The Excretion of Drugs and Chemicals in Human Milk
Cheston M. Berlin Jr.
Introduction
During the 1960s and the early 1970s, the incidence of breastfeeding reached a nadir; in 1972 only 20% of newborns discharged from nurseries were breast-fed, and only 15% were still being breast-fed at 6 months of age (1). Both medical and psychological studies have emphasized the considerable biologic and psychological benefits for breastfeeding infants (2,3). The last 35 years has seen a great increase in the incidence of breastfeeding due to the enthusiastic efforts of both professional (American Academy of Pediatrics) and lay organizations (La Leche League International) with support from the Federal Government (4). The most recent data from the National Health and Nutrition Examination Surveys (NHANES) for 2005 to 2006 show that 77% of infants are discharged from nurseries breastfeeding and at 6 months of age 35% are still being breast-fed (5). Lactating mothers take significantly more medications than pregnant mothers (6). The medications most often used by breastfeeding mothers are multivitamins, nonsteroidal anti-inflammatory drugs, acetaminophen, progestins, antibiotics, and decongestants.
With this increased emphasis and interest in breastfeeding, there has been a parallel increase in concern over the excretion of drugs and chemicals into breast milk. It is very important that this concern, while appropriate, not translate into discouraging nursing when it is necessary for a mother to take medication for a medical condition. In caring for both the mother and her nursing infant, there are two important objectives: (a) protect the nursing infant from adverse effects from maternal medication and (b) permit the mother to receive necessary pharmacotherapy while preserving her ability to breast-feed her infant. A brief review of breast function and milk synthesis will help elucidate the possible mechanisms involved in the excretion of drugs into breast milk.
It must be emphasized that virtually all experimental work on breast function/milk secretion has been done in animal species. The difficulties and limitations of studying human lactation (anatomically and physiologically) using methods of continuous milk collection, serial biopsy of lactation tissue, and the administration of isotopic biochemical precursors are obvious. There are also known biochemical differences among the milk of different species: protein, fat, and carbohydrate content seem related to growth requirements (especially the rate of growth) of the infant of the particular species. Some of these differences will obviously dictate differences in drug transfer from maternal plasma to the milk. The pH of human milk, for example, is usually 7.0 or above; in the cow (where lactation has been most extensively studied), the pH is usually 6.8 or below. As seen later, this will have significant effect on the transport of some drugs depending on their pKa. However, there is no reason to suppose that the formation of human milk is qualitatively different from that in other animal species.
The lactating breast resembles a bunch of grapes, with each grape being tear-shaped and consisting of a cluster of alveolar cells in which breast milk is synthesized and secreted into a central lumen. The lumens feed into small ducts that meet each other in channels of increasing size until the nipple region is reached. With the possible exception of water transport, little alteration in the composition of milk occurs once it leaves the alveolar lumen. Excretion of drugs and chemicals most likely occurs only within this lumen.
Human milk is a suspension of fat and protein in a carbohydrate (lactose) and mineral solution. A nursing mother can easily produce 600 mL of milk per day (the amount needed for the complete nourishment of a 4-kg infant) containing 6.0 g of protein, 22.2 g of fat, and 42 g of lactose with the correct amount of minerals and most vitamins. Human milk also contains immunoglobulins, macrophages, lymphocytes, transferrin, lactoferrin, interferon, complement, fibronectin, erythropoietin, and many other unusual biologic compounds, all of which are designed to protect the infant and accelerate maturation of many physiologic processes (7). The exact composition of milk varies with duration of lactation, and may even vary within the time of a single feeding. Using a noninvasive computerized breast measurement system, it was demonstrated that infants were self-regulating their milk intake,
and that the rate of milk synthesis was at a maximum when the breast was empty and a minimum when the breast was full (8,9,10).
and that the rate of milk synthesis was at a maximum when the breast was empty and a minimum when the breast was full (8,9,10).
Milk protein is synthesized within the mammary gland. A small amount of maternal plasma protein does enter into breast milk, presumably across the alveolar cell and/or through the extracellular space between these cells. This explains the presence of cow milk protein (β-lactoglobulin from maternal cow milk consumption) in the serum of exclusively breast-fed infants. The major human milk proteins are casein and lactalbumin (the latter is also needed for lactose synthesis). Synthesis is initiated by prolactin and needs insulin and hydrocortisone to continue. The proteins are very digestible and contribute to the low curd tension of human milk. Proteins are transported from the endoplasmic reticulum into the Golgi apparatus, migrate from the base of the cell toward the apex, and are discharged into the alveolar lumen by apocrine secretion. The role of these proteins in binding drugs has yet to be investigated completely. In the two reported studies that specifically measured drug (theobromine and theophylline) binding to human milk protein, it was found to vary between 0% and 24% (11,12).
