The Effects of Maternal Drugs on the Developing Fetus
David A. Beckman
Lynda B. Fawcett
Robert L. Brent
Every conception has a risk of ending in abortion or serious congenital anomaly (Tables 15-1,15-2). Furthermore it is axiomatic that every drug administered or taken by a pregnant woman presents the mother and fetus with both risks and benefits. The controversies in this field are primarily related to the nature and magnitude of the risks for these drugs.
Abortion and birth defects have some common etiologies, but in many instances the causes of these two areas of adverse reproductive outcome are divergent. Most human teratogens affect the embryo during a relatively narrow period of early embryonic development (18-40 days for major malformations excluding genital malformations and cleft palate which have longer periods of sensitivity). However, there are a few teratogens and many fetotoxic agents that have deleterious effects during the second and even the third trimester.
In this chapter, we evaluate the data concerning the potential risks of selected prescribed and self-administered drugs in human pregnancy. The evaluations were made after a review of the available clinical, epidemiological and experimental data and an analysis based on reproducibility, consistency and biological plausibility. Only key references or reviews are cited which will guide the reader to additional relevant literature.
CHARACTERIZATION OF ADVERSE REPRODUCTIVE OUTCOMES
Spontaneous Abortion
The definition of spontaneous abortion is based on the stage of embryonic development when viability was not possible outside the uterus. This stage is presently considered to be 20 weeks or less of gestation and a fetal weight of less than 500 grams, although these criteria are not universally accepted.
The frequency of spontaneous abortion varies with the stage of gestation Tables 15-2: more than 80% of abortions occur in the first trimester and there is a steady decline in the risk of abortion as pregnancy progresses. Therefore it is essential that epidemiological studies into the cause of abortion compare control and “exposed” populations with the same mean stage and range of abortion. Two pregnant populations with a 2-week difference in mean stage of pregnancy will have a different background incidence of abortion. Abortion in human populations include the following causes Tables 15-3:
Chromosomal Abnormalities
The earlier the abortion, the higher the proportion of chromosomal abnormalities (1,2). Approximately 53% of spontaneous abortions in the first trimester are as a result of chromosomal abnormalities, 36% are as a result of chromosomal abnormalities in the second trimester and only 5% of stillbirths in the third trimester are as a result of chromosomal abnormalities. Over 95% of abortuses with chromosomal abnormalities were as a result of autosomal trisomy, double trisomy, monosomy, triploidy or tetraploidy (3,4). Most chromosomal abnormalities are not the cause of repetitive abortion, although in about 4% of couples with two or more spontaneous abortions, a normal-appearing parent could be a carrier for a balanced translocation or may be a mosaic with abnormal cells in the germ cell line. Environmental exposures during pregnancy cannot account for any of these abortions because most aneuploidies result from meiotic nondisjunction during gametogenesis before conception.
TABLE 15-1 FREQUENCY OF REPRODUCTIVE RISKS IN THE HUMAN | ||||||||||||||||||||||||||||
---|---|---|---|---|---|---|---|---|---|---|---|---|---|---|---|---|---|---|---|---|---|---|---|---|---|---|---|---|
|
Abortions With Normal Chromosomes (Euploidy)
Hertig (1) and many other investigators reported the occurrence of malformed or blighted embryos as a cause of abortion. These embryonic losses may occur later in the first trimester and have been shown to have normal karyotypes (3). The etiology of these abortions are manifold and include the following:
TABLE 15-2 ESTIMATED OUTCOME OF 100 PREGNANCIES VERSUS TIME FROM CONCEPTION | ||||||||||||||||||||||||||||||||||||||||||
---|---|---|---|---|---|---|---|---|---|---|---|---|---|---|---|---|---|---|---|---|---|---|---|---|---|---|---|---|---|---|---|---|---|---|---|---|---|---|---|---|---|---|
|
Genetic Abnormalities
Dominant mutations (lethals), polygenic genetic abnormalities, and recessive disease may rarely account for repetitive abortion but in most instances they will occur sporadically. A review of gene knockouts and mutations in mice suggests that embryonic death resulted from disrupting basic cellular functions, vascular circulation, hematopoiesis or nutritional supply from the mother rather than affecting embryonic organ systems (5).
Maternal Diabetes
Type I (insulin dependent) diabetes mellitus with poor metabolic control increases the risk of abortion and still births but there is no increased risk with good metabolic control.
Maternal Hyper- and Hypothyroidism
Abnormal thyroid function is rare in patients with recurrent abortion.
Corpus Luteum or Placental Progesterone Deficiency (Luteal Phase Deficiency)
It is controversial whether low hormone levels after implantation result from impending abortion or are the cause of the abortion.
Maternal Infection
Infections of the genital tract could be responsible for abortion but it is not easy to document causality. The data suggesting that infection with Chlamydia trachomatis, Borrelia burgdorferi, Mycoplasma hominis, Listeria monocytogenes, and Ureaplasma urealyticum result in abortion are not conclusive. In contrast, maternal disease resulting in fetal infection with Treponema pallidum, Plasmodium falciparum, Toxoplasma gondii, herpes simplex virus, parvovirus B19, or cytomegalovirus has the potential to cause stillbirth or spontaneous abortion.
TABLE 15-3 ETIOLOGY OF SPONTANEOUS ABORTION IN THE HUMAN | |
---|---|
|
Severe, Debilitating Maternal Diseases
Hepatitis, collagen diseases, untreated hyperthyroidism, Wilson’s disease or severe malnutrition can lead to abortion.
Antiphospholipid Antibodies
Lupus anticoagulant and anticardiolipin antibodies predispose women to recurrent abortion in both first and second trimesters as a result of vascular disruption in the placenta.
Maternal-Fetal Histocompatibility
It is suggested that embryonic loss increases if the mother and fetus are more histocompatible at the human leukocyte antigen (HLA) locus, resulting in the failure to develop maternal blocking antibodies against paternal antigens.
Overmature Gametes
Either the ovum or sperm could age because insemination occurred a few days prior to ovulation or ovulation occurred prior to insemination. The magnitude of this risk factor as a cause of spontaneous abortion is not known and some investigators are skeptical that this phenomenon is clinically significant.
