The clinical burden imposed by the collective group of monogenic disorders demands novel therapies that are effective at achieving phenotypic cure early in the disease process before the development of permanent organ damage. This is important for lethal diseases and also for non-perinatally lethal conditions that are characterised by severe disability with little prospect of postnatal cure. Where postnatal treatments are limited to palliative options, intrauterine stem-cell therapies may offer the potential to arrest pathogenesis in the early undamaged fetus. Intrauterine stem-cell transplantation has been attempted for a variety of diseases, but has only been successful in immune deficiency states in the presence of a competitive advantage for donor cells. This disappointing clinical record requires preclinical investigations into strategies that improve donor cell engraftment, including optimising the donor cell source and manipulating the microenvironment to facilitate homing. This chapter aims to outline the current challenges of intrauterine stem-cell therapy.
Introduction
As with intrauterine gene therapy (reviewed by Mattar et al. in this issue of Best Practice and Research Clinical Obstetrics and Gynaecology ), the objective of intrauterine stem-cell therapy (IUSCT) is to remedy a monogenic disease in the fetus through delivery of functioning stem cells that engraft in the host and, through normal proliferation, return the missing protein to normal levels. Allogeneic or autologous stem cells can be used as donor cells, although the latter must undergo genetic manipulation to be suitable for transplantation. The justifications for IUSCT are similar to the rationale used in intrauterine gene therapy, where a disease is selected for treatment that is either perinatally lethal or where early onset pathology and a lack of adequate postnatal therapy results in permanent severe morbidity. Additionally, the selected disease must be amenable to phenotype rescue with stem-cell transplantation in a postnatal recipient. This chapter aims to outline the justifications for IUSCT, candidate diseases, autologous and allogeneic strategies, and discuss briefly the clinical obstacles to surmount before human application.
Applicability of stem-cell therapy to monogenic disease
Certain monogenic diseases have been selected for haemopoietic stem cell therapy (HSCT) in adults or children. Such diseases have in common a primary pathology (e.g. failure of erythropoiesis) that is often accompanied by progressive secondary organ damage (e.g. cardiomyopathy), availability of palliative rather than curative treatments, treatment-related complications that add to the disease burden, and are often lethal if sub-optimally treated.
Haemopoietic stem-cell transplantation is the most extensively investigated form of stem-cell therapy and is potentially applicable to several monogenic disorders in utero ( Table 1 ). Some diseases are treatable and potentially curable with postnatal HSCT, such as the haemoglobinopathies and severe primary immunodeficiencies. Haemopoietic stem-cell transplantation may potentially arrest neurological deterioration in demyelinating or lysosomal enzyme-deficiency syndromes. Recent evidence indicates that allogeneic HSCT, with or without co-transplanted mesenchymal stem cells, can lead to significant biochemical and phenotype improvements in women with recessive dystrophic and junctional epidermolysis bullosa. Ideally, in an environment devoid of erythropoietic competition from host cells, normally functioning donor cells can engraft in haemopoietic niches and re-populate the bone marrow, allowing normal erythropoiesis to resume, restoring erythropoiesis, re-constituting the immune system or providing a steady supply of macrophages (Kupffer cells and microglia), which can traverse the blood–brain barrier and replace deficient enzymes within the nervous system to improve neurological outcomes.