Short-chain fatty acids are synthesized in the mammary gland from acetate. Long-chain fatty acids are supplied by transfer from plasma. Both classes of fat are esterified in the breast with glycerol (from intracellular glucose). The lipids collect in the endoplasmic reticulum. As they ascend the cell in ever-increasing sizes of droplets, they acquire a three-layered membrane (lipoprotein) and are extruded into milk as milk fat globules (13). It is intriguing to speculate on the possibilities of drug binding to both the protein and fat components of the milk fat globule. It is also possible that some lipid-soluble drugs may be trapped entirely within the milk fat globule.
Lactose is entirely synthesized in the breast alveolar cell. Its synthesis proceeds from UDP-galactose and glucose, with lactose synthetase (galactose transferase plus lactalbumin) as the enzyme. Prolactin is absolutely required. Lactose is excreted from the cell into the alveolar lumen alone and with milk protein. Electrolytes, vitamins, and water are supplied by the cell (from plasma water) to achieve the final concentration.
All the preceding elements achieve a concentration in human milk that provides the ideal nutrient supply to the infant for at least the first 6 months. After 6 months of age, the infant’s caloric need usually requires supplemental food.
The transport of drugs into breast milk from maternal tissues and plasma may proceed by a number of routes. Figure 17.1 is a schematic drawing of the alveolar breast cell; it illustrates the cellular structures a substance in maternal plasma must traverse to enter milk contained within the alveolar lumen. After crossing the capillary endothelium, the drug traverses the interstitial space and must cross the basement membrane (consisting mostly of mucopolysaccharides of the alveolar breast cell). The cell plasma membrane is trilaminar with the usual phospholipid–protein membrane structure. After reaching the cell cytoplasm, the compound travels apically and leaves the cell by diffusion, reverse pinocytosis, or apocrine secretion (apical part of cell disintegrates). This entire process is nicely detailed by Vorherr (14). Excellent scanning electron micrographs of this process in the human breast are presented in the paper by Ferguson and Anderson (15).
Transcellular diffusion. Small un-ionized molecules with lipid solubility, such as urea and ethanol, transverse the capillary epithelium, intercellular water, basal cell membrane, alveolar cell, and its apical membrane by diffusion. This mechanism is supported by the observations that milk concentrations of such compounds mirror simultaneous maternal plasma levels (milk/plasma [M/P] ratio = 1) and that the elimination rate constants (as calculated from the elimination phases) are very similar (18).
Intercellular diffusion. This route avoids the breast alveolar cell entirely. Histologic studies in some animal species suggest that such a space may not exist or is very tight. It may be important functionally in the human and may explain how large molecules such as interferon, immunoglobulins, and cow milk protein enter human milk.
The electron microscopic studies in humans suggest that these junctions exist (15).
The electron microscopic studies in humans suggest that these junctions exist (15).
Passive diffusion. Small ionized molecules and small proteins may enter the basal part of the cell from interstitial water through passive diffusion in water-filled channels.
Ionophore diffusion. Polar substances may penetrate by being bound to carrier proteins within the cell membranes.
Diffusion appears to be the most common mechanism for a drug to cross a cell membrane. The distribution of a drug across a lipid biologic membrane has been shown to depend on the degree of ionization of the drug plus the assumption that a pH difference exists across the membrane; it is the un-ionized fraction that diffuses through the membrane (16,17,18,19). Knowing the degree of ionization of the drug (pKa) plus the pH difference across the mammary alveolar cell membrane, one can calculate the theoretical M/P ratio. [A complete discussion of the distribution of ionized drugs between plasma and milk is given by Rasmussen (20). Acknowledgment must be made of the elegant experiments by Rasmussen in defining this process in the animal models of the lactating cow and goat. Equally, acknowledgment must be made of Wilson et al. (21,22) for pointing out the limitations of using the M/P ratio to predict quantitation excretion of drugs into milk.] Figure 17.2 illustrates the situation for two weakly acidic drugs with markedly different pKa values: salicylic acid (pKa = 3.0) and phenobarbital (pKa = 7.2). Plasma pH is assumed to be 7.4, and milk pH is assumed to be 7.0 (commonly accepted for human milk). For a weak acid, the Henderson–Hasselbach equation defines the ratio of un-ionized (U) to ionized (I) drug at equilibrium as
log(U/I) = pKa – pH
and for a weak base as
log(I/U) = pKa – pH
![]() Figure 17.2. Theoretical distribution of weakly acidic drugs across a lipid cell membrane. Units are arbitrary for illustration. M/P, Milk/plasma ratio. |
The theoretical M/P ratio for total salicylate (un-ionized plus ionized) is 0.40; the experimental value obtained by Miller et al. (23) is 0.35. The theoretical ratio for total phenobarbital is 0.46; the experimental value is 0.7 (20,24). This difference for phenobarbital may be explained by the significant lipid solubility of this drug (chloroform/water distribution of un-ionized drug = 4.8). Thus, the presence on one side of a biologic membrane of a substance rich in fat (milk) will introduce another factor in the final determination of the concentration of a drug in breast milk.