Mechanical or Physical Problems Related to Uterine Abnormalities, Multiple Pregnancies or Trauma
A hostile intrauterine environment can result from submucosal or intramural myomas, adhesions (Asherman’s syndrome), multiple embryos or abnormalities of the uterus (bifid uterus, infantile uterus). Uterine trauma from a direct blow or penetrating injury may rarely be responsible for an abortion and if this type of injury occurs it would be more likely to result in a still birth at midgestation or later.
Cervical Incompetence
Cervical incompetence is more likely to result in second trimester than first trimester abortions.
Abnormal Placentation
Hypoplastic trophoblast and circumvallate implantation increase the risk of fetal loss.
Some Environmental Teratogens and Reproductive Toxins
Severe malformations or growth retardation implantation increase the risk of fetal loss.
Congenital Malformations
The etiology of congenital malformations can be divided into three categories: unknown, genetic, and environmental Tables 15-4. The etiology of 65%-75% of human malformations is unknown. A significant proportion of congenital malformations of unknown etiology is likely to have an important genetic component. Malformations with an increased recurrence risk, such as cleft lip and palate, anencephaly, spina bifida, certain congenital heart diseases, pyloric stenosis, hypospadias, inguinal hernia, talipes equinovarus, and congenital dislocation of the hip, fit in the category of multifactorial disease and in the category of polygenic inherited disease (6). The multifactorial/threshold hypothesis postulates the modulation of a continuum of genetic characteristics by intrinsic and extrinsic (environmental) factors (6). Although the modulating factors are not known, they probably include: placental blood flow, placental transport, site of implantation, maternal disease states, maternal malnutrition, infections, drugs, chemicals, and spontaneous errors of development.
Spontaneous errors of development may account for some of the malformations that occur without apparent abnormalities of the genome or environmental influence. We postulate that there is some probability for error during embryonic development based on the fact that embryonic development is such a complicated process. It is estimated that 75% of all conceptions are lost before term; 50% within the first 3 weeks of development (1,2). The World Health Organization estimated that 15% of all clinically recognizable pregnancies end in a spontaneous abortion, 50%-60% of which are as a result of chromosomal abnormalities (4,7,8). Finally, 3%-6% of offspring are malformed, which represents the background risk for human maldevelopment. This means that, as a conservative estimate, 1,176 clinically recognized pregnancies will result in approximately 176 miscarriages and 30 to 60 of the infants will have congenital anomalies in the remaining 1,000 live births. The true incidence of pregnancy loss is much higher because undocumented pregnancies are not included in this risk estimate.
TABLE 15-4 ETIOLOGY OF HUMAN CONGENITAL MALFORMATIONS OBSERVED DURING THE FIRST YEAR OF LIFE | ||||||||||||||||||
---|---|---|---|---|---|---|---|---|---|---|---|---|---|---|---|---|---|---|
|
Based on his review of the literature, Wilson (9) provided a format of theoretical teratogenic mechanisms: mutation; chromosomal aberrations; mitotic interference; altered nucleic acid synthesis and function; lack of precursors, substrates, or coenzymes for biosynthesis; altered energy sources; enzyme inhibition; osmolar imbalance, alterations in fluid pressures, viscosities, and osmotic pressures; and altered membrane characteristics. We suggest a revised list of mechanisms for teratogenesis Tables 15-5.
Even though an agent can produce one or more of these pathological processes, exposure to such an agent does not guarantee that maldevelopment will occur. Furthermore, it is likely that a drug, chemical or other agent can have more than one effect on the pregnant woman and the developing conceptus and therefore the nature of the drug or its biochemical or pharmacological effects will not in themselves predict a teratogenic effect in the human. In fact, the discovery of human teratogens has come primarily from human epidemiological studies. Animal studies and in vitro studies can be very helpful in determining the mechanism of teratogenesis and the pharmacokinetics related to teratogenesis (10). However, even if one understands the pathological effects of an agent, one cannot predict the teratogenic risk of an exposure without taking into consideration the developmental stage, the magnitude of the exposure and the reparability of the embryo.
TABLE 15-5 MECHANISMS OF TERATOGENESIS | |
---|---|
|
Various maternal viral, bacterial, and parasitic infections are known to cause maldevelopment in humans including cytomegalovirus, fetal herpes virus infections (type 1 or 2), parvovirus B19 (erythema infectiosum), rubella virus, congenital syphilis (Treponema pallidum), T. gondii infection, varicella-zoster virus, and venezuelan equine encephalitis (11). The incidence of serum antibody to human immunodeficiency virus (HIV) in pregnant women is increasing from the 1991 estimate of 1.5 per 1,000 women delivering in the United States (12); the incidence is as high as 31% in pregnant women in some African cities (13). Several studies support the conclusion that asymptomatic HIV pregnancies are not associated with an increased risk of congenital malformations, low birth weight or abortion (14,15,16,17). It is likely that sexually transmitted diseases, opportunistic maternal infections, and symptomatic HIV pregnancies may increase the risk of low birth weight and morbidity in noninfected offspring.
The lethal or developmental effects of infectious agents are the result of mitotic inhibition, direct cytotoxicity or necrosis. Repair processes may result in metaplasia, scarring or calcification, which causes further damage by interfering with histogenesis. Infectious agents appear to be exceptions to some of the principles of teratogenesis because the relevance of dose and time of exposure cannot be demonstrated as readily for replicating teratogenic agents. Transplacental transmission of an infectious agent does not necessarily result in congenital malformations, growth retardation, or lethality.
Vascular disruption is a rare event associated with intrauterine death and a wide range of structural anomalies, including cerebral infarctions, certain types of visceral and urinary tract malformations, congenital limb amputations of the nonsymmetrical type; and orofacial malformations such as mandibular hypoplasia, cleft palate, and Moebius syndrome, which vary too widely to constitute a recognized syndrome. Some anomalies associated with twin pregnancies can be explained by vascular disruption resulting from placental anastomoses in shared placenta of monozygotic twins, anastomoses in a small percentage of dichorionic placentas in the case of dizygotic twins, or death of one twin resulting in emboli, intravascular coagulation and altered fetal hemodynamics in the co-twin (18,19). Vascular disruption may also result from physical trauma causing chorion bleeding, such as chorionic villous sampling, and exposure to some developmental toxicants, such as cocaine and misoprostol. Although uterine bleeding during the first trimester may result in fetal anomalies, the malformations associated with vascular disruption can also occur later in gestation. This topic is discussed in greater detail below with specific drugs.
