Introduction
Forensic science is the application of a broad spectrum of scientific disciplines to assist in legal decision making. The modern use of forensic evidence in sexual assault cases has evolved over the past century. Forensic evidence collected in emergency departments and sexual assault centers focuses on the transfer of body fluids and other materials from perpetrator to victim during the sexual encounter. The primary focus of this evolving quest is to establish the identity of the perpetrator.
Serum proteins and antigens, particularly those that are highly polymorphic, (having multiple alleles) with varying distributions in the population, became essential to analyzing body fluids collected in sexual assault cases. By the mid 1970s, evidence samples were analyzed using multiple polymorphic blood group antigens plus a few serum proteins, and calculations were made of the probability of a random match between a suspect and the evidence sample based on the distribution of the genotypes in the general population. These calculations often gave very small probabilities for a match between two unrelated individuals, but the sensitivity and specificity of the genetic markers were limited. More powerful, stable, and reliable methods were desirable. Forensic identification technology changed abruptly in 1985 with the introduction of DNA methodology to criminal investigation, and use of DNA testing was widespread by the late 1990s. Ultimately, the goal of any physical evidence examination is to perform presumptive testing through serological, chemical, or other methods to identify materials gathered from the victim, the suspected perpetrator, and the crime scene that have the greatest potential to yield valid DNA profiles.
This chapter discusses the application of forensic evidence testing in cases of sexual assault (more specifically, in cases of child sexual abuse and assault), the role of trace evidence, and the scientific and legal interpretation of the results of laboratory testing of evidence. Two general types of forensic evidence are used in sexual assault cases, transfer evidence and identification evidence. Transfer or trace evidence includes foreign materials found on the surface of the victim’s body or clothing that were transferred to the victim from the abuser or from the crime scene. Identification evidence includes biological materials primarily bodily fluids that can be used to identify the abuser.
Analysis of Bodily Fluids
Semen/Seminal Fluid
Semen, or seminal fluid, is a complex fluid derived from the prostate and other accessory sex glands. Semen is composed of two major components, the cellular fraction and the seminal plasma. The ejaculate is consists of up to 10% spermatozoa (220-300 million sperm cells) and 90% seminal plasma. The cellular fraction contains spermatozoa, plus other cellular elements including epithelial cells. The seminal plasma or fluid component of seminal fluid is derived primarily (65%-75%) from the seminal vesicles. It contains carbohydrates, proteins, citric acid, and zinc. Screening tests for prostatic acid phosphatase (PAP), prostate specific antigen (PSA or p30), and semenogelin in seminal plasma have been used to detect the presence of seminal fluid. PSA has a higher sensitivity than semenogelin or PAP, but semenogelin has higher specificity.
Alternate light sources (ALS) also are used in screening for the presence of semen, displaying a high sensitivity approaching that of the chemical tests, but a lower specificity due to common fluorescence of other materials. ALS have the advantage of not consuming any of the sample. Maximal semen fluorescence is produced by visible blue light at wavelengths between 450 and 490 nm. Standard Wood’s lamps or ultraviolet lamps with shorter wavelengths, including those with old bulbs, might not cause semen to fluoresce. Selecting alternative light sources that produce light with wavelengths between 450 and 490 nm will maximize sensitivity and specificity. ,
The forensic application of DNA technology to biological evidence takes advantage of the greater stability of DNA compared with the enzymes and proteins used in serological testing. Compared with serological markers, DNA allows evidence to be extracted from much smaller amounts of material, and increases the specificity of the match between the evidence sample and the suspect’s DNA. , DNA from every human except identical twins is unique because of polymorphisms, the occurrence of more than one allele of a genetic marker at the same locus on the DNA strand. On average, 3 million base pairs differ between any two individuals. Polymorphisms may be length-based or sequence-based. Length-based polymorphisms are repetitive DNA sequences found within 20% to 30% of noncoding DNA. Many repetitive regions vary in the number of core repeats between different individuals, and are designated “variable number tandem repeats” or VNTR loci. The “short tandem repeats” or STR loci are particular repetitive regions that are used in nuclear DNA profiling. Sequence-based polymorphisms are differences in one or more base pairs in similar length DNA fragments at a particular location in the genome. These point mutations are also known as “single nucleotide polymorphisms” or SNP loci. SNP tests and mitochondrial DNA tests are examples of sequence-based tests.
The VNTR loci had the advantage of having a large number of alleles to produce high discrimination power, but analysis was limited by requiring a relatively large amount of high quality DNA and by being very time consuming. Polymerase chain reaction (PCR) amplification increased the sensitivity of DNA testing by allowing amplification of even very tiny amounts of degraded DNA into the quantities required for analysis. Smaller size variable areas, the STR loci, could be used increasing both sensitivity and specificity because STR loci are more numerous than VNTR loci. Automated technology has allowed for more efficient, rapid, and simultaneous analysis of multiple STR loci, known as “multiplexing,” leading to the development of national DNA databases for use in criminal identification. The United States’ Combined DNA Index System, or CODIS, uses 13 specific STI loci identified by the Federal Bureau of Investigation (FBI) to create a database for use in matching crime scene materials to previously typed individuals. “Single nucleotide polymorphisms” (SNP loci) represent single point mutations that are very numerous, but typically produce only two alleles. Therefore a relatively large number of SNP loci would have to be analyzed to provide significant discriminatory power for forensic purposes.