Milk also has 1.0 g per 100 mL of proteins, any one of which also may bind drugs. Few data are available, but Rasmussen (25) demonstrated sulfonamide binding in milk to be present from 0% to 40% depending on the sulfonamide. As a general statement, weak acids (e.g., sulfanilamide, phenobarbital, salicylate, and penicillin) have an M/P ratio (both theoretical and experimental) of less than 1.0. Weak bases (e.g., antipyrine, lincomycin, quinine, ephedrine) have an M/P ratio greater than 1.0 (20,21). The precise experimental value will depend on factors such as maternal dosing intervals, milk pH, protein binding in plasma and milk, and lipid solubility of the drug (21,24,25,26,27).
The ratio of drug in the ultrafiltrate of milk to that in the ultrafiltrate of plasma was found to be independent of plasma levels (M/P ratio identical at all levels of plasma concentration). The concentration in milk was also found to be constant regardless of the volume of milk in the mammary gland. These observations support the thesis that diffusion is the major mechanism for the appearance of drugs in breast milk (20).
A number of reviews discuss the excretion of drugs in milk, and some provide tables of the concentration of drugs in breast milk (26,28,29,30,31). Many of the studies cited in these reviews are single case reports or small series of mother–child pairs. Many of the values consist of a single measurement of the drug concentration in milk; the period of time from maternal ingestion is frequently not defined, nor is maternal dose, frequency of dose, frequency of nursing, or duration of lactation. Measurement of the drug in the nursing infant’s blood or urine is frequently not mentioned. Hence, it is usually not possible to determine the risk to the nursling at a single isolated point. Many of the quoted references refer to data from the 1940s and the 1950s, when analytical methods were primitive. The oft-quoted reference to salicylates, for example, dates from 1935 and employed a semiquantitative method (FeCl3) for salicylate analysis; no quantitative values are given (32). The discipline of pharmacokinetics was not developed when many of the drugs in breast milk were initially assayed. Hence, few detailed studies employing drug measurements over a period of time in both maternal and infant body fluids are available. The availability of newer techniques (high-pressure liquid chromatography, mass spectroscopy) coupled with increased interest will result in more comprehensive studies.
Table 17.1 Excretion of Drugs in Human Milk | ||||||||||||||||||||||||||||||||||||||||||||||||||||||||||||||||||||||||||||||||||||||||||||||||||||||||||||||||||||||||||||||||||||||||||||||||||||||||||||||||||||||||||||||||||||||||||||||||||||||||||||||||||||||||||||||||||||||||
---|---|---|---|---|---|---|---|---|---|---|---|---|---|---|---|---|---|---|---|---|---|---|---|---|---|---|---|---|---|---|---|---|---|---|---|---|---|---|---|---|---|---|---|---|---|---|---|---|---|---|---|---|---|---|---|---|---|---|---|---|---|---|---|---|---|---|---|---|---|---|---|---|---|---|---|---|---|---|---|---|---|---|---|---|---|---|---|---|---|---|---|---|---|---|---|---|---|---|---|---|---|---|---|---|---|---|---|---|---|---|---|---|---|---|---|---|---|---|---|---|---|---|---|---|---|---|---|---|---|---|---|---|---|---|---|---|---|---|---|---|---|---|---|---|---|---|---|---|---|---|---|---|---|---|---|---|---|---|---|---|---|---|---|---|---|---|---|---|---|---|---|---|---|---|---|---|---|---|---|---|---|---|---|---|---|---|---|---|---|---|---|---|---|---|---|---|---|---|---|---|---|---|---|---|---|---|---|---|---|---|---|---|---|---|---|---|---|---|---|---|---|---|---|---|---|---|---|---|---|---|---|---|
|
In spite of the widespread use of drugs by the lactating women, there are only a very small number of reports of adverse reactions in breast-fed infants. Anderson et al. (33) surveyed the literature in Medline from 1966 to 2002. They identified 94 reports involving 100 infants of adverse reactions in nursing infants. Using the Naranjo method (34) they classified 53 of the reports as “possibly” being related to the drugs use by the mother and 47 of the reports as “probable.” No report met the criteria for “definite.” The age of the infants is of interest: 37% of the case reports occurred in infants younger than 2 weeks of age; 78% of reports were for infants 2 months of age or younger. Only 4% of the reports were for infants older than 6 months of age. Because of the immaturity of the hepatic and renal systems of these very young infant, they would be the most vulnerable to even the small concentrations of drugs excreted in milk. The very young infant, especially neonates, appears to be potentially most vulnerable.
