequence changes produced after bisulfite treatment of DNA, which converts unmethylated cytosines to uracil, and subsequent polymerase chain reaction using primers designed for either methylated or unmethylated DNA. Polymerase chain reaction was performed with 3.0 μL of bisulfite-modified DNA template in a 25 μL reaction. The CpG islands were identified on the Li Lab website ( www.urogene.org ). Primer sequences are listed in Table 2 .
Primers name | Primer sequences |
---|---|
Grhl3 | Left M primer, 5′- TTAAAGCGTAACGTAGAGTAAACGT -3′ |
Right M primer, 5′- ACCTCGATATACTAAAAAAACCGAA -3′ | |
Left U primer, 5′- TTTAAAGTGTAATGTAGAGTAAATGT -3′ | |
Right U primer, 5′- ACCTCAATATACTAAAAAAACCAAA -3′ | |
Pax3 | Left M primer, 5′- GTATTGTGTTCGTTTTTTCGTTTC -3′ |
Right M primer, 5′- GCTACGTAAATAATTCTACCCCGA -3′ | |
Left U primer, 5′- TTGTGTTTGTTTTTTTGTTTTGTTT -3′ | |
Right U primer, 5′- ACTACATAAATAATTCTACCCCAAAC -3′ | |
Tulp3 | Left M primer, 5′- TTTTCGATTTTTTTATTTGTAATGC -3′ |
Right M primer, 5′- CAACTCAATTCTAATCCTACTCGTA -3′ | |
Left U primer, 5′- TTTGATTTTTTTATTTGTAATGTGT -3′ | |
Right U primer, 5′- CCAACTCAATTCTAATCCTACTCATA -3′ |
Statistical analysis
Sample sizes were preestimated based on our previous studies before experiments were performed. Data on neural tube defect rates of each experimental group were analyzed by Fisher exact test or a χ 2 test. Data on protein and messenger RNA expression were presented as means ± SE. A one-way analysis of variance was performed using the SigmaStat 3.5 software (Systat Software Inc, San Jose, CA) followed by a Tukey test to estimate the significance of results ( P < .05).
Results
Epigallocatechin gallate ameliorates maternal diabetes–induced neural tube defects
Mouse embryonic neurulation occurs during embryonic day 8.5–10.5. To determine whether Epigallocatechin gallate treatment could ameliorate maternal diabetes–induced neural tube defects, concentrations of either 1 μM or 10 μM Epigallocatechin gallate were given to wild-type nondiabetic and diabetic pregnant mice at embryonic day 5.5 in drinking water.
The neural tube defect rate in embryos from diabetic dams was significantly higher than that in embryos from nondiabetic dams, with or without Epigallocatechin gallate treatment ( Table 3 ). Treatment with 10 μM Epigallocatechin gallate dramatically decreased neural tube defect formation in embryos from diabetic dams, compared with untreated diabetic dams ( Table 3 ). Treatment with 1 μM Epigallocatechin gallate did not reduce maternal diabetes–induced neural tube defects significantly. Therefore, 10 μM Epigallocatechin gallate was used in subsequent experiments.
Group | Total embryos | Embryos with NTD | Blood glucose levels | NTD rate, % |
---|---|---|---|---|
Nondiabetic, no EGCG (n = 12) | 85 | 0 | 167.71 ± 7.1 | 0 |
Nondiabetic, 1 μM EGCG (n = 4) | 27 | 0 | 157.3 ± 8.2 | 0 |
Nondiabetic, 10 μM EGCG (n = 4) | 24 | 0 | 152.5 ± 11.3 | 0 |
Diabetic, no EGCG (n = 12) | 78 | 23 | 442.2 ± 14.7 | 29.5 a |
Diabetic, 1 μM EGCG (n = 7) | 46 | 11 | 417.5 ± 18.4 | 23.9 a |
Diabetic, 10 μM EGCG (n = 8) | 51 | 1 | 406.0 ± 20.1 | 2.0 |
a Significant difference compared with the other groups in χ 2 test ( P < .05). The diabetic no Epigallocatechin gallate group is significantly different when compared with the nondiabetic no Epigallocatechin gallate, nondiabetic 1 μM Epigallocatechin gallate, nondiabetic 10 μM Epigallocatechin gallate, and diabetic 10 μM Epigallocatechin gallate groups. The diabetic 1 μM Epigallocatechin gallate group and the diabetic no Epigallocatechin gallate group are not significantly different, and the diabetic 1 μM Epigallocatechin gallate group is significantly different when compared with the nondiabetic no Epigallocatechin gallate, nondiabetic 1 μM Epigallocatechin gallate, nondiabetic 10 μM Epigallocatechin gallate, and diabetic 10 μM Epigallocatechin gallate groups.
