Since chemotherapeutic agents were first administered for the treatment of malignancy, infection has been a cause of significant morbidity and mortality. Initial reports documented an infection-related mortality rate among patients with acute leukemia that was greater than 70%. In the past five and one-half decades, cancer survival rates have improved dramatically in high-income countries, with more than 80% of children with cancer surviving beyond 5 years from diagnosis. However, the immunosuppressive consequences of chemotherapy continue to exist, and infections still account for a substantial number of deaths in children receiving intensely myelosuppressive chemotherapy, especially in the 2 weeks after diagnosis.
For many decades, researchers have sought to identify risk factors for infection and to devise risk-stratified therapeutic approaches to reduce infectious mortality rates in patients receiving chemotherapy. Early descriptive pediatric studies confirmed that the depth and duration of neutropenia was a risk factor for developing infection. Furthermore fever was a frequent and sometimes the only clinical sign indicating the presence of infection in this at-risk population. In these early years, fever in patients with neutropenia prompted an infectious evaluation, but empiric antibiotic therapy was not considered standard of care. Because infection was the most common cause of fever in neutropenic patients and because some patients with fever and neutropenia had rapid progression to death, particularly due to Pseudomonas aeruginosa bacteremia, empiric therapy was explored. In 1971, Schimpff et al. published one of the sentinel experiences of combination empiric therapy with carbenicillin and gentamicin in a series of patients with fever and neutropenia. Their efforts revealed a reduction in infectious mortality with this approach relative to historical controls. Specifically, in the historical control period, when empiric antibiotic therapy was not utilized, 20 of 22 P. aeruginosa infections ultimately resulted in death. This compared to only four deaths out of 21 P. aeruginosa infections during the study period. Subsequent landmark trials by Pizzo et al. supported the continuation of empiric antibiotics through the duration of neutropenia and prompted the addition of empiric antifungal therapy in the setting of prolonged fever and neutropenia despite broad-spectrum antibiotic therapy.
In the decades that followed, empiric antibacterial and antifungal therapy has evolved into standard of care for patients with fever and neutropenia. Clinical practice guidelines from various societies have better standardized the approach to this clinical scenario. Although these guidelines have been useful, they have primarily focused on adult patient populations. This has left pediatricians to apply guidelines that have not accounted for important differences between children and adults presenting with fever and neutropenia. Fortunately, in 2012, an international group of investigators formed the Pediatric Fever and Neutropenia Guideline Panel with the objective of systematically reviewing the available literature to inform an evidence-based guideline for the management of the child with fever and neutropenia. The initial version of this guideline addressed a number of the important differences in the management of pediatric and adult patients with fever and neutropenia. In addition to providing guidance on current management practices, the committee identified knowledge gaps with the goal of directing future investigation that will provide further data to improve the breadth of evidence-based clinical practice.
This chapter focuses on the epidemiology of fever and neutropenia and approaches for risk stratification that are pertinent to pediatric patients. Advances in modalities for diagnosing bacterial and fungal infections are also explored. Finally empiric, preemptive, and prophylactic therapeutic interventions are reviewed. At relevant points, areas for necessary future investigation are highlighted. There are many other etiologies of neutropenia (e.g., congenital neutropenia and autoimmune neutropenia), and such patients will also incur periods of fever and neutropenia. However, the importance of fever in these settings varies substantially and thus the focus of this chapter is on fever in the setting of chemotherapy-induced neutropenia.
Epidemiology of Fever and Neutropenia
Children receiving chemotherapy are often confronted with at least one episode of fever and neutropenia during the course of their cancer care. The frequency of fever and neutropenia varies depending on factors such as type and extent of malignancy, intensity of chemotherapy, and receipt of prophylactic granulocyte colony-stimulating factor, and the incidence can range from 10% to 60%. The incidence of fever and neutropenia can increase to 70% to 100% in patients receiving particularly intensive and myelosuppressive chemotherapy.
Bacterial Pathogens
Fever in the setting of neutropenia is often the initial and potentially the only clinical sign of a bacterial, fungal, or viral infection. Of patients in whom a pathogen is identified, bacteria predominate. In a landmark study evaluating 793 pediatric cancer patients with fever and neutropenia, Pizzo et al. found that 87% of patients with a microbiologically documented infection had a bacterial pathogen identified. Recent epidemiologic studies specific to pediatric fever and neutropenia have suggested a reduction in the proportion of episodes that are attributable to bacteria. However, despite the reduction, bacterial pathogens still accounted for the majority of all identified pathogens.