Adverse Effects Produced Later in Pregnancy
The fetal period is characterized by histogenesis involving cell growth, differentiation, and migration. Drugs that produce permanent cell depletion, vascular disruption, necrosis, specific tissue or organ pathology, physiological decompensation, or severe growth retardation have the potential to cause deleterious effects throughout gestation. Sensitivity of the fetus for induction of mental retardation and microcephaly is greatest at the end of the first and the beginning of the second trimester. Other permanent neurological effects can be induced in the second and third trimesters.
The classic example of a drug that presents little risk to the developing embryo during organogenesis but can affect the near-term fetus if high doses are used is aspirin. It is possible that other antiinflammatory drugs present a similar risk.
FACTORS THAT AFFECT SUSCEPTIBILITY TO THE DELETERIOUS EFFECTS OF DRUGS
A basic tenet of environmentally produced embryo- and fetotoxicity is the effects of teratogenic or abortigenic milieu which have certain characteristics in common and follow certain basic principles. These principles determine the quantitative and qualitative aspects of developmental toxicity Tables 15-6.
Stage of Development
The induction of developmental toxicity by environmental agents usually results in a spectrum of morphological anomalies or intrauterine death, which varies in incidence depending on stage of exposure and dose. The developmental period at which an exposure occurs will determine which structures are most susceptible to the deleterious effects of the drug or chemical and to what extent the embryo can repair the damage. The period of sensitivity may be narrow or broad, depending on the environmental agent and the malformation in question. Limb defects, produced by thalidomide, have a very short period of susceptibility Tables 15-7 although microcephaly produced by radiation has a long period of susceptibility. Our knowledge of the susceptible stage of the embryo to various environmental influences is continually expanding and is vital to evaluating the significance of individual exposures or epidemiological studies.
TABLE 15-6 FACTORS THAT INFLUENCE SUSCEPTIBILITY TO DEVELOPMENTAL TOXICANTS | |
---|---|
|
During the first period of embryonic development, from fertilization through the early postimplantation stage, the embryo is most sensitive to the embryolethal effects of drugs and chemicals. Surviving embryos have malformation rates similar to the controls not because malformations cannot be produced at this stage but because significant cell loss or chromosome abnormalities at theses stages have a high likelihood of killing the embryo. Because of the omnipotentiality of early embryonic cells, surviving embryos have a much greater ability to have normal developmental potential. Wilson and Brent (20) demonstrated that the all-or-none phenomenon, or marked resistance to teratogens, disappears over a period of a few hours in the rat during early organogenesis utilizing ionizing X-irradiation as the experimental teratogen. The term all-or-none phenomenon has been misinterpreted by some investigators to indicate that malformations cannot be produced at this stage. On the contrary, it is likely that certain drugs, chemicals, or other insults during this stage of development can result in malformed offspring, but the nature of embryonic development at this stage will still reflect the basic characteristic of the all-or-none phenomenon which is a propensity for embryo lethality rather than surviving malformed embryos.
TABLE 15-7 DEVELOPMENTAL STAGE SENSITIVITY TO THALIDOMIDE-INDUCED LIMB REDUCTION DEFECTS IN THE HUMAN | ||||||||||||||||||||||
---|---|---|---|---|---|---|---|---|---|---|---|---|---|---|---|---|---|---|---|---|---|---|
|
The period of organogenesis (from day 18 through about day 40 of postconception in the human), is the period of greatest sensitivity to teratogenic insults and the period when most gross anatomic malformations can be induced. Most environmentally produced major malformations occur before the 36th day of gestation in the human. The exceptions are malformations of the genito-urinary system, the palate, the brain or deformations as a result of problems of constraint, disruption or destruction. Severe growth retardation in the whole embryo or fetus may also result in permanent deleterious effects in many organs or tissues.
The fetal period is characterized by histogenesis involving cell growth, differentiation, and migration. Teratogenic agents that produce permanent cell depletion, vascular disruption, necrosis, specific tissue or organ pathology, physiological decompensation, and/or severe growth retardation have the potential to cause deleterious effects throughout gestation. Additionally, sensitivity of the fetus for induction of mental retardation and microcephaly is greatest at the end of the first and the beginning of the second trimester. Other permanent neurological effects can be induced in the second and third trimesters. Effects such as cell depletion or functional abnormalities, not readily apparent at birth, may give rise to changes in behavior or fertility which are apparent only later in life. The approximate last gestational day on which certain malformations may be induced in the human is presented in Table 15-2.
Magnitude of the Exposure
The dose-response relationship is extremely important when comparing effects among different species because usage of mg/kg doses are, at most, rough approximations. Dose equivalence among species can only be accomplished by performing pharmacokinetic studies, metabolic studies, and dose-response investigations in the human and the species being studied. Furthermore, the response should be interpreted in a biologically sound manner. One example is that a substance given in large enough amounts to cause maternal toxicity is likely to also have deleterious effects on the embryo such as death, growth retardation, or retarded development. Another example is that because the steroid receptors that are necessary for naturally occurring and synthetic progestin action are absent from nonreproductive tissues early in development, the evidence is against the involvement of progesterone or its synthetic analogues in nongenital teratogenesis (21,22).
An especially anxiety-provoking concept is that the interaction of two or more drugs or chemicals may potentiate their developmental effects. Although this is an extremely difficult hypothesis to test in the human, it is an especially important consideration because multichemical or multitherapeutic exposures are common. Fraser (23) warns that the actual existence of a threshold phenomenon when nonteratogenic doses of two teratogens are combined could easily be misinterpreted as potentiation or synergism. Potentiation or synergism should be invoked only when exposure to two or more drugs is just below their thresholds for toxicity.
Several considerations affect the interpretation of dose-response relationships:
Active metabolites: Metabolites may be the proximate teratogen rather than the administered chemical, i.e., the metabolites phosphoramide mustard and acrolein may produce maldevelopment resulting from exposure to cyclophosphamide (24).
Duration of exposure: A chronic exposure to a prescribed drug can contribute to an increased teratogenic risk, e.g., anticonvulsant therapy; in contrast an acute exposure to the same drug may present little or no teratogenic risk.
Fat-solubility: Fat-soluble substances such as polychlorinated biphenyls (25) can produce fetal maldevelopment for an extended period after the last ingestion or exposure in a woman because they have an unusually long half life.
