Objective
We sought to investigate the cost-effectiveness of prenatal screening for spinal muscular atrophy (SMA).
Study Design
A decision analytic model was created to compare a policy of universal SMA screening to that of no screening. The primary outcome was incremental cost per maternal quality-adjusted life year. Probabilities, costs, and outcomes were estimated through literature review. Univariate and multivariate sensitivity analyses were performed to test the robustness of our model to changes in baseline assumptions.
Results
Universal screening for SMA is not cost-effective at $4.9 million per quality-adjusted life year. In all, 12,500 women need to be screened to prevent 1 case of SMA, at a cost of $5.0 million per case averted. Our results were most sensitive to the baseline prevalence of disease.
Conclusion
Universal prenatal screening for SMA is not cost-effective. For populations at high risk, such as those with a family history, SMA testing may be a cost-effective strategy.
Spinal muscular atrophy (SMA) is a neurodegenerative disorder characterized by death of the alpha motor neurons in the spinal cord leading to progressive proximal muscular weakness. SMA affects approximately 1 in 10,000 live births and is the leading genetic cause of infant mortality and the second most common autosomal-recessive disorder, after cystic fibrosis.
For Editors’ Commentary, see Table of Contents
See related editorials, pages 207 and 209
SMA is subdivided into 4 groups. Type I, Werdnig-Hoffmann disease, is the most common and the most severe form with onset <6 months of age and death usually within the first 2 years of life, mainly secondary to respiratory failure. Type II is slightly less severe, with onset usually between 6 and 18 months of age. These children are usually able to sit but unable to walk or stand. Type III, Wohlfart-Kugelberg-Welander disease, typically has an onset during the first few years of life, but may be delayed until adolescence, and while these children are usually able to walk they may still have difficulty rising from a seated position. Type IV, or adult-onset SMA, is a mild form of disease that may not be noticed until adulthood, if at all.
SMA is caused by the homozygous deletion of the survival motor neuron (SMN1) gene in 95% of cases. The remaining cases are caused by a heterozygous deletion on one allele and a point mutation on the other. Genetic testing is available to both identify carriers (through polymerase chain reaction identification of a single abnormally deleted SMN1 gene) and to diagnose affected individuals (2 abnormal copies). Although similar to other testing for other genetic conditions with identified mutations, there are several limitations to carrier screening. In all, 5% of the population has 2 normal copies of the SMN1 gene on one chromosome and a deletion mutation on the other. These individuals would not be identified as carriers even though they do possess 1 abnormal SMN1 allele. Additionally, screening cannot determine which of the 4 types an affected individual will manifest.
Current controversy exists as to whether prenatal carrier screening for SMA should be offered to women or couples who are pregnant or planning pregnancy. The American College of Medical Genetics issued a statement saying that “carrier testing should be offered to all couples.” However, the American College of Obstetricians and Gynecologists (ACOG) issued a contradictory statement that “preconception and prenatal screening for SMA is not recommended in the general population at this time.” Moreover, ACOG stated that prior to recommending universal prenatal screening, several issues must be addressed, including cost-effectiveness analysis. As such, our study seeks to further informed debate with regard to carrier screening by investigating whether a policy of universal prenatal carrier screening would be a cost-effective strategy.
Materials and Methods
A decision analytic model ( Figure 1 ) was created with software (TreeAgePro 2008; TreeAge Software Inc, Williamstown, MA). The study is a theoretic decision analytic model, and thus exempt from institutional review board approval as no human subjects were involved. Our theoretic cohort consisted of all women presenting for prenatal care at an early enough gestational age to allow for prenatal carrier screening. We compared a policy of universal carrier screening to that of no screening.

In the no screening arm, we assumed a baseline population risk for SMA of 1 in 10,000. We divided children with SMA into 2 categories: severe disease (type I and some with type II) and milder disease (some type II, and all types III and IV). In the screening arm, women underwent serum carrier screening; if the screen was positive, their partners were also screened. If both partners were identified as carriers, an amniocentesis for fetal diagnosis was performed. We accounted for miscarriages as a result of these procedures. The model assumed that pregnancies were terminated if a fetal diagnosis of SMA was made.