Early reports on the epidemiology of these bacterial infections highlighted gram-negative pathogens as the predominant cause of infection in neutropenic children and adults. Escherichia coli, Pseudomonas aeruginosa, and Klebsiella spp. were the three most commonly isolated pathogens. Subsequently, towards the end of the 1980s, gram-positive pathogens were being increasingly recognized as an important source of fever of neutropenia, and, soon thereafter, gram-positive pathogens were found to be more common than gram-negative organisms. This shift in pathogens has been attributed to increased use of central venous lines, a greater prevalence of mucositis, and prophylactic regimens with predominantly gram-negative activity. Additionally, escalation to more intensive chemotherapy regimens, such as those containing high-dose cytarabine, have resulted in more impressive mucositis, which has contributed to increases in specific pathogens such as viridans group streptococci.
The spectrum of bacterial pathogens will depend on specific chemotherapy, use of antimicrobial prophylaxis, and setting. Table 68.1 displays the distribution of bacterial infections across various international pediatric oncology cohorts. Variation in the frequency in isolation of a bacterial pathogen across cohorts is apparent. This is likely secondary to variation in geographic location, composition of each cohort, diagnostic testing practices, and prophylactic antimicrobial regimens. With the exception of the Malaysian cohort, the predominance of gram-positive pathogens has persisted in pediatric oncology cohorts, consistent with data published toward the end of the previous century. Among the gram-positive pathogens viridans group streptococci, Staphylococcus aureus, and coagulase-negative staphylococci are predominant. E. coli, P. aeruginosa, and Klebsiella spp. remain the most commonly identified gram-negative pathogens.
Ariffin (2002) | Lehrnbecher (2004) | Castagnola (2007) | Hakim (2009) | Gupta (2011) | |
---|---|---|---|---|---|
Study location | Malaysia | Germany | Italy | United States | El Salvador |
Patient type | Any malignancy | Acute myeloid leukemia | Leukemia, solid tumor, or allogeneic HSCT | Any malignancy | Any malignancy |
Clinical scenario | Fever and neutropenia | Fever with and without neutropenia | Fever and neutropenia | Fever and neutropenia | Fever and neutropenia |
Infection episodes (total patients) | 762 (513) | 855 (304) | 614 (NA) | 337 (337) | 106 (86) |
Bacterial pathogen isolation; N (%) | 270 (35.4%) | 252 (29.5%) | 97 (15.8%) | 54 (16%) | 23 b (22%) |
Gram-positive pathogens; n (%) | 103 (38.1%) | 203 (80.6%) | 57 (58.8%) | 31 a (57%) | 14 (61%) |
Gram-negative pathogens; n (%) | 167 (61.9%) | 49 (19.4%) | 40 (41.2%) | 23 (43%) | 11 (48%) |
a Includes seven episodes of Clostridium difficile infection.
Fungal Pathogens
As discussed in more detail later, the likelihood of invasive fungal disease (IFD) during an episode of fever and neutropenia varies depending on a number of risk factors. Generally fungal pathogens are considered as the etiology of fever and neutropenia when these two clinical signs are concurrently prolonged or when fever is recurrent during the same episode of neutropenia in the setting of broad-spectrum antibiotic therapy. Defining the epidemiology of IFD in specific patient populations can be challenging. Published consensus criteria for defining the presence an IFD have been helpful in standardizing reporting of such infections. However, limitations in diagnostic modalities for identifying an IFD persist and thus published IFD rates are likely underestimated. Despite these challenges, prospective multicenter data have documented a proven or probable IFD rate ranging from 3% to 5% of children hospitalized with fever and neutropenia. Candida spp. are the most commonly isolated fungal pathogens, likely related to the frequent existence of Candida as a commensal organism on the skin and mucosal surfaces. Compromise of these important anatomical barriers (e.g., mucositis) can result in invasive candidiasis. Additionally Candida spp. can infect central venous catheters, which are commonly present in children with cancer. Although mortality data specific to candidiasis in pediatric oncology patients are limited, the attributable mortality of invasive candidiasis in all pediatric patients has been estimated to be 10%.