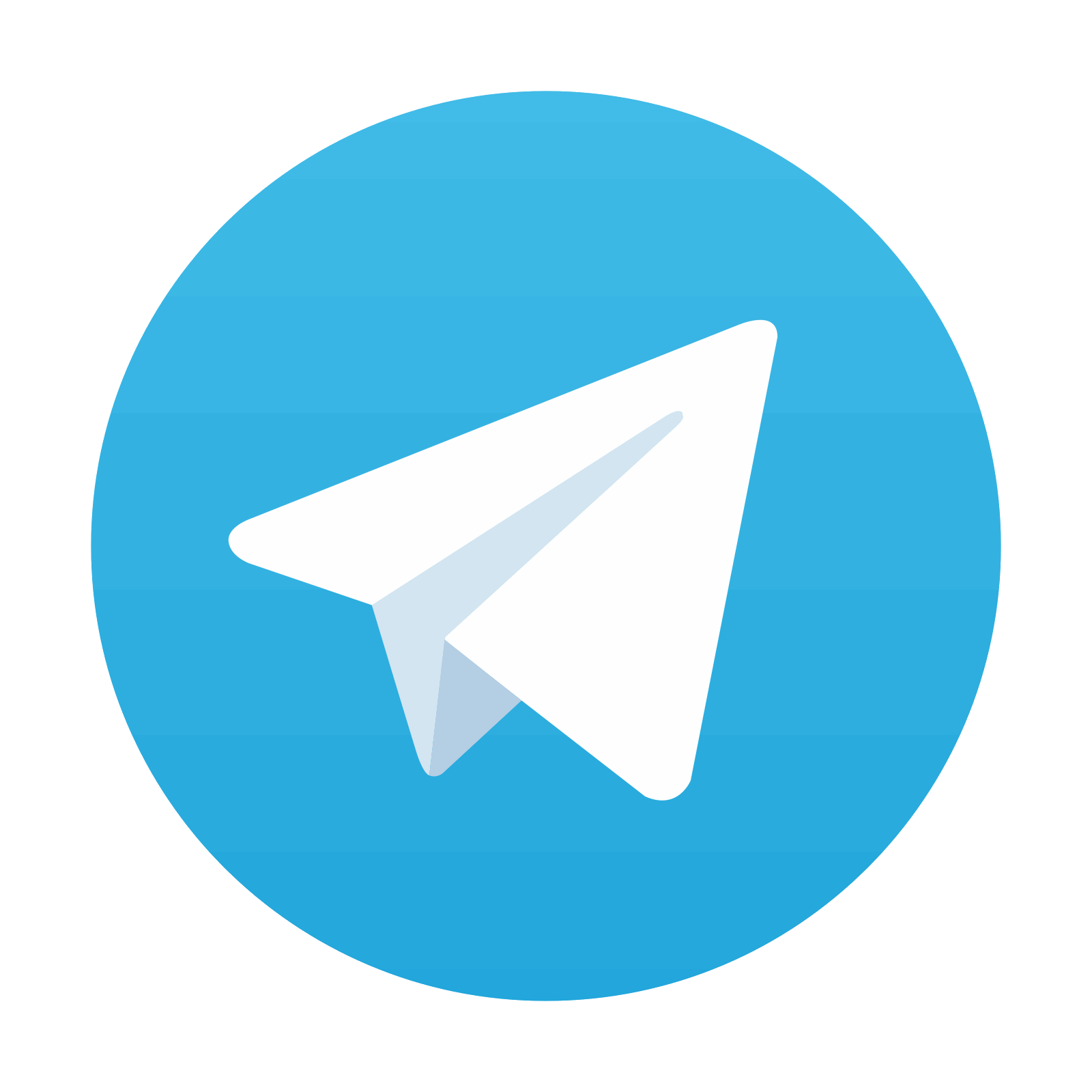
Stay updated, free articles. Join our Telegram channel

Full access? Get Clinical Tree
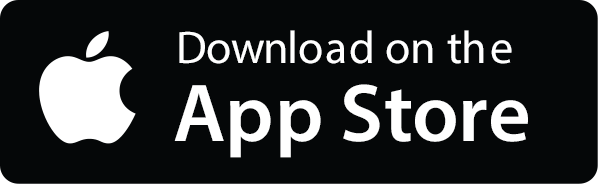
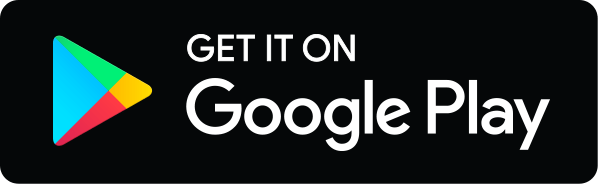