As a baseline, we assumed a 100% uptake rate for each subsequent diagnostic test and that all pregnancies with a fetal SMA diagnosis were terminated. Variation in the choice of pregnancy termination was subject to sensitivity analysis.
Probabilities
Table 1 displays our baseline model inputs. The carrier screening regimen in our model is a serum probe amplification of all exons in the SMN1 gene. Carrier screening is a dosage analysis test; individuals with 50% of normal SMN1 allele levels are identified as carriers. We assumed that there would be no false-positive screens. However, for the 5% of individuals with 2 copies of the SMN1 gene on 1 chromosome, we did allow for false-negative screening. Carrier screening also does not test for more subtle point mutations. Given these limitations, we assumed an overall 90% sensitivity rate for carrier detection.
Variable | Value | Range considered in sensitivity analysis | Reference |
---|---|---|---|
Probabilities | |||
Prevalence of SMA | 0.0001 | 0.0030–0.0001 | |
Proportion of SMA that is severe | 0.7000 | 0.5000–0.9000 | |
Sensitivity of carrier screening | 0.9000 | 0.8000–1.0000 | |
Proportion of cases from de novo mutation | 0.0200 | 0.0000–0.0400 | |
Miscarriage risk following amniocentesis | 0.0029 | 0.0017–0.0050 | |
Costs (2009$) | |||
Carrier screening | $425 | $100–1000 | |
Fetal diagnostic testing | $395 | $100–1000 | |
Amniocentesis | $1277 | $500–3000 | |
Pregnancy termination | $1743 | $1000–4000 | |
Lifetime cost, child with severe disease | $322,126 | $50,000–2,000,000 | |
Lifetime cost, child with mild disease | $819,762 | $500,000–3,000,000 | |
Utilities (maternal) | |||
Fetal loss (miscarriage or termination) | 0.92 | 0.85–0.95 | |
Having a child with severe disease | 0.78 | 0.50–0.90 | |
Having a child with mild disease | 0.81 | 0.50–0.90 | |
Average life expectancy, y | |||
Maternal | 50 | – | |
Child with severe disease | 2 | 1–5 | |
Child with mild disease | ≥50 | – |
In addition, we accounted for de novo mutations. A total of 98% of SMA cases arise in a traditional autosomal-recessive inheritance pattern; however, 2% of cases arise from new mutations. We assumed that these de novo cases occur only in offspring with at least 1 carrier parent. The possibility of 2 de novo mutations producing disease should be exceedingly rare (and there would be no effect of a single de novo mutation in a child carrier). Thus, in our model, we assume that 25% of offspring with 2 carrier parents will have SMA, 0% of offspring with no carrier parents will have SMA, and 0.005% of offspring with only 1 carrier parent will have SMA (calculated assuming a 1 in 10,000 prevalence of disease).
Costs
Cost data were derived through available literature review. All costs were inflated to 2009 dollars using the general Consumer Price Index (CPI). Of note, as cost estimates by category of care were not available (eg, pharmacy, inpatient, physician), the general CPI was chosen to avoid overinflation by using the overall medical care component. A 3% discount rate was applied and a societal perspective was assumed.
Genetic testing costs were available through the Seattle Children’s Hospital Genetics Laboratory World Wide Website. Carrier screening testing was through dosage analysis of multiple ligation-dependent probe amplification of all exons in the SMN1 gene, at a cost of $425 per test. Diagnostic fetal testing was an analysis of exons 7 and 8 of the SMN1 gene from fetal cells obtained during amniocentesis and maternal DNA was compared to fetal DNA to evaluate for maternal cell contamination at a cost of $395 per test. This was in addition to the cost of the amniocentesis procedure itself, which was estimated from the literature.