Invasive mold disease (IMD) is less common but an extremely important complication of neutropenia, given the poor outcomes related to these pathogens. In two contemporary pediatric cases series, only 54% to 60% of patients with IMD responded to therapy in the first 12 weeks from diagnosis, and approximately 30% of patients diagnosed with an IMD died within the same time period. Among the mold pathogens, Aspergillus spp. are most common, followed by organisms of the Mucorales order.
Viral Pathogens
Owing to advancement in viral diagnostic methodologies, multiple publications have attempted to delineate the attribution of fever and neutropenia events to viral pathogens. Table 68.2 displays the frequency of recovered viral upper respiratory pathogens in pediatric patients presenting with fever, with or without symptoms of a viral infection. Although only Torres et al. was limited to episodes of fever and neutropenia, the majority of patients in the other cohorts had fever and neutropenia at the time of their evaluation. The frequency of laboratory-confirmed viral respiratory infection ranged from 8% to 59%. A more recent publication using administrative data from the Kid’s Inpatient Database suggested that up to 12.1% of fever and neutropenia episodes are the result of viral infection. However, in this study, the identification of fever and neutropenia admissions and presence of viral infection were dependent on ICD-9 discharge diagnosis codes. These codes have not been validated for either fever and neutropenia or viral infection, and thus it is difficult to ascertain the accuracy of these findings.
Long (1987) | Arola (1995) | Christensen (2005) | Koskenvuo (2008) | Torres (2012) | |
---|---|---|---|---|---|
Study duration | 5 years | 17 months | 12 months | 5.5 years | 21 months |
Patient type | Leukemia, solid tumors | Any malignancy | Any malignancy | Leukemia | Any malignancy |
Clinical scenario | Suspicion of virus | Fever | Fever | Fever | Fever and neutropenia |
Total patients (episodes) | 200 (NR) | 32 (75) | 66 (250) | 51 (138) | 193 (331) |
Testing methods | Culture; immunofluroesence | Culture; antigen; and antibodies | PCR | Culture; antigen; and PCR | PCR |
Respiratory virus isolation rate | 148 (NA) | 28 (37%) | 19 (8%) a | 61 (59%) | 190 (57%) |
Sterile site bacterial pathogen plus virus isolation | Not reported | None | Not reported | 13% | 33% |
a Mouth swabs and not nasopharyngeal swabs performed on a majority of patients.
Although some authors have suggested routine comprehensive viral testing at the time of presentation, the utility of routine viral testing is not clear. First, ideally, the identification of a viral pathogen should allow for discontinuation of broad-spectrum antibiotics in the febrile and neutropenic patient. However, bacterial and viral infections are not mutually exclusive, with as many as 13% to 33% of patients with a viral pathogen suffering a simultaneous sterile site bacterial infection. Second, the evolution in the availability of viral PCR testing may result in detection of virus well after clinical resolution. Finally, only a few antiviral agents are available for the treatment of some of these viral pathogens, and their effectiveness is not clearly delineated. Viral testing should be limited to patients in whom positive results would effect a change in management, such as deescalation of antibiotic therapy, initiation of an appropriate antiviral therapy (e.g., neuraminidase inhibitor for influenza), or institution of appropriate isolation precautions.
Fever and Neutropenia of Unknown Origin
Despite evaluation, many patients have negative cultures and do not have a clinically evident site of infection. This presentation is often referred to as fever of unknown origin (FUO). Although children with FUO can have an occult infection, an infectious pathogen or clinical infection is not detected. In a combined pediatric and young adult cohort, Pizzo et al. described 306 consecutive episodes of fever and neutropenia in patients cared for at the National Institute of Health. They found that only 54% of patients had an identifiable infection within 7 days from presentation. Despite advances in infectious diagnostic modalities (i.e., improved blood culture mechanisms, sensitive viral tests), the rates of unidentified etiology of fever and neutropenia in children have remained high, ranging from 33% to 79%.
Risk Stratification
Risk Stratification at Initial Presentation
The risk of infection and serious morbidity is highly heterogeneous among patients with fever and neutropenia, and this heterogeneity has resulted in efforts to risk stratify patients. Most of the efforts in risk stratification have been directed toward identifying patients at low risk of bacteremia and adverse outcomes who may be managed with less aggressive interventions such as outpatient management, early discontinuation of antibiotics, or oral therapy. Some studies have focused on identifying high-risk patients to facilitate intensification of diagnostic investigation, empiric therapy, or monitoring. In adults, the predominant risk stratification schema that has emerged is the Multinational Association for Supportive Care in Cancer (MASCC) Scoring System. Unfortunately this system incorporates parameters that are not applicable to children, such as lack of chronic obstructive pulmonary disease and age less than 60 years, to determine low-risk status. The inclusion of these parameters limits the applicability of this scoring system to children.