Threshold Phenomena
The threshold dose is the dosage below which the incidence of death, malformation, growth retardation, or functional deficit is not statistically greater than that of controls. The threshold level of exposure is usually from less than one to three orders of magnitude below the teratogenic or embryopathic dose for drugs and chemicals that kill or malform half the embryos. A teratogenic agent therefore has a no-effect dose as compared to mutagens or carcinogens, which have a stochastic dose response curve. Threshold phenomena are compared to stochastic phenomena in Table 15-8. The severity and incidence of malformations produced by every exogenous teratogenic agent that has been appropriately tested have exhibited threshold phenomena during organogenesis (9).
TABLE 15-8 STOCHASTIC AND THRESHOLD DOSE-RESPONSE RELATIONSHIPS OF DISEASES PRODUCED BY ENVIRONMENTAL AGENTS | ||||||||||||||||||||||||
---|---|---|---|---|---|---|---|---|---|---|---|---|---|---|---|---|---|---|---|---|---|---|---|---|
|
Pharmacokinetics and Metabolism
The physiological alterations in pregnancy and the bioconversion of compounds can significantly influence the teratogenic effects of drugs and chemicals by affecting absorption, body distribution, active form(s), and excretion of the compound. Physiological alterations in the mother during pregnancy which affect the pharmacokinetics of drugs include the following: decreased gastrointestinal motility and increased intestinal transit time may delay absorption of drugs absorbed in the small intestine as a result of increased stomach retention but enhance absorption of slowly absorbed drugs; decreased plasma albumin concentration, which alters the kinetics of compound normally bound to albumin; increased plasma and extracellular fluid volumes that affect concentration-dependent transfer of compounds; renal elimination, which is generally increased but is influenced by body position during late pregnancy; inhibition of metabolic inactivation in the liver late in pregnancy; and variations in uterine blood flow, although little is known about how this affects transfer across the placenta (26,27,28).
The fetus also undergoes physiological alterations, which affect the pharmacokinetics of drugs (28): the amount and distribution of fat varies with development and affects the distribution of lipid-soluble drugs; the fetal circulation contains a higher concentration of unbound drug largely because the plasma fetal proteins are lower in concentration than in the adult and may lower drug affinity; the functional development of pharmacological receptors is likely to proceed at different rates in the various tissues; and i.v. drugs excreted by the fetal kidneys may be recycled by swallowing of amniotic fluid.
The role the placenta plays in drug pharmacokinetics, reviewed by Juchau and Rettie (29) and Miller (25), involves transport [discussed in detail in the section Placental Transport; the presence of receptors sites for a number of endogenous and xenobiotic compounds (β-adrenergic, glucocorticoid, epidermal growth factor, IgG-Fc, insulin, low-density lipoproteins, opiates, somatomedin, testosterone, transcobalamin II, transferrin, folate, retinoid)] (25); and the bioconversion of xenobiotics. Bioconversion of xenobiotics has been shown to be important in the teratogenic activity of several xenobiotics. There is strong evidence that reactive metabolites of cyclophosphamide, 2-acetylaminofluorene, and nitroheterocycles (niridazole) are the proximal teratogens (30). There is also experimental evidence that suggests that other chemicals undergo conversion to intermediates that have deleterious effects on embryonic development including phenytoin, procarbazine, rifampicin, diethylstilbestrol, some benzhydrylpiperazine antihistamines, adriamycin, testosterone, benzo(a)pyrene, methoxyethanol, caffeine, and paraquat (29,30).
The major site of bioconversion of chemicals in vivo is likely to be the maternal liver. Placental P450-dependent monooxygenation of xenobiotics will occur at low rates unless induced by such compounds as those found in tobacco smoke (29). However, the fetus also develops functional P450 oxidative isozymes capable of converting proteratogens to active metabolites.
Maternal Disease
Maternal disease states such as diabetes mellitus, epilepsy, phenylketonuria, and endocrinopathies are associated with adverse effects on the fetus. In some cases, it may be difficult to determine whether a maternal disease or the treatment for the disease plays a role in the etiology of malformations associated with the treatment for that disease during pregnancy. For example, the genetic and environmental milieu, which cause epilepsy may also contribute to the maldevelopment associated with exposure to diphenylhydantoin (31).
The role of maternal malnutrition is an important area for investigation because it may be a contributing factor to many teratogenic milieu. A series of investigations provided evidence suggesting that folic acid supplementation could reduce the incidence of recurrence of neural tube defects in the human (32,33,34,35). It was later shown convincingly that periconceptional supplementation with folic acid, 4 mg/day, reduces the risk of recurrence of neural tube defects in subsequent siblings of children with neural tube defects (36). Furthermore, low-dose folic acid supplementation, 0.8 mg/day, was reported to decrease the incidence of neural tube defects in a population not at increased risk for these defects (37). Although folate supplementation reduces the incidence of neural tube defects, folate supplementation will not prevent all neural tube defects and it is not known whether folic acid supplementation corrects an undefined metabolic defect or a nutritional deficiency.
Placental Transport
The exchange between the mammalian embryo and the maternal organism is controlled by the placenta which includes the chorioplacenta, the yolk sac placenta and the paraplacental chorion. The placenta varies in structure and function among species and for each stage of gestation. As an example, the rodent yolk sac placenta continues to function as an organ of transport for a much greater part of gestation than in the human. Thus differences in placental function and structure may affect our ability to apply teratogenic data developed in one species directly to other species, including the human (38). As pharmacokinetic techniques and the actual measurement of metabolic products in the embryo become more sophisticated, the appropriateness of utilizing animal data to project human effects may improve.
Historically a placental barrier was thought to exist, which prevented harmful substances from reaching the embryo. It is now clear that there is no “placental barrier” per se. The fact is that most drugs and chemicals cross the placenta. It will be a rare substance that will cross the placental barrier in one species and be unable to reach the fetus in another (39). No such chemical exists except for selected proteins whose actions are species-specific.
Even before there were chemical techniques to demonstrate the presence of drugs or chemicals in the embryo there was clear evidence that they had reached the fetus because of clinical manifestations of the drugs: anticoagulants such as warfarin can affect the clotting of fetal blood; many drugs can affect the fetal cardiac rate; changes in the fetal electroencephalogram (EEG) can be demonstrated as a result of the many drugs that affect the central nervous system (CNS); and newborns may exhibit withdrawal symptoms from drugs taken by their mothers, either medications or substances of abuse such as alcohol or opiates.