Disease | Authors | Specific disorders | Donor cells | Outcomes |
---|---|---|---|---|
Haemoglobinopathies | Hayward et al., 1998 Westgren et al., 1996 | Alpha-thalassaemia | Paternal bone marrow; liver | Microchimerism observed, but transfusion-dependent |
Westgren et al., 1996 Touraine, 1991 Touraine et al. 2004 | Beta-thalassaemia | Parental and sibling bone marrow or peripheral blood progenitor cells; autologous fetal cells | Variable engraftment; no phenotype rescue and surviving recipients remained transfusion-dependent | |
Westgren et al., 1996 | Sickle cell disease | Fetal liver cells | Transfusion dependent | |
Primary immune deficiency syndromes | Touraine et al., 1989 Wengler et al., 1996 Flake et al., 1996 Westgren et al., 2002 Touraine et al., 2004 | Bare lymphocyte syndrome Severe combined immunodeficiency Chronic granulomatous disease | Fetal liver cells and parental bone marrow cells | Survivors with bare lymphocyte syndrome and severe combined immunodeficiency showed engraftment and good outcomes; fetuses with chronic granulomatous disease showed no engraftment. |
Musculoskeletal disease | Le Blanc et al., 2005 Chan (unpublished data) | Osteogenesis imperfecta | Fetal mesenchymal stem cells | Chimerism with improvement in growth and reduction in fracture incidence |
Inborn metabolic diseases | Flake and Zanjani 1997 Leung et al., 1999 Bambach et al., 1997 | Globoid cell leukodystrophy Hurler syndrome MPS I Niemann-pick disease Metachromatic dystrophy | Fetal liver and parental bone marrow cells | Overall no engraftment reported and poor outcomes |
Applicability of stem-cell therapy to monogenic disease
Certain monogenic diseases have been selected for haemopoietic stem cell therapy (HSCT) in adults or children. Such diseases have in common a primary pathology (e.g. failure of erythropoiesis) that is often accompanied by progressive secondary organ damage (e.g. cardiomyopathy), availability of palliative rather than curative treatments, treatment-related complications that add to the disease burden, and are often lethal if sub-optimally treated.
Haemopoietic stem-cell transplantation is the most extensively investigated form of stem-cell therapy and is potentially applicable to several monogenic disorders in utero ( Table 1 ). Some diseases are treatable and potentially curable with postnatal HSCT, such as the haemoglobinopathies and severe primary immunodeficiencies. Haemopoietic stem-cell transplantation may potentially arrest neurological deterioration in demyelinating or lysosomal enzyme-deficiency syndromes. Recent evidence indicates that allogeneic HSCT, with or without co-transplanted mesenchymal stem cells, can lead to significant biochemical and phenotype improvements in women with recessive dystrophic and junctional epidermolysis bullosa. Ideally, in an environment devoid of erythropoietic competition from host cells, normally functioning donor cells can engraft in haemopoietic niches and re-populate the bone marrow, allowing normal erythropoiesis to resume, restoring erythropoiesis, re-constituting the immune system or providing a steady supply of macrophages (Kupffer cells and microglia), which can traverse the blood–brain barrier and replace deficient enzymes within the nervous system to improve neurological outcomes.
Disease | Authors | Specific disorders | Donor cells | Outcomes |
---|---|---|---|---|
Haemoglobinopathies | Hayward et al., 1998 Westgren et al., 1996 | Alpha-thalassaemia | Paternal bone marrow; liver | Microchimerism observed, but transfusion-dependent |
Westgren et al., 1996 Touraine, 1991 Touraine et al. 2004 | Beta-thalassaemia | Parental and sibling bone marrow or peripheral blood progenitor cells; autologous fetal cells | Variable engraftment; no phenotype rescue and surviving recipients remained transfusion-dependent | |
Westgren et al., 1996 | Sickle cell disease | Fetal liver cells | Transfusion dependent | |
Primary immune deficiency syndromes | Touraine et al., 1989 Wengler et al., 1996 Flake et al., 1996 Westgren et al., 2002 Touraine et al., 2004 | Bare lymphocyte syndrome Severe combined immunodeficiency Chronic granulomatous disease | Fetal liver cells and parental bone marrow cells | Survivors with bare lymphocyte syndrome and severe combined immunodeficiency showed engraftment and good outcomes; fetuses with chronic granulomatous disease showed no engraftment. |
Musculoskeletal disease | Le Blanc et al., 2005 Chan (unpublished data) | Osteogenesis imperfecta | Fetal mesenchymal stem cells | Chimerism with improvement in growth and reduction in fracture incidence |
Inborn metabolic diseases | Flake and Zanjani 1997 Leung et al., 1999 Bambach et al., 1997 | Globoid cell leukodystrophy Hurler syndrome MPS I Niemann-pick disease Metachromatic dystrophy | Fetal liver and parental bone marrow cells | Overall no engraftment reported and poor outcomes |
Limitations of postnatal haemopoietic stem-cell transplantation
Haemopoietic stem-cell transplantation is most commonly achieved with bone-marrow transplantation (BMT), although recently umbilical cord blood transplantation has been used increasingly. Much of our knowledge of the factors determining the success of BMT comes from decades of studying adults and children transplanted for transfusion-dependent beta-thalassaemia major. The Pesaro group experience 3 decades ago helped delineate the key risk factors determining a successful outcome: quality of iron chelation, pre-existing hepatomegaly and portal fibrosis. Thalassaemia-free survival was highest in children with no risk factors who received human leukocyte antigen (HLA)-identical donor marrow, whereas treatment failure and mortality was highest among adults with pre-existing organ damage because of the lower responsiveness of scarred adult tissue to treatment. The evidence is clear that the best outcomes are achieved in younger recipients with early disease and minimal or no organ damage.