A number of both retrospective and prospective pediatric cohort studies have been performed to evaluate various clinical prediction rules for designating children as low-risk for infection in the setting of fever and neutropenia. These studies have been summarized in multiple meta-analyses. Considered collectively, data from these studies support the potential success of implementing a risk stratification protocol. However, each trial used varied patient-relevant factors (e.g., age, malignancy), clinical factors (e.g., timing of chemotherapy, degree of fever, presence of hypotension), and laboratory parameters (e.g., duration and depth of neutropenia, C-reactive protein value, platelet count) and had various outcome definitions (e.g., bacteremia, serious infection, death). Furthermore attempts to validate six of the pediatric risk stratification schemas in separate populations have resulted in suboptimal performance compared to the original study. *
* References .
A primary reason for suboptimal performance during validation is variation in the clinical setting between the derivation and validation cohorts. Currently centers applying a risk prediction model for children with fever and neutropenia should identify a model that is most consistent with their patient population (rules tend to perform less favorably when applied in different settings) and their local clinical practice and is most feasible to implement. Additionally, at the time of implementing a risk stratification protocol, a center should clearly delineate the goals of its risk stratification. This articulation should include a framework on how the risk stratification will inform clinical decision-making (i.e., low-risk patients can be discharged to home with outpatient follow-up). The framework should be developed by a multidisciplinary team and clearly communicated to both health care providers and patients and their families.More recently an individual patient data meta-analysis inclusive of data from 22 datasets representing previous cohorts of children with fever and neutropenia was performed as part of a global collaboration called Predicting Infectious Complications in Children with Cancer (PICNICC). The analysis considered demographic, clinical, and laboratory results in deriving a risk prediction model for likelihood of microbiologically defined infection in children with fever and neutropenia. The final model included malignancy type, temperature, clinical appearance of the child, hemoglobin level, total white blood cell count, and absolute monocyte count. The resultant prediction rates for microbiological infection closely correlated with actual estimates, and the model was robust to internal validation. Because the data that informed this model derivation were assembled from cohorts across various clinical settings, it represents a potential tool to be leveraged in various fever and neutropenia settings. Prior to widespread application, it needs to be validated externally.
As biomarkers continue to be discovered, there is always the potential to improve on current prediction rules. Investigators have studied the clinical utility of more than 170 different biomarkers, including cytokines, cell surface markers, coagulation system molecules, receptors in various inflammatory cascades, and acute phase reactants in various pediatric and adult clinical settings. A number of biomarkers have emerged as potentially useful tools to guide approaches for fever and neutropenia, some of which have already been used in the aforementioned prediction rules. However, although some biomarkers are often elevated in patients with a bacterial infection, an inability to identify a threshold value for a single biomarker to accurately discriminate between infected and uninfected patients has limited their clinical applicability. Future investigations that incorporate multiple biomarkers with traditional laboratory values and clinical findings may improve the discriminating ability of a pediatric risk prediction rule.
Risk Stratification for Invasive Fungal Disease
The risk stratification prediction rules just discussed are typically relevant to risk for microbiologically documented infections at the time of presentation for fever and neutropenia. However, concern for an IFD is often increased when fever and neutropenia persist despite initial empiric antibiotic therapy. Unlike the risk for microbiologically documented infection at presentation, there have been no pediatric studies dedicated to deriving an IFD risk prediction rule in children with persistent fever and neutropenia. However, a number of observational studies have identified important factors that are associated with an increased risk for IFD.