These observations demonstrate clinically significant placental transport of drugs only in the latter portion of gestation and may not be a means of evaluating embryonic exposure during early organogenesis.
Those factors which determine the ability of a drug or chemical to cross the placenta and reach the embryo include: molecular weight, lipid affinity or solubility, polarity or degree of ionization, protein binding, and receptor mediation. Compounds with low molecular weight, lipid affinity, nonpolarity, and without protein-binding properties will cross the placenta with ease and rapidity. As an example, ethyl alcohol is a chemical which reaches the embryo rapidly and in concentrations equal to or greater than the level in the mother.
High molecular weight compounds like heparin, 20,000 daltons, do not readily cross the placenta and therefore heparin is used to replace warfarin-like compounds during pregnancy for the treatment of hypercoagulation conditions. Rose Bengal does not cross the placenta. In general, compounds with molecular weights of 1,000 or greater do not readily cross the placenta, although 600 dalton compounds usually do; most drugs are 250-400 daltons and cross the placenta (40).
In addition to the particular properties of the drug or chemical, three other conditions affect the quantitative aspect of placental transport: (a) placental blood flow, (b) the pH gradient between the maternal and fetal serum and tissues, and (c) placental metabolism of the chemical or drug. The biotransformation properties of the placenta and/or maternal organism are important because a number of chemicals or drugs are not teratogenic in their original form.
The most important concept regarding placental transport of teratogens must be reemphasized. An agent is teratogenic because it affects the embryo directly or indirectly by its ability to produce a toxic effect in the embryo or extraembryonic membranes at exposures which are attained in the human being, not because it crosses the placenta per se.
Genotype
The genetic constitution of an organism is an important factor in the susceptibility of a species to a drug or chemical. More than 30 disorders of increased sensitivity to drug toxicity or effects have been reported in the human as a result of an inherited trait (41). The effect of a drug or chemical depends on both the maternal and fetal genotypes and may result in differences in cell sensitivity, placental transport, absorption, metabolism (activation, inactivation, active metabolites), receptor binding, and distribution of an agent. This accounts for some variations in teratogenic effects among species and in individual subjects.
Estimating the Developmental Risks of Drugs During Human Pregnancy
Evaluation of Data Available for the Human
Although chemicals and drugs can be evaluated for fetotoxic potential by utilizing in vivo animal studies and in vitro systems, it should be recognized that these testing procedures are only one component in the process of evaluating the potential teratogenic risk of drugs and chemicals in the human. The evaluation of the teratogenicity of drugs and chemicals should include, when possible, (a) data obtained from human epidemiological studies, (b) secular trend data in humans, (c) animal developmental toxicity studies, (d) the dose-response relationship for developmental toxicity and the relationship to the human pharmacokinetic equivalent dose in the animal studies, and (e) considerations of biological plausibility Tables 15-9 (42,43). This method is of greatest value when utilized for the evaluation of chemicals and drugs that have been in use for some time or for evaluating new drugs that have a similar mechanism of action, structure, pharmacology and purpose similar to other, extensively studied agents. The ability to establish a causal relationship between an environmental agent and abortigenic effect is more difficult, for the following reasons:
-
Abortion is a very frequent reproductive event and therefore the incidence can vary considerably between different populations of women. Differences in the abortion incidence between two populations in a single study may be as a result of chance alone.
-
There are multiple causes of abortion and most epidemiological studies dealing with abortion make no attempt to determine the etiology of the abortions. Since most abortions are as a result of preconceptual or periconceptual events, it is extremely difficult to match patients in case control studies and it would be necessary to have large increases in a particular etiological category of environmentally induced abortion to demonstrate a statistically significant increase in the incidence of spontaneous abortion in an “exposed” population of pregnant women.
-
Confounding factors appear to be more significant in abortion studies than in birth defect studies (cocaine, smoking, alcohol, syphilis, narcotics, caffeine). This further decreases the possibility that the agent being studied has a direct abortigenic effect.
TABLE 15-9 EVIDENCE FOR POTENTIAL DEVELOPMENTAL TOXICITY IN THE HUMAN | ||
---|---|---|
|
One of the advantages of reproductive effects is that there is frequently, but not always, concordance of effects involving more than one parameter (growth, malformations, abortion, stillbirth, prematurity, etc.). Isolated abortion studies that do not study the totality of reproductive effects are at a serious disadvantage, because spurious or nonetiological results may be misinterpreted as being causally related to a drug or environmental toxicant.
Some investigators and regulatory agencies divide drugs and chemicals into developmentally toxic and nontoxic compounds. In reality, potential developmental toxicity can be evaluated only if one considers, as a minimum, the agent, the dose, the species and the stage of gestation. Working definitions for developmental toxicity in the human are suggested in Table 15-10.
Potential human teratogens and abortifacients comprise a large group of drugs because they include all drugs and chemicals that can produce embryotoxic and fetotoxic effects at some exposure. Since these exposures are not utilized or attained in the human, they represent no or minimal risks to the human embryo.
Misconceptions in Evaluating Developmental Toxicity in the Human
Misconceptions have lead to confusion regarding the potential effects of even proven teratogens. Examples of erroneous concepts include: if an agent can produce one type of malformation, it can produce any malformation; an agent presents a risk at any dose, once it can be proven to be teratogenic; and an agent that is teratogenic is likely to be abortigenic.
TABLE 15-10 DEFINITIONS OF POTENTIAL FOR DEVELOPMENTAL TOXICITY IN THE HUMAN | ||
---|---|---|
|
These concepts are incorrect. The data clearly indicate that proven teratogens do not have the ability to produce every birth defect. Many teratogens can be identified on the basis of the malformations that are produced. Thus, the concept of the syndrome is probably more appropriate in clinical teratology than any other area of clinical medicine. Some symptoms or signs appear in many teratogenic syndromes, such as growth retardation or mental retardation, and therefore are not very discriminating. On the other hand, rare or specific effects, such as deafness, retinitis, or a pattern of cerebral calcifications, may point to a specific teratogen. It is also true that there is substantial overlap in malformation syndromes which may not always be separable. Environmentally produced birth defects may be confused with genetically determined malformations. Using thalidomide as an example, a patient with bilateral radial aplasia and a ventricular septal defect may have the Holt Oram syndrome or the thalidomide syndrome. It may or may not be possible to make a diagnosis with absolute certainty, even if one has a history of thalidomide ingestion during pregnancy. It is possible, however, to refute the suggestion that thalidomide was responsible for congenital malformations in an individual by the nature of the limb malformation.