Two main issues limit the applicability of this potentially curative treatment. The first is the necessity to overcome the host immune system. As most transplants are carried out using allogeneic donor cells capable of normal haemopoiesis, creation of a tolerant microenvironment is required to facilitate engraftment, commonly achieved with busulfan (to ablate recipient bone marrow) and cyclophosphamide (for immunosuppression). The removal of host stem cells allows the empty bone marrow niches to act as ‘docking stations’ for the engraftment of donor cells, and suppression of the host immune system facilitates donor cell proliferation (in the absence of competition from host cells) and eventual reconstitution of the bone marrow. Inadequate immunosuppression and failure to eradicate massive erythroid hyperplasia lead to graft rejection by the host immune system. Pre-transplant conditioning, however, is associated with bone-marrow suppression, failure to thrive, malignancy, and a 30% mortality rate. Secondly, only 25–30% of women in need of HSCT will find HLA-identical donors. For the remainder, alternative haematopoietic stem-cell sources have been used, although only HLA well-matched unrelated donor and HLA-identical sibling cord blood transplants are acceptable clinical procedures. Most women who qualify for HSCT will therefore remain on life-long palliative treatment.
Rationale for intrauterine therapy
With the knowledge that earlier intervention is likely to achieve greater therapeutic gains, the objective of IUSCT is to correct the genetic disorder early in the evolution of disease, to prevent permanent tissue damage and possibly death where pathogenesis begins in utero , and to leverage on the potential therapeutic advantages offered by the early-gestation pre-immune fetus, which has been discussed in greater detail by Nathwani et al in this issue of Best Practice and Research Clinical Obstetrics and Gynaecology . In brief, tolerance toward the transplanted cells may be induced in the pre-immune fetus before antigen-recognition develops at the end of the first trimester, facilitating engraftment in an immature bone-marrow compartment where there is little competition from host cells. Because of the physical limitation on the quantity of donor stem cells that can be harvested and transplanted, fetal size offers a distinct advantage allowing a greater concentration of stem cells to be achieved in the target organ compared with postnatal recipient several log-fold larger. An ontological window has been demonstrated in potential target organs, such as the central nervous system, which may be a function of the temporal appearance of cell surface receptors and metabolic pathways and the more permissive state of restrictive barriers such as the blood–brain barrier. Fetal tissue in the absence of extensive cellular damage has a greater capacity for healing and responsiveness to therapy compared with scarred adult tissue setting the stage for more complete recovery. Additionally, unique characteristics of human fetal stem cells facilitate use as gene-transfer vehicles. Fetal stem cells are more susceptible to vector transduction, and show a higher proliferative, self-renewal and differentiation capacity than postnatal and adult stem cells. Fetal hematopoietic stem cells (HSC) engraft several-fold more efficiently than umbilical cord or adult stem cells, and their presence is detected in every part of the fetus, owing to widespread distribution of progenitor cells during development. Another emerging cell type being considered for IUSCT is the human fetal mesenchymal stem cells (hfMSC), which have longer telomeres, express significantly higher amounts of telomerase compared with adult mesenchymal stem cells (MSC) and efficiently differentiate into muscle and neuronal lineages. Human fetal mesenchymal stem cells are readily transduced by integrating vectors and do not express HLA-II or the co-stimulatory molecules CD80 and CD86 necessary to prime T cells, suggesting a usefulness for both cell-based gene therapy and allogeneic transplantation. The rapidly-expanding fetal bone marrow allows continuous development of haemopoietic niches, which encourage donor cell engraftment and bone-marrow repopulation. This evidence supports the argument in favour of IUSCT for well-selected diseases.