Patient populations with reported IFD incidence greater than 10% include children with acute myeloid leukemia (AML), relapsed acute lymphoblastic leukemia (ALL), and recipients of an allogeneic stem cell transplantation. Additionally increasing duration of neutropenia is a commonly identified risk factor for IFD. The exact duration at which the risk significantly increases is not definitively known but observational data in children with AML suggest it is somewhere between 10 and 15 days of neutropenia. Depth of neutropenia is also an important component of the neutropenia risk profile for IFD. An absolute neutrophil count (ANC) of less than 100/µL or an absolute monocyte count (AMC) of less than 100/µL during an episode of fever and neutropenia was each associated with an increased risk of IFD. Exposure to high-dose steroids and/or prolonged steroid exposure (>10 days) have also been identified as a risk factor for IFD. Finally increasing age has been associated with increasing risk for IFD. Age thresholds of 7.5 years and 10 years have been suggested for dichotomizing IFD risk by age. However, an optimal threshold for categorizing age related to IFD risk is unclear.
Based on these data, pediatric fever and neutropenia guidelines recommend that patients with fever and neutropenia should be considered IFD high risk if they have recently received intensive chemotherapy (e.g., AML or relapsed ALL patient) with expected prolonged neutropenia and/or recent exposure to prolonged courses of steroids. This stratification for identification of IFD high-risk patients is a general guide, and it should be noted that IFD has been described in patients who would not meet this high-risk classification. Additional work is necessary to establish specific prediction rules to incorporate multiple risk factors into a single risk prediction rule to more accurately discriminate between IFD high- and low-risk patients.
History and Physical Exam
Prior to initiating routine diagnostic testing, a complete history and physical exam should be performed. A comprehensive history can identify important exposures that could alert the physician to consider less common opportunistic infections and direct early additional diagnostic testing. The history should include details about symptoms other than fever, adherence to current medications (especially prophylactic antimicrobials), and most recent chemotherapy regimen. Additionally the clinician should ask about sick contacts at home, updates on recent travel, daycare/school/occupational exposures, animal exposures (e.g., new pets such as cats or birds, or contact with farm or wild animals), and dietary exposures (e.g., unpasteurized cheeses). A thorough history of prior dwelling locations is becoming increasingly important among pediatric oncology patients in the evolving era of country-to-country transfers. Knowledge on prior living locations may have an impact on a patient’s risk for multidrug-resistant pathogens and help to identify potential dietary or environmental exposures that predispose patients to rarely seen pathogens. On physical exam, specific attention should be focused on areas sometimes overlooked, such as the naso- and oropharynx, middle ears, genitalia, perineum (in particular, the perianal area), and scalp. Central venous line exit sites are also important areas for inspection.
Initial Diagnostic Evaluation
Regardless of the risk designation of a pediatric patient presenting with fever and neutropenia, an infectious diagnostic evaluation is warranted in all such patients. Various testing modalities may be employed depending on the status of the presenting patient. In all patients, routine blood cultures should be performed prior to initiation of antibiotics. Because most patients have central venous access, this would include culturing all lumens of the central venous catheter.
Performing a peripheral blood culture in addition to central venous cultures is controversial. Multiple studies of paired cultures taken from pediatric and adult patients with underlying malignancy suggest a small but consistent probability of detecting true bacteremia from the peripheral culture when the central culture is negative. A meta-analysis determined that 13% of bacteremias were only identified with a peripheral culture. These data suggest that a peripheral blood collection should be considered concurrent with cultures from the central line to optimize detection of a causative pathogen for the fever and neutropenia episode.
The yield of repeated daily blood cultures after the initial evaluation is low. In a series of 193 consecutive fever and neutropenia episodes in 117 pediatric patients, 95 of the episodes revealed that the index culture was negative and the fever and neutropenia persisted. Among these 95 episodes, 421 additional blood cultures were obtained in the ensuing 2 weeks. Only 4 of the 421 additional blood cultures were positive for a bacterial pathogen. Given the low yield of subsequent blood cultures in the setting of persistent fever and neutropenia with an initial negative culture, it is reasonable to follow the adult guideline recommendations, which suggest daily blood cultures for only the first 3 days of fever and neutropenia. Any additional blood cultures should be reserved for patients with a significant clinical change (e.g., hypotension, tachypnea) or in those whose fever initially resolved but then recurred.
A urine culture is warranted if the patient presents with signs or symptoms of a urinary tract infection (UTI). The importance of routine urine cultures for children presenting with fever and neutropenia without signs or symptoms of a UTI is controversial. In a typical pediatric population, UTI is a common source of FUO. Asymptomatic UTIs are likely to be more common in neutropenic children given the lack of an appropriate inflammatory response. This is evidenced by the fact that only 4% of oncology patients with a urine culture yielding a UTI pathogen will have pyuria on urinalysis.