Epigallocatechin gallate inhibits maternal diabetes–increased DNA methyltransferase expression
To assess whether Epigallocatechin gallate treatment prevents diabetes–induced neural tube defects by regulating embryonic DNA methylation level, we first examined the DNA methyltransferase expression level in embryos from diabetic dams, with or without Epigallocatechin gallate treatment. Messenger RNA expression of Dnmt1 and Dnmt3a did not differ in embryos from both the nondiabetic and diabetic groups, regardless of Epigallocatechin gallate treatment ( Figure 1 A). However, Dnmt3b messenger RNA expression was significantly higher in embryos from the diabetic group than in embryos from the nondiabetic group, with or without Epigallocatechin gallate treatment. We also observed that Epigallocatechin gallate treatment blocked maternal diabetes–induced Dnmt3b expression ( Figure 1 A).
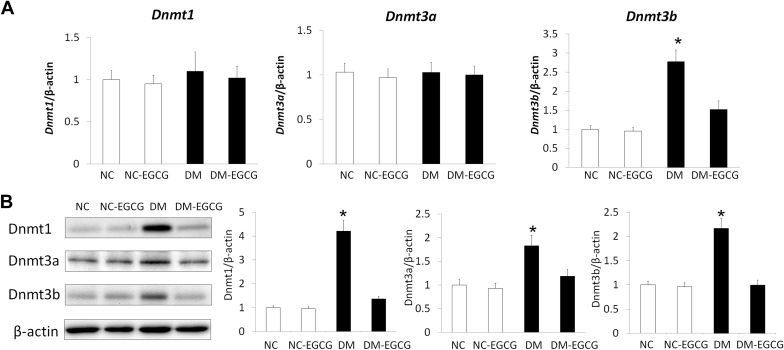
We next examined the DNA methyltransferaseprotein expression by immunoblotting. The levels of all 3 DNA methyltransferases, DNA methyltransferase-1, DNA methyltransferase-3a, and DNA methyltransferase-3b, were significantly higher in embryos from the diabetic group than that in embryos from the nondiabetic group. Treatment with 10 μM Epigallocatechin gallate suppressed maternal diabetes–increased DNA methyltransferase protein expression, whereas Epigallocatechin gallate treatment did not further suppress DNA methyltransferase protein expression in embryos from the nondiabetic group ( Figure 1 B). These data suggested that Epigallocatechin gallate treatment inhibits maternal diabetes–increased DNA methyltransferase expression.
Epigallocatechin gallate reduces maternal diabetes–increased DNA methyltransferase activity and global methylation levels
Total DNA methyltransferase activity was determined in embryos from diabetic dams vs nondiabetic dams. DNA methyltransferase activity was dramatically increased in embryos from diabetic dams compared with those in embryos from nondiabetic dams, with or without Epigallocatechin gallate treatment. However, the DNA methyltransferase activity in embryos from diabetic dams treated with 10 μM Epigallocatechin gallate was similar to those seen in embryos from nondiabetic dams, with or without Epigallocatechin gallate treatment, and was significantly lower than that in the diabetic group without Epigallocatechin gallate treatment ( Figure 2 A).
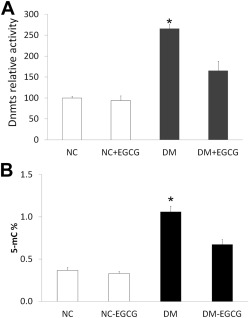
We tested whether Epigallocatechin gallate treatment could affect global DNA methylation levels in embryos from diabetic dams. Consistent with our observation of DNA methyltransferase activity, maternal diabetes increased global DNA methylation levels in embryos from the diabetic group, compared with the nondiabetic group, and Epigallocatechin gallate treatment blocked maternal diabetes–increased global DNA methylation levels ( Figure 2 B).
Epigallocatechin gallate decreases methylation in the CpG islands of neural tube closure essential genes
Hypermethylation of CpG islands in the promoter regions of genes involved in embryogenesis is an important mechanism to silence gene expression. To determine whether maternal diabetes induces hypermethylation of CpG islands in the promoters of neural tube closure essential genes, we measured DNA methylation in the CpG islands of several neural tube closure essential genes, including Grhl3 , Pax3 , and Tulp3 . CpG islands were identified the promoter regions of the Grhl3 , Pax3 , and Tulp3 gene ( Figure 3 A ). We defined a CpG island using the following criteria: size >200 bp, guanine-cytosine percentage >50%, CpG observed/CpG expected >0.6.