Natural and experimental evidence supporting intrauterine stem-cell transplantation
The goal of stem-cell transplantation is to achieve effective chimerism where engrafted donor cells produce sufficient levels of the missing protein. Natural chimeras are well described in mammals as a consequence to circulation of ‘donor’ blood in the recipient’s vascular system during gestation. Cattle twins demonstrate life-long chimerism in the bone marrow and liver, and non-human primate twins show mutual tolerance and chimerism of both lymphocyte and monocyte population in the bone marrow. In human twins, shared placental circulation or feto–fetal transfusion allow intrauterine exchange of circulating HSC, which in turn facilitates chimerism of donor erythroid, myeloid and lymphoid cells in the recipient, indicating mutual immune tolerance. Chimerism is also described in dichorionic diamniotic twins. These models provide the proof of principle that has inspired attempts at experimentally replicating donor HSC transplantation in utero .
Candidate diseases and supporting evidence for autologous and allogeneic intrauterine stem-cell transplantation
Two main groups of candidate diseases exist. First, conditions that result in perinatal mortality or irreversible end-organ damage in surviving infants, such as alpha-thalassaemia and type I mucopolysaccharidosis, which are amenable to postnatal HSCT, are obvious candidates for fetal therapy. The second group comprises non-lethal diseases with early postnatal onset where postnatal options are of limited effectiveness or mainly palliative. This group includes beta-thalassaemia and sickle-cell anaemia, muscular dystrophies and other lysosomal storage disorders.
Stem-cell transplantation can be carried out with autologous or allogeneic donor cells ( Table 2 ). The main advantage of the autologous approach is the possibility of circumventing the host immune system. For the purpose of treating monogenic disease, autologous cells will first require ex-vivo genetic manipulation with an integrating vector to permanently express the correct transgene. Autologous HSC have been used as gene-transfer vehicles in the treatment of children and adults with beta-thalassaemia major, severe combined immunodeficiencies, the leukodystrophies and Wiskott–Aldrich syndrome. Experimental data on autologous IUSCT in sheep and dogs have demonstrated overall poor effectiveness despite moderate engraftment. The main challenges are harvesting a sufficient number of stem cells from the first-trimester fetus for transduction, failure of which precludes the patient from treatment, creating an environment that is permissive to sustained engraftment, currently achieved with non-myeloablative bone-marrow conditioning. Autologous transplantation is likely to present significant technical obstacles for clinical translation because of the narrow window in which to confirm the diagnosis, carry out a minimally invasive procedure to harvest fetal stem cells, transduce the cells in vitro and carry out transplantation, all preferably before the end of the first trimester to induce tolerance to the transgenic protein.