The specificity of some teratogens can sometimes point to the mechanism or site of action. For instance, the predominant central nervous system effects of methyl mercury are understood when one realizes the propensity for organic mercury to be stored in lipid.
Epidemiologists sometimes use poor judgment when grouping malformations. As an example, limb reduction defects are frequently studied regarding their association with environmental teratogens but, in some studies, limb defects that are clearly related to problems of organogenesis are lumped with congenital amputations even though it is very unlikely that any agent will be responsible for both types of malformations. It is clear that epidemiological studies could be markedly improved if there was more input from clinical teratologists in planning and performing the studies.
Case control studies concerning spontaneous abortion may contain serious errors unless the populations being studied are similar regarding the stage of pregnancy when abortion occurred. This study design diminishes the possibility that the abortion rate will differ on the basis of the selection process and not the drug or environmental agent being studied. Unfortunately most epidemiological studies dealing with drug or environmentally induced abortion do not attempt to determine the etiology of the abortion.
Potential Embryo- and Fetotoxicity of Selected Prescribed and Self-administered Drugs
We evaluated the literature concerning selected drugs that cause or are suggested to cause deleterious effects during pregnancy in the human. The data included human epidemiological studies, secular trend data in humans where appropriate, and animal developmental toxicity studies. In our analysis we considered the dose-response relationship of teratogenicity, the relationship to the human pharmacokinetic equivalent dose in the animal studies and biological plausibility Tables 15-9 (42,43). Table 15-11 focuses on these drugs, listing their potential adverse effects in the human. Although these drugs account for a small percentage of all malformations and abortions, they are important because these exposures may be preventable.
Alcohol
Adverse effects in offspring from excessive alcohol consumption during pregnancy were recognized more than 200 years ago (46). It was Jones and associates (47) however, who defined the fetal alcohol syndrome (FAS) in children with intrauterine growth retardation, microcephaly, mental retardation, maxillary hypoplasia, flat philtrum, thin upper lip, and reduction in the width of palpebral fissures. Cardiac abnormalities were also seen. Many of the children of alcoholic mothers had FAS and all of the affected children evidenced developmental delay (47,48).
A period of greatest susceptibility is not clearly established but the risk for adverse effects increases with increased consumption and binge drinking early in pregnancy may be associated with an increased risk of alcohol-related effects (49). The risk of decreased brain growth and differentiation that results from high alcohol consumption is greater during the second and third trimester. Chronic consumption of 6 oz of alcohol per day constitutes a high risk although the FAS is not likely when the mother consumes fewer than two drinks (equivalent to 1 oz of alcohol) per day (50). Reduction of alcohol consumption or cessation of drinking early in pregnancy will reduce the incidence and severity of alcohol-related effects (49,51,52,53) but may not entirely eliminate the risk of some degree of physical or behavioral impairment. The human syndrome is likely to involve the direct effects of alcohol and the indirect effects of genetic susceptibility and poor nutrition. Alcoholism can have maternally deleterious effects on intermediary metabolism and nutrition, especially if alcoholic cirrhosis is present, which can contribute to an adverse milieu for the developing embryo.
Although alcoholic mothers frequently smoke and consume other drugs, there is little doubt from the human and animal data that alcohol ingestion alone can have a disastrous effect on the developing embryo or fetus. The reported incidence of FAS varies widely in different studies but appears to be approximately 6% in offspring of women who drink heavily during pregnancy (51). Fetal alcohol syndrome may be the most commonly recognized cause of environmentally induced mental deficiency; there are at least several hundred children born each year with full FAS and probably many more with subtler fetal alcohol effects (50,54).
TABLE 15-11 EFFECTS AND ESTIMATED RISKS OF SELECTED PRESCRIBED AND SELF-ADMINISTERED DRUGS HUMAN PREGNANCY | |||||||||||||||||||||||||||||||||||||||||||||||||||||||||||||||||||||||||||||||||||||||||||||||||||||||||||||||
---|---|---|---|---|---|---|---|---|---|---|---|---|---|---|---|---|---|---|---|---|---|---|---|---|---|---|---|---|---|---|---|---|---|---|---|---|---|---|---|---|---|---|---|---|---|---|---|---|---|---|---|---|---|---|---|---|---|---|---|---|---|---|---|---|---|---|---|---|---|---|---|---|---|---|---|---|---|---|---|---|---|---|---|---|---|---|---|---|---|---|---|---|---|---|---|---|---|---|---|---|---|---|---|---|---|---|---|---|---|---|---|
|
Aminopterin and Methotrexate
Aminopterin and methotrexate (methylaminopterin) are folic acid antagonists that inhibit dihydrofolate reductase, resulting in cell death during the S phase of the cell cycle (55). Aminopterin-induced therapeutic abortions have resulted in malformations (hydrocephalus, cleft palate, meningomyelocele and growth retardation) in some of the abortuses (56,57,58). Three case reports of children exposed to aminopterin in utero included observations of growth retardation, abnormal cranial ossification, high-arched palate, and reduction in derivatives of the first branchial arch (59). The pattern of malformations associated with exposure to either compound has been referred to as the fetal aminopterin/methotrexate syndrome (60). Key features of this pattern of malformations include prenatal growth deficiency, abnormal cranial ossification, micrognathia, small low set ears, and limb abnormalities. There have also been three case reports to date of severe developmental delay in children with methotrexate syndrome (61,62,63). Methotrexate is used therapeutically as an abortifacient, treatment of rheumatoid arthritis and other autoimmune disorders, and as an antineoplastic agent. Skalko and Gold demonstrated a threshold effect and a dose-dependent increase in malformations in mice exposed to methotrexate in utero (64). Although malformations were induced in rats at doses exceeding those used in humans (65), smaller doses than those used in humans have resulted in malformation in rabbits (66). Analysis of human data indicate a critical period of exposure to methotrexate from 6-8 weeks from conception at a dose above 10 mg per week for the development of aminopterin/ methotrexate syndrome (67). The risk of adverse effects as a result of aminopterin in the usual therapeutic range is not know precisely but appears to be moderate to high (58).