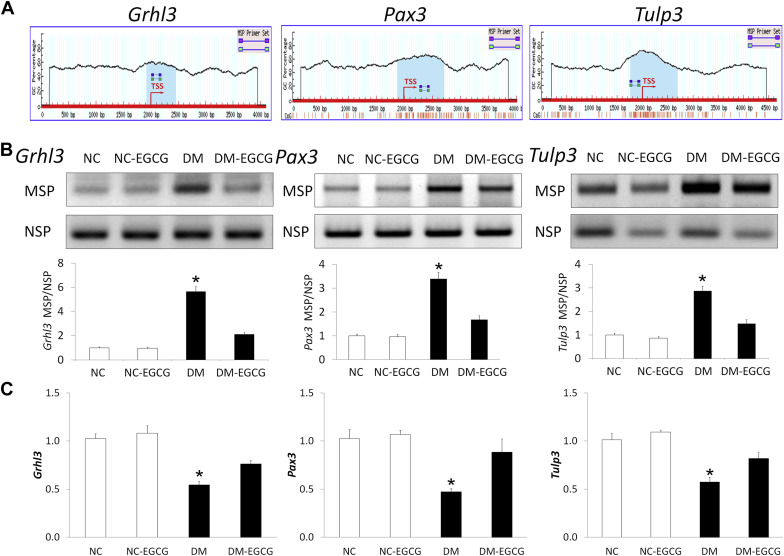
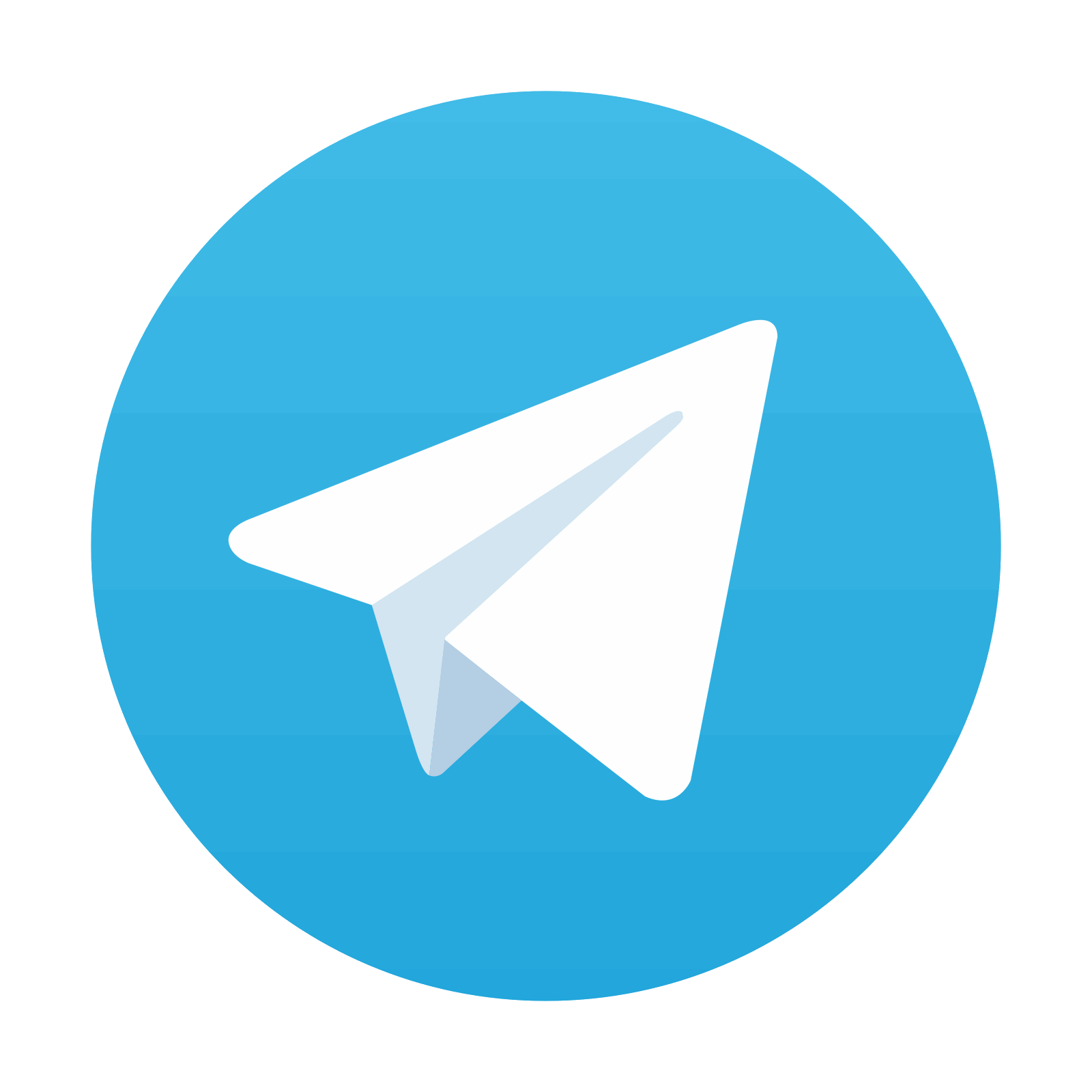
Stay updated, free articles. Join our Telegram channel

Full access? Get Clinical Tree
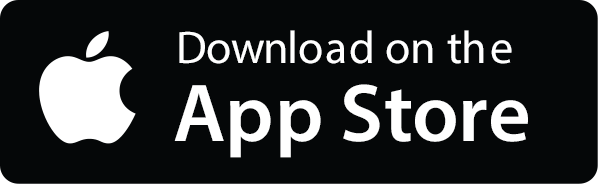
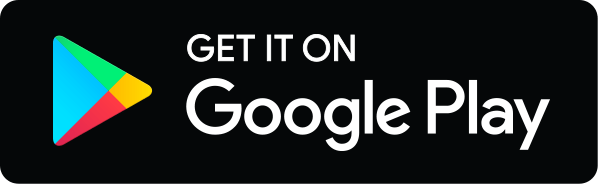