We could find no direct data from which to estimate the lifetime cost of caring for a child with either severe or mild SMA. As such, we used the best proxies currently available in the literature. For mild disease, we assumed that the lifetime cost of caring for a child would be similar to that of caring for a child with cerebral palsy and used available estimates of total direct and indirect cost of care for such children. We inflated the direct costs of care to 2009 dollars using the CPI. For indirect costs, we used the overall compensation index to inflate the figure. For severe SMA, respiratory complications are the main cause of morbidity and mortality. The need for respiratory support for either treatment or palliation accounts for the vast majority of lifetime cost expenditures. To estimate the cost of respiratory support, we used published data from a national cross-sectional survey of primary caregivers for ventilator-dependent individuals. Direct costs of care included the cost of equipment, utilities, home health personnel, health services (physician, hospital and skilled nursing facility utilization), and monthly annualized costs of 1-time purchases and home remodeling. Indirect costs were also considered, including lost wages for the primary care giver. Estimates were of true cost not charge. We inflated the estimate from 1994 to 2009 dollars using the compensation index for wages and lost income and the CPI for other costs.
Quality-adjusted life years
We only considered maternal quality-adjusted life years (QALYs) in our analysis. Given our model included the possibility of pregnancy termination as a result of information obtained from prenatal screening, considering neonatal QALYs in our model would have biased against any screening regimen. We used a 3% discount rate for QALYs.
For pregnancies that ended in fetal loss (either procedure-related miscarriage or pregnancy termination of an affected fetus) we used a maternal utility of 0.92, which is a standard gamble utility estimate, thus applied to remaining life years. For women who have an affected child with severe disease, we used an estimate of 0.78. This estimate comes from work that used a Likert scale to specifically estimate the impact of type I SMA on parental QALY. We applied this QALY estimate for 2 years only (average life expectancy for a child with severe disease). Beyond the 2 years, we used the reduced maternal QALY of 0.92 associated with fetal loss. For mild disease, there was no available parental QALY estimate. We therefore used the standard gamble estimate for Down syndrome (0.81) as a proxy.
Analysis
We calculated total costs and QALYs to determine the incremental cost-effectiveness of offering prenatal screening for SMA. In addition, we calculated clinical outcomes, including total number of cases of SMA, pregnancy terminations, and procedure-related miscarriages. We considered cost per QALY as well as cost per case of SMA averted.
We performed sensitivity analyses to evaluate the robustness of our conclusions. For univariate analysis, we used ranges available either from literature or estimated through best clinical estimate ( Table 1 ). For those costs and probabilities that appeared to have greatest effect on model outcomes, we performed threshold analysis to determine the range over which the outcome was still cost-effective. Additionally, we conducted a Monte Carlo simulation to test the robustness of our model to simultaneous multivariable changes in probability, cost, and utility inputs. For this simulation, costs were assumed to have a gamma distribution with SD of 50%. This is similar to a normal distribution but with a left skew, which is appropriate for medical costs given that there are often outliers in the upper cost ranges. For probability estimates we used beta distributions with SD of 20%. Beta distributions are the multivariate equivalent of binomial distributions. We ran the Monte Carlo simulation with 100,000 trials.