A number of observational studies have evaluated the yield of urine cultures in pediatric patients presenting with fever and neutropenia. Hakim et al. noted that of 337 patients presenting with fever and neutropenia 2 (0.6%) had a positive urine culture. Data from Santolaya et al. revealed the presence of a urinary pathogen in 4.6% of fever and neutropenia episodes. Urine culture was not a component of the routine diagnostic evaluation in either study, so it is possible that there were more patients with a UTI that went undetected. When urine culture is routinely performed in children presenting with fever and neutropenia, the frequency of a UTI is reported to be 8.6%.
Given these data, urine culture in patients presenting with fever and neutropenia should be obtained if a mid-stream or clean catch specimen is feasible. In patients who require urinary catheterization for specimen collection, the risks of the intervention should be weighed against the benefit of potentially identifying the source of the fever. However, antibiotic administration should never be delayed if a patient is unable to provide a timely urine specimen.
In children presenting with focal respiratory signs (e.g., tachypnea or auscultatory changes) or symptoms (e.g., cough or increased work of breathing), chest radiography should be a component of the initial diagnostic workup. Additionally, in children with respiratory symptoms who are capable of producing an adequate sputum sample, the clinician should consider sputum collection for culture. The utility of chest radiography has been examined in children presenting with fever and neutropenia without respiratory symptoms. Together these studies evaluated 487 episodes of pediatric fever and neutropenia; only five chest radiographs revealed pneumonia in the absence of any respiratory signs or symptoms. These data do not support the routine use of chest radiography in children with fever and neutropenia in the absence of signs or symptoms of respiratory issues.
Finally important findings noted on history and/or physical exam should dictate additional testing for less common sources of infection. For instance a patient presenting with fever and neutropenia and a reported history of a lovebird in the home should prompt additional testing for cryptococcosis early in the evaluation period. Likewise identification of black cutaneous lesion(s) in a patient with antecedent neutropenia should prompt consideration for immediate biopsy to determine if the lesion represents a cutaneous mold infection. In the setting of exotic exposures or important clinical findings, consultation with an infectious disease physician with experience in immunocompromised patients may be warranted early in the hospitalization because early identification of less common opportunistic infections will likely translate into improved patient care.
Initial Therapy for Fever and Neutropenia
Traditionally the standard of care for fever and neutropenia has been hospital admission with administration of intravenous broad-spectrum antibiotics. However, employing a risk stratification rule (as discussed in the previous Risk Stratification section) offers the opportunity to differentially manage children at low risk of having bacteremia or an adverse outcome at the time of presentation for fever and neutropenia. Low-risk patients may be managed with oral antibiotics if oral therapy is feasible (e.g., absence of vomiting and malabsorption) in the inpatient or outpatient setting. If the hospital infrastructure supports an ambulatory approach, then patients may be treated as outpatients with oral or intravenous therapy. Those who are not identified as low risk should be admitted for initiation of a parenteral antibiotic regimen with broad-spectrum gram-negative coverage, including P. aeruginosa and gram-positive coverage for organisms such as the viridans group streptococci. Antibiotic options that meet these criteria include an anti-pseudomonal penicillin (e.g., piperacillin-tazobactam), a cephalosporin (e.g., cefepime), or a carbapenem (e.g., meropenem or imipenem). Two separate pediatric meta-analyses compared anti-pseudomonal penicillins to cephalosporins, as well as anti-pseudomonal penicillins to carbapenems, and concluded similar effectiveness of each of these options for empiric therapy.
It is important to note that a meta-analysis of predominantly adult randomized controlled trials (RCTs) identified a significant increased risk for all-cause mortality in patients receiving cefepime compared to those receiving other β-lactam agents for fever and neutropenia. However, in a subsequent analysis, the US Food and Drug Administration (FDA) supplemented the available published data with unpublished mortality data specific to febrile neutropenic patients and found no mortality difference between the antibiotic options. Importantly the FDA specifically reviewed the available case report forms from the fever and neutropenia trials and could not identify a biologically plausible reason for the previously identified association between cefepime and death. An additional meta-analysis of fever and neutropenia trials, as well as a retrospective investigation of pediatric AML patients, did not reveal a difference in mortality risk between those exposed to cefepime compared to other antibiotics commonly used for fever and neutropenia. Evaluating the available data, cefepime should remain as a viable option for empiric therapy for febrile neutropenia.