Androgens
Masculinization of the external genitalia of the female has been reported following in utero exposure to large doses of testosterone, methyltestosterone, and testosterone enanthate (68,69,70). The masculinization is characterized by clitoromegaly with or without fusion of the labia minora and no indication of nongenital malformations. Affected females experience normal secondary sexual development at puberty (71).
Many animal models show the masculinizing effects of androgens. Well-known studies were performed by Greene and coworkers in the rat, Raynaud in the mouse, Bruner and Witschi in the hamster, Jost in the rabbit, and Wells and Van Wagenen in the monkey (72,73,74,75,76). These studies demonstrated the masculinization of the urogenital sinus, its derivatives, and the external genitalia, although there was little effect on the mullerian ducts, and ovarian inversion did not occur. Based on experimental animal studies of altered sexually dimorphic behavior in female guinea pigs, rats and monkeys (77,78,79,80,81,82), behavioral masculinization of the female as a result of prenatal exposure to androgens in the human will be rare. The available literature indicates that the effects of androgens on the fetus are dependent on the dose and stage of development during which exposure occurred.
Angiotensin Converting Enzyme Inhibitors
The first angiotensin converting enzyme (ACE) inhibitor, captopril, was introduced in 1981 for the treatment of severe refractory hypertension. Since then the number of ACE inhibitors has increased and now includes enalapril, lisinopril, quinapril, perindopril, fosinopril, ramipril, and cilazapril (83). Their relative effectiveness, combined with a paucity of side effects as compared to other antihypertensives, have made these drugs extremely popular for the treatment of all types of hypertension and congestive heart failure and diabetic nephropathy.
ACE inhibitors are competitive inhibitors of ACE, a carboxypeptidase that forms an integral part of the renin angiotensin system (83). ACE catalyses the conversion of angiotensin I to angiotensin II, one of the most potent vasoconstrictors known. It is the same enzyme as kininase II, and also catalyses the breakdown of bradykinin. A vasodilatory peptide itself, bradykinin stimulates the release of other vasodilatory substances including prostaglandins and endothelium derived relaxation factor (84). Both mechanisms of action contribute to the decrease in blood pressure resulting from ACE inhibi-tion (85).
Although they are considered relatively safe for the treatment of hypertension, the use of ACE inhibitors during pregnancy has been associated with adverse fetal outcomes in both humans and experimental animals. The first case of adverse fetal outcome in humans was reported in 1981 (86). In that report, treatment with captopril began on the 26th week of gestation, oligohydramnios was detected 2 weeks later, and a cesarean section was performed the following week. The child was anuric and hypotensive and died on day 7. The kidneys and bladder were morphologically normal but hemorrhagic foci were found in the renal cortex and medulla. Numerous cases of severe and often lethal adverse fetal effects associated with ACE inhibitor use during pregnancy have since been reported (87,88,89). The most consistent findings have been associated with a disruption of fetal renal function resulting in oligohydramnios and neonatal anuria accompanied by severe hypotension (87,89). Intrauterine growth retardation, pulmonary hypoplasia, hypocalvaria, persistent patent ductus arteriosis, and renal tubular dysgenesis have also been reported (89,90,91). Some of these effects may also result from the condition for which ACE inhibitors were prescribed (87). These effects have been associated with ACE inhibitor treatment only during the second and third trimester. There are no reports of adverse fetal outcome associated with ACE inhibitor use during the first trimester (89,91). As ACE inhibitors do not appear to affect organogenesis in either humans or in animal studies, it is not a classical teratogen. For this reason Pryde and associates (89) have proposed the term ACE inhibitor fetopathy to describe the characteristic syndrome that results from ACE inhibitor use during pregnancy.
The majority of adverse fetal effects associated with ACE inhibitor use during pregnancy result from the direct therapeutic action of ACE inhibitors on the fetus. ACE inhibitors readily cross the placenta in which they inhibit fetal ACE activity (89,92). The decreased renal blood flow caused by vasodilation of renal efferent arterioles results in a loss of glomerular filtration pressure leading to fetal anuria and oligohydramnios (92,93). This in turn may result in other adverse fetal outcomes, such as pulmonary hypoplasia. Fetal urine production and tubular function does not begin until approximately 9-12 weeks of gestation and probably explains the lack of adverse fetal effects when ACE inhibitor treatment is discontinued in the first trimester. Renal dysplasia, in particular a lack of renal proximal tubule differentiation, has also been noted in some effected fetuses (90,93,94).
Exposure to ACE inhibitors during pregnancy has also resulted in several cases of hypocalvaria, an ossification defect of the membranous bones of the skull that leaves the fetal brain inadequately protected (89,90). Although the pathogenesis is still unknown, inadequate perfusion of developing bone as a result of fetal hypotension combined with pressure from uterine muscles as a result of oligohydramnios may explain this defect (90,91). It has also been suggested that ACE inhibitors may affect ossification by acting on osteoblast-derived growth factors (90).
Despite consistent reports of adverse ace inhibitor fetopathy, there are no controlled studies available to assess the risks associated with the use of ACE inhibitors during pregnancy. Because there is no reported incidence of adverse fetal effects as a result of ACE inhibitor use during the first trimester of pregnancy, there is no contraindication to ACE inhibitors in women of reproductive age. If the woman becomes pregnant therapy is then changed to an alternative antihypertensive that represents less risk to the fetus.
Antibiotics
The incidence of intraamniotic infection is about 1% of all pregnancies but 3%-40% of women with ruptured membranes for 24 hours or more (95). Intraamniotic infection is associated with increased morbidity in the newborn including pneumonia, and sepsis. There is also a significant increase in perinatal mortality associated with intraamniotic infection although this is in part related to prematurity.
Increased neonatal mortality and morbidity, especially from group B streptococcal infection, can be largely prevented by intrapartum chemoprophylaxis. Neonatal sepsis is also significantly reduced if mothers receive intrapartum antibiotics. With few exceptions (such as streptomycin) acute exposure to antibiotics in usual therapeutic doses poses little significant risk to the fetus, especially in comparison to the potentially devastating effects of neonatal sepsis.
Aminoglycosides
This class of antimicrobials includes gentamicin, tobramycin, streptomycin and kanamycin. The only drug in this category with confirmed developmental toxicity is streptomycin. Based on case reports, there appears to be a small increased risk of sensorineural deafness in offspring of women treated with streptomycin for tuberculosis during pregnancy (96,97). Other congenital anomalies have not been associated with in utero exposure to streptomycin in the human (98). Because of the risk for ototoxicity, streptomycin should not be given during pregnancy. However, other aminoglycosides, such as kanamycin, appears to have minimal risk of causing similar adverse effects (99,100).