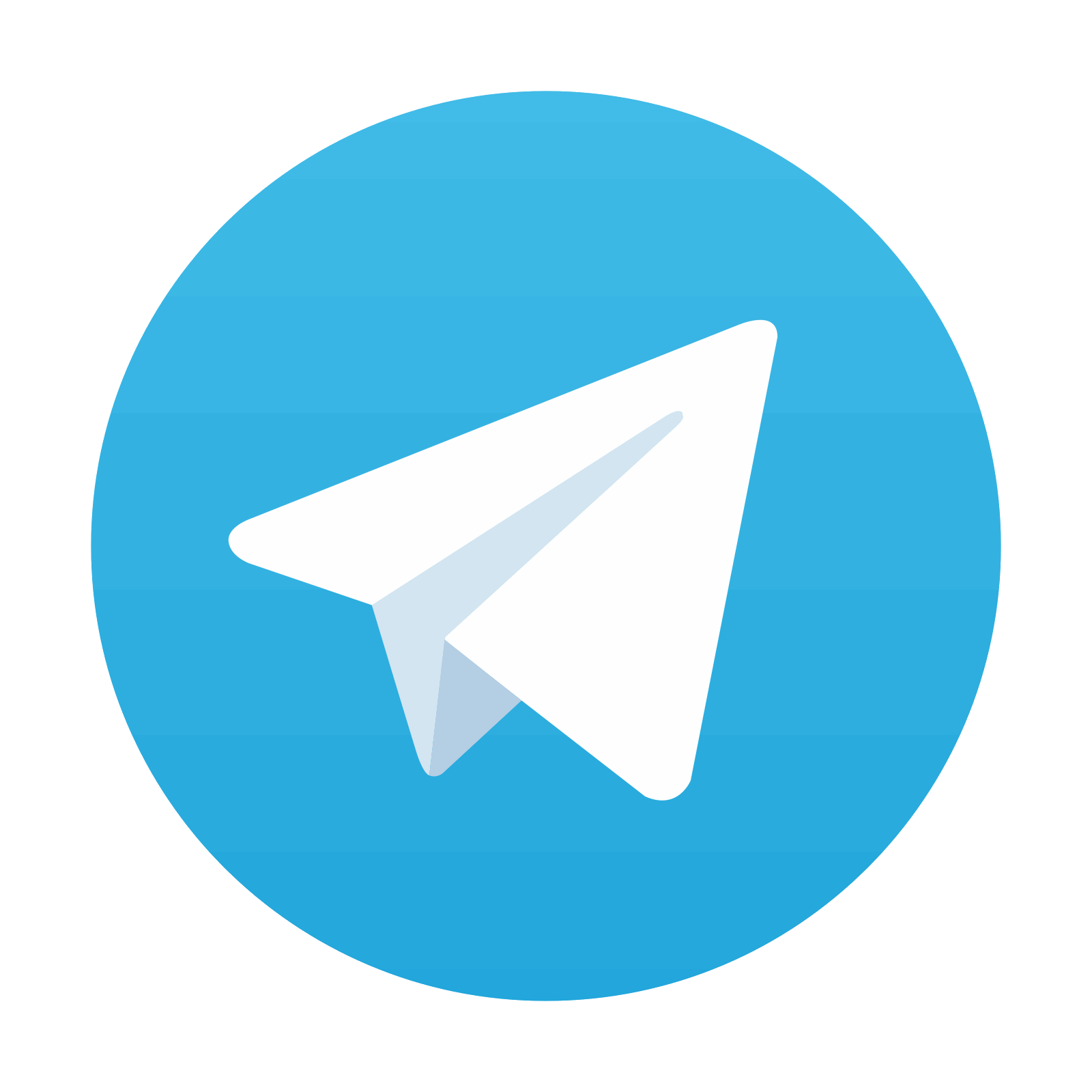
Stay updated, free articles. Join our Telegram channel

Full access? Get Clinical Tree
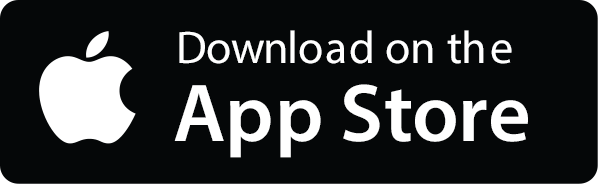
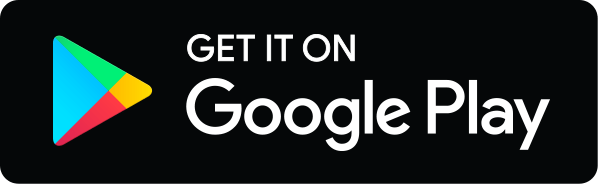