In general, for a child presenting with fever and neutropenia that is otherwise stable, monotherapy with an antipseudomonal penicillin, an antipseudomonal fourth-generation cephalosporin, or an antipseudomonal carbapenem is appropriate. An empiric monotherapy regimen is supported by data from recent meta-analyses in pediatric fever and neutropenia that all found no statistically significant differences in treatment failure, infection-related mortality, or overall mortality when comparing monotherapy with combination regimens. Both the current pediatric and adult fever and neutropenia guidelines support empiric anti-pseudomonal monotherapy.
Because gram-positive organisms are an increasing cause of fever and neutropenia episodes, the utility of adding additional broad gram-positive coverage (e.g., vancomycin) to the empiric regimen has been questioned. Although pediatric-specific data do not exist, multiple adult RCTs have investigated the efficacy of adding a glycopeptide to various β-lactam–containing regimens. These trials were summarized in two separate meta-analyses, each of which failed to identify a significant benefit relative to all-cause mortality in patients receiving empiric glycopeptide therapy. Therefore routine empiric glycopeptides are not recommended for fever and neutropenia.
As suggested by the adult and pediatric fever and neutropenia guidelines, certain patient-specific factors should be evaluated when managing antibiotic regimens. For instance, in patients presenting or developing hemodynamic instability, broadening the coverage to include resistant gram-negative and -positive organisms, such as the addition of a second gram-negative agent (e.g., an aminoglycoside) and vancomycin, is important. Additionally broad gram-positive therapy should be considered in patients with evidence of a focal gram-positive infection (e.g., cellulitis or central access tunnel infection). Finally a history of an invasive infection with a resistance organism (e.g., methicillin-resistant S. aureus [MRSA]) should inform empiric antibiotic choices during subsequent fever and neutropenia episodes.
These recommendations for empiric antibiotic coverage should be considered guiding principles rather than dictums. Each center’s local empiric antibiotic regimen should be customized based on information regarding pathogen resistance from the institution’s antibiogram that is specific to the oncology patient population. For example, viridans group streptococci are a common group of pathogens that result in bacteremia and sepsis in AML patients. The resistance profile of these organisms is variable over time and by institution. A center may have elevated MRSA rates, and thus vancomycin would be a reasonable component to the empiric therapy regimen for fever and neutropenia until at least the initial blood culture results are available. The threshold of resistance that would trigger a change in the locally recommended empiric therapy regimen is not known. Such decisions regarding empiric regimen changes should be made with input from oncologists, infectious disease specialists, microbiologists, and pharmacists.
For low-risk patients, outpatient management with either oral or parenteral therapy for fever and neutropenia has garnered much support in recent years. A number of pediatric-specific randomized trials have noted similar safety for early hospital discharge on intravenous therapy versus continued hospitalization, early hospital discharge on oral therapy versus continued hospitalization, inpatient intravenous versus inpatient oral therapy, and outpatient intravenous versus outpatient oral therapy. Although each of these studies was limited by small sample sizes, a pediatric-stratified analysis of randomized trials and a pediatric-specific meta-analysis of prospective studies support the safety of outpatient management and oral antibiotic administration in low-risk children with fever and neutropenia. In addition, outpatient management of low-risk febrile neutropenic patients is associated with a large reduction in health care costs compared with inpatient management. Patients and parents both report that the home environment has a positive impact on physical activity, self-esteem, and family activities. However, the acceptance of an outpatient management plan by patients and their caregivers is limited by the perception of reduced effectiveness of outpatient management and the potential burden of outpatient care. In a discrete choice experiment, Sung et al. found that an outpatient management plan that required three times a week clinic visits or a risk for readmission that was 7.5% or greater would not be tolerable to patients and families. These data highlight that as the standard of care for low-risk fever and neutropenia transitions from the inpatient to outpatient setting, it will be necessary to include families and patients in care decisions.