Anti-Tuberculosis Therapy
Drugs prescribed for the treatment of tuberculosis include aminoglycosides, ethambutol, isoniazid, rifampin, and ethionamide. The ototoxic effects of streptomycin are the only proven adverse effects of these drugs on the fetus. Neither ethambutol nor rifampin have been associated with an increase in the incidence of growth retardation, premature birth or malformations (96,97,101,102).
Early reports did not associate therapeutic exposures to isoniazid with an increased risk of malformations (96,97) but there is an unconfirmed association with CNS dysfunction (103,104). There was one attempted suicide involving 50 tablets of isoniazid per day during the 12th week that resulted in a stillbirth with arthrogryposis multiplex congenita syndrome (105). Isoniazid may have small increased risk for adverse effects on the CNS but there is no apparent increase in risk for congenital malformations or abortions with therapeutic exposures. Only one report associated ethionamide with an increased risk of teratogenic effects (106). However, this association is tenuous and not supported by other case reports (107).
As a general observation, the antituberculosis drugs produce adverse effects on the fetus of experimental animals in greater doses than those equivalent to therapeutic exposures and not in all species. Embryotoxicity can be demonstrated for streptomycin in the mouse, kanamycin in the guinea pig, isoniazid in the rat, rifampin in the rat, and ethionamide in the rabbit (108,109,110). While this does not eliminate the possibility of adverse effects on the fetus following exposure to the other prescribed tuberculostatic medications discussed, therapeutic exposures appear to represent a very small risk of teratogenesis and even less risk of abortion.
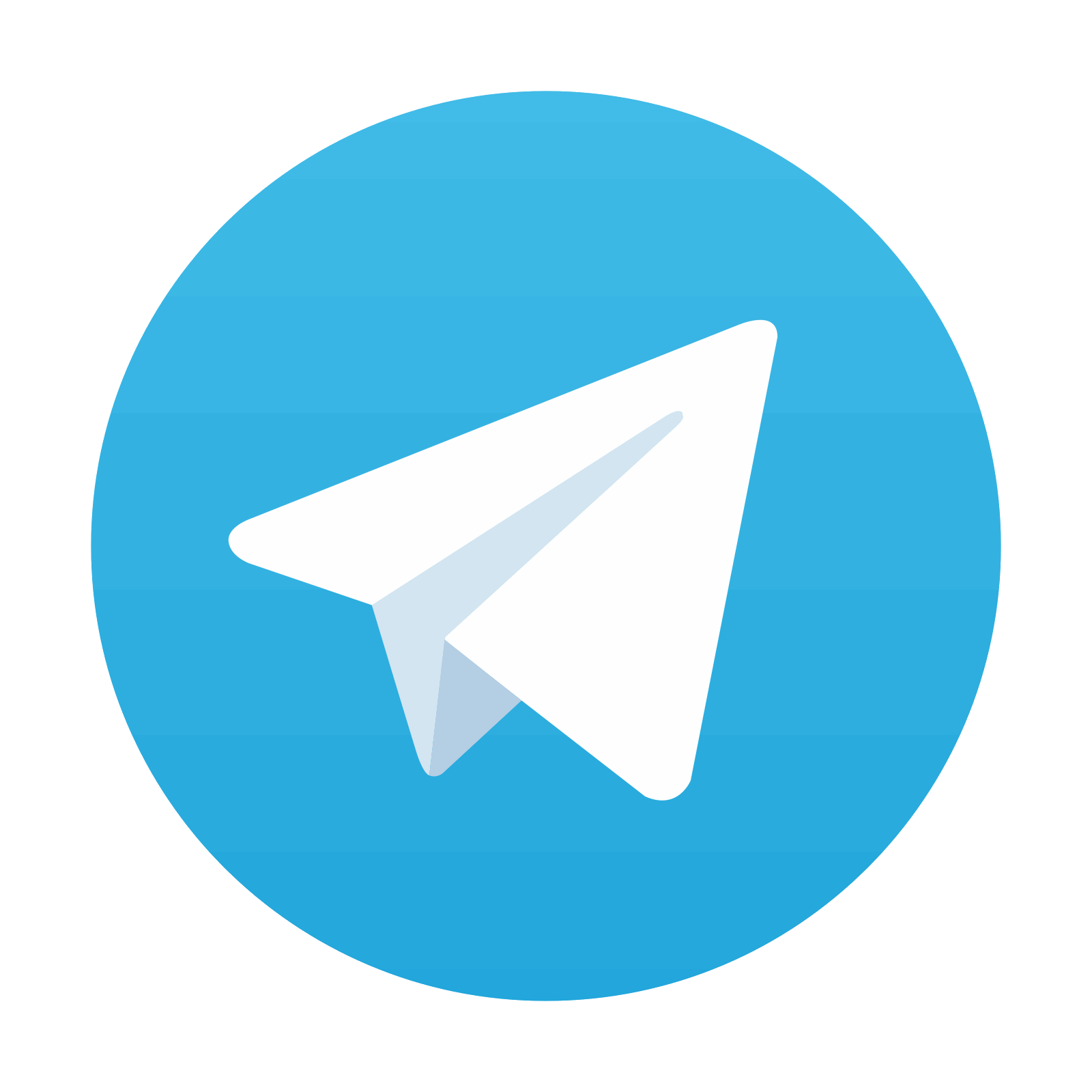
Stay updated, free articles. Join our Telegram channel

Full access? Get Clinical Tree
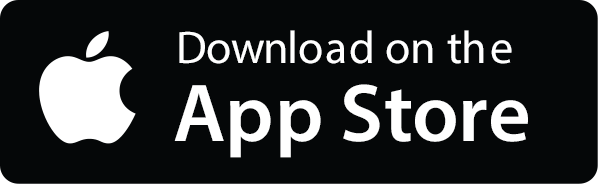
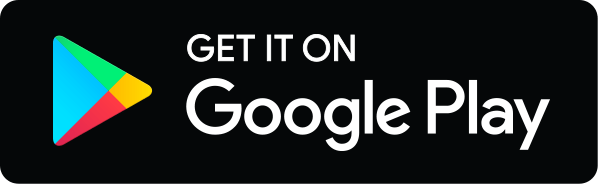