Author | Animal and disease model | Experimental approach | Outcomes |
---|---|---|---|
Hajdu et al., 1996 | Mouse | Allogeneic fetal murine liver cells transplanted at midgestation. | Chimeric mice tolerated donor-specific skin graft. |
Hayashi et al., 2002 | Mouse | Allogeneic adult bone marrow cells. | High-dose donor lymphocyte infusions allowed conversion into complete donor cell peripheral blood chimerism. |
Hayashi et al., 2003 | Mouse (Beta-thalassaemia and sickle cell disease models) | Allogeneic fetal liver or adult bone marrow derived donor cells. | Higher chimerism with fetal liver cells in both disease models; unsustained phenotype improvement despite stable engraftment. |
Frattini et al., 2005 | Mouse (ATP6a3 deficient model of (autosomal recessive osteopetrosis) | Allogeneic HSC from green fluorescent protein transgenic mice | Dramatic phenotype rescue with engraftment sustained for variable periods. |
Shaaban et al., | Mouse | Allogeneic HSC supplemented with stem cell factor and vascular endothelial growth factor | Improved short-term chimerism, and tolerance but long-term engraftment unchanged. |
Vanleene et al., 2011 ; Guillot et al., 2008 | Mouse (osteogenesis imperfect) | Xenogeneic human fetal MSC | Significant reduction in fracture frequency with production of COL1a2 protein by engrafted cells. |
Chan et al., 2007 | Mouse (muscular dystrophy) | Xenogeneic human fetal MSC | Widespread long-term engraftment with donor myogenic differentiation; low-level chimerism. |
Moreno et al., 2012 | Rabbits | Allogeneic fetal rabbit liver MSC | Long-term engraftment with degree of engraftment related to gestational age at injection. |
Noia et al., 2004 | Sheep | Xenogeneic transfer of human umbilical cord blood HSC. | Bone-marrow chimerism detected. |
Hedrick et al., 1994 | Sheep | Allogeneic adult ovine HSC transplanted into 0.4G ovine fetuses. | Chimerism of adult donor cells did not facilitate tolerance to donor-specific renal allografts. |
Shaw et al., 2011 | Sheep | Autologous amniotic fluid MSC to determine efficacy for cell-based gene therapy. | Amniotic fluid-derived mesenchymal stem cells collected at 0.4G, transduced with LV-GFP, ontraperitoneal injection at 0.5G. Widespread cell distribution and green fluorescent protein expression. |