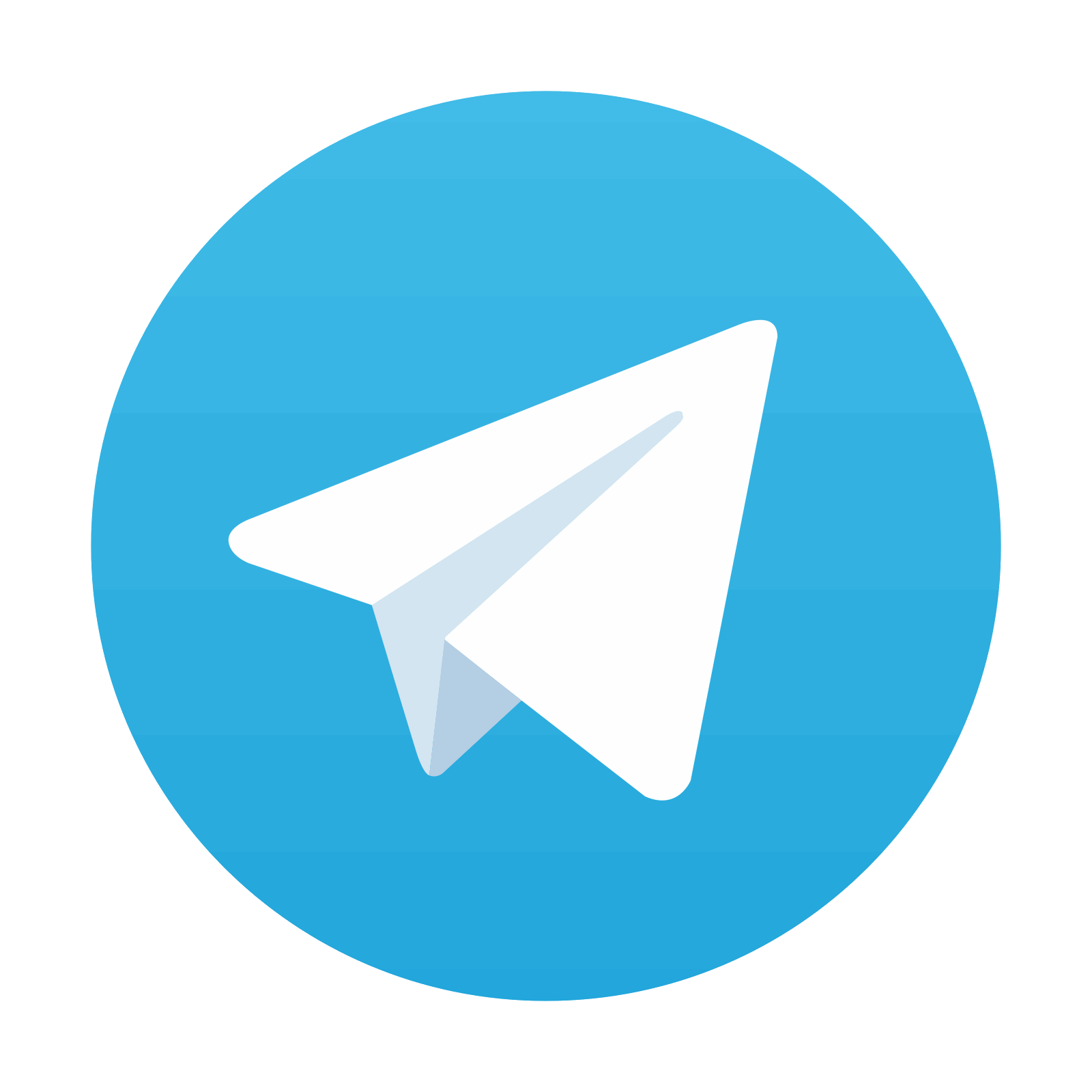
Stay updated, free articles. Join our Telegram channel

Full access? Get Clinical Tree
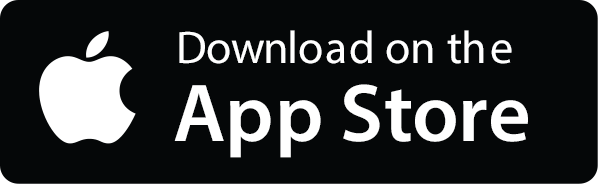
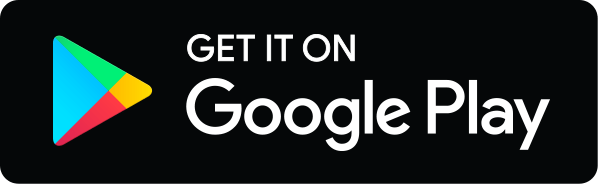