Zanjani et al., | Sheep | Human adult bone marrow HSC. | Transplanted human HSC from adult bone marrow resulted in successful engraftment and long-term multi-lineage expression and long-term marrow repopulation in secondary recipients. |
Almeida-Porada et al., | Sheep | Adult or fetal sheep HSC. | Co-transplantation of sheep stromal cells with donor HSC lead to increased donor haematopoiesis. |
Oppenheim et al., 2001 | Goat | Allogeneic fetal liver and adult bone marrow cells. | Low levels of chimerism despite genetic similarities and pre-immune fetuses. |
Lovell et al, 2001 | Goat | Allogeneic male fetal liver HSC injected into female fetuses. | Short-term chimerism detectable for only a few days. |
Blakemore et al., 2004 | Canine | Allogeneic adult bone marrow cells. | Microchimerism across several tissues not influenced by gestational age or cell dose. |
Petersen et al., 2007 | Canine | Allogeneic donor cells with anti-T cell antibodies. | Microchimerism. |
Shields et al., 2005 | NHP | Allogeneic mobilised peripheral blood or HSC derived from bone marrow. | No discernible difference in engraftment. |
Shields et al. | NHP | Allogeneic adult bone marrow HSC with varying levels of T-cell depletion. | Engraftment increased with increasing number of T cells within donor cell graft. |
Mychaliska et al., 1997 | NHP | Allogeneic intrauterine paternal bone marrow cell transplantation to improve tolerance of postnatal kidney transplantation. | Less than 0.1% microchimerism in bone marrow. Increased short-term survival of renal allograft in chimeric monkeys. |
Shields et al., 2004 | NHP | Allogeneic cells; fetuses received immune suppression or postnatal donor stem cells boost to examine methods with which to boost chimerism. | Low-level chimerism with both interventions. |
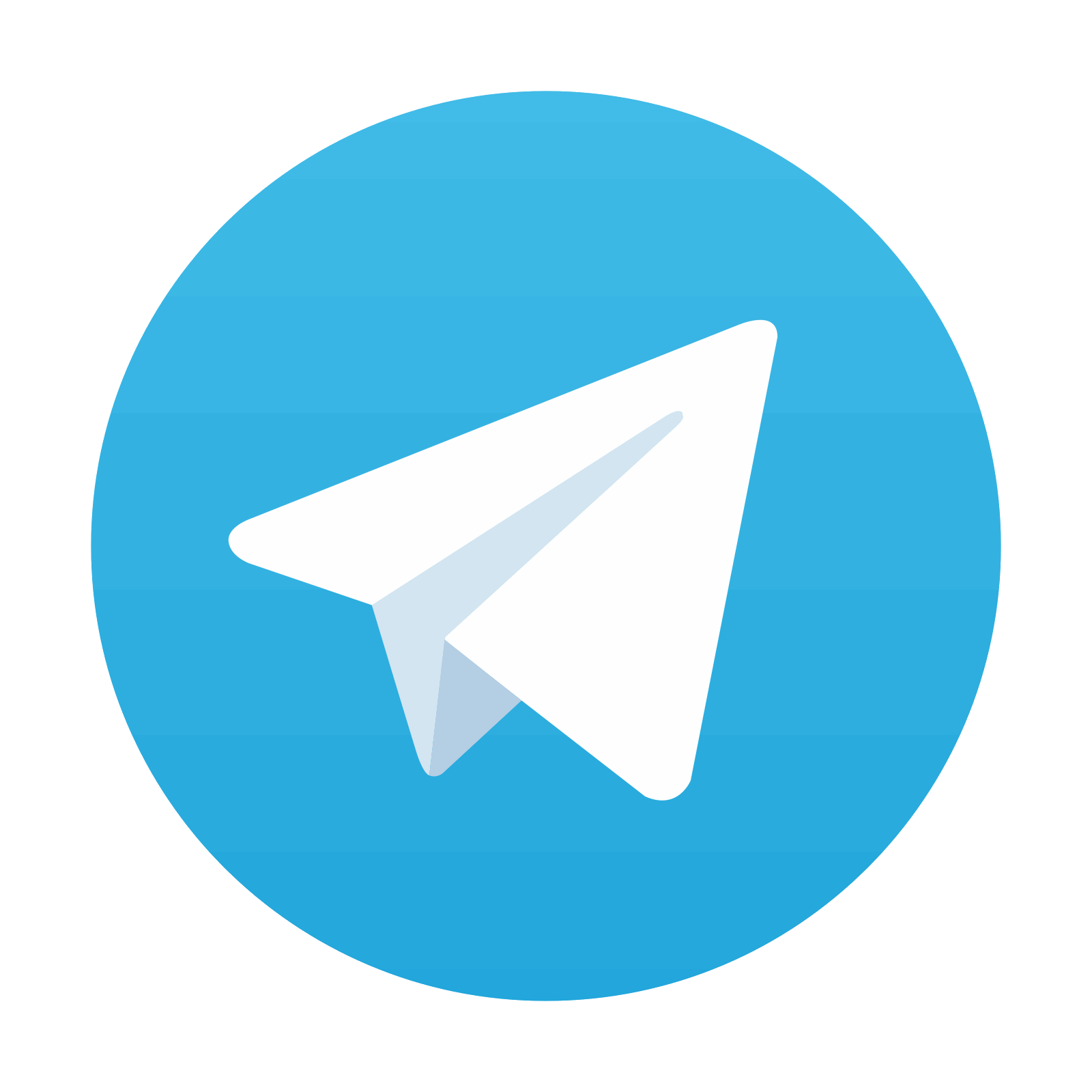
Stay updated, free articles. Join our Telegram channel

Full access? Get Clinical Tree
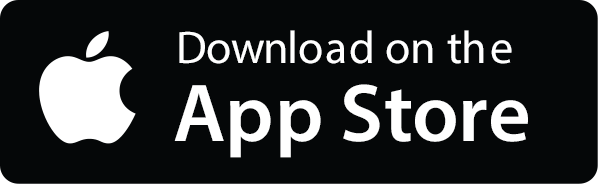
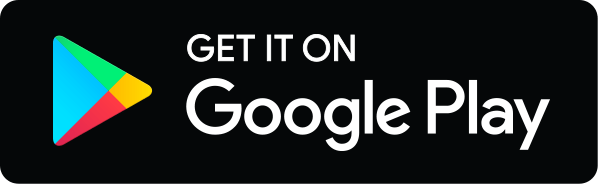