Mitochondria contain their own small DNA genomes known as mitochondrial DNA (mtDNA). Normally mtDNA is inherited only from the mother. Cells contain only one nucleus with one copy of nuclear DNA, but the cytoplasm of each cell contains multiple mitochondria with up to thousands of copies of mtDNA. The forensic advantage is that specimens with absent or highly degraded nuclear DNA can be analyzed if mtDNA is present. The mtDNA units are smaller, containing only a fraction of the genome compared with nuclear DNA, so mtDNA lacks the discriminatory power or specificity of nuclear STR loci. The resulting discriminatory power of mtDNA sequencing is on the order of one in a few hundred.
The Y chromosome is transmitted as a single copy only from fathers to sons, and contains hundreds of recognized STR loci. Y chromosomal analysis is particularly useful in resolving mixtures of DNA from different males, when the male component of mixtures is completely masked by female DNA in sexual assault cases, and when some seminal fluid is present but no intact sperm remain. The overall discriminating power of Y chromosomal DNA is compromised by the relatively limited portion of the genome on the Y chromosome and because the results are incompatible with the nuclear DNA databases. ,
DNA analysis can result in three determinations regarding identity: exclusion, inconclusive, and match. “Exclusion” means the two DNA samples are different, “inconclusive” means the results cannot confirm similarity or difference, and a “match” means the DNA types are similar with no significant differences. Somewhat less than half of DNA tests result in a match of the suspect to the crime. The current technology for DNA typing has progressed to the point where the reliability and validity of properly collected, properly preserved, and properly analyzed DNA data should not be in doubt. However, the analysis can only yield probabilities and absolute certainty is beyond the realm of science. ,
Blood
Most screening tests for the presence of blood are based on the peroxidase-like reaction of the hemoglobin molecule with chemical agents including luminol, phenolphthalein, leucomalachite green, and several proprietary reagents. The specificity of the tests is limited by frequent cross-reactivity with iron and copper containing salts and certain vegetables and fruits containing peroxidase enzymes. When tested against five other agents, luminol had the highest sensitivity and specificity, and did not destroy the DNA contained in the samples. Newer tests based on using anti-human hemoglobin antibodies to identify human blood have very high specificity, but they have a lower sensitivity than the chemical tests. Alternate light sources (ALS) can also be used to detect blood, with blood having a strong narrow absorption band for fluorescence at about 415 nm. Luminol is significantly more sensitive than ALS in detecting blood. Blood found on body surfaces, body orifices, or clothing of sexual assault victims often comes from the victim, but sometimes it can originate from the perpetrator. Although the density of DNA is lower in blood than in seminal fluid, the white blood cells are nucleated and provide a relatively good source of DNA evidence.
Identification using conventional serology is based on the fact that approximately 80% of the humans are “secretors”; all their bodily fluids including semen, saliva, vaginal secretions, and perspiration will contain the genetic markers found in their blood or serum. The frequencies of the various alleles of individual markers in the general population are known, and the probability of multiple markers occurring in a single person can be calculated for specific combinations of alleles. At one time the most commonly used genetic markers were the ABO blood group antigens, Lewis blood group antigens, phosphoglucomutase, glycooxalase I, and peptidase A, augmented with other blood group antigen systems and polymorphic enzymes to improve discrimination.
DNA testing replaced serology because of the serology’s significant limitations in both sensitivity and specificity. Approximately 20% of humans are “nonsecretors” with no genetic markers in body fluids other than blood. The effects of proteolytic enzymes present in semen, and bacterial and environmental degradation, can result in the rapid loss of markers in blood, semen, and saliva, leading to erroneous results. Furthermore, the genetic markers in semen are rapidly degraded in vaginal secretions after intercourse, usually after 6 hours, but can persist longer in dry stains.
Saliva
Human saliva is 98% water, but also contains electrolytes, mucus, antibacterial compounds, α-amylase, lysozyme, lingular lipase, and cellular material. As many as 8 million human cells and 500 million bacterial cells are present per milliliter of saliva. Forensic testing of suspected saliva samples includes screening tests that typically identify the presence of α-amylase. The α-amylase in saliva hydrolyzes complex carbohydrates such as starch into oligosaccharides, and this reaction produces a characteristic color change, indicating a positive result.
Obstacles in presumptive saliva screening include the innate variation of salivary amylase levels among humans, difficulties in interpreting color change-based tests, body fluid specificity issues, and relatively poor sensitivity compared to screening tests for blood and semen. Salivary α-amylase is found in lower concentrations in other body fluids such as perspiration, tears, breast milk, urine, serum, feces, and semen. Pancreatic α-amylase can be found in vaginal secretions, urine, serum, fecal material, and semen. A ubiquitous enzyme, α-amylase also is found in many plants and in the saliva of pigs, rodents, elephants, and nonhuman primates.
Screening for saliva in the clinical setting is often based on a history of oral contact or presence of bite marks; however, the use of multiwavelength or “tunable” alternate light sources are helpful in finding stains that might contain semen or saliva. , This technique can also be used in the laboratory for presumptive identification of saliva and semen stains on fabric. An advantage of this technique is the preservation of the original samples, allowing the entire specimen to be available for DNA processing. , Maximal saliva fluorescence is produced by visible blue light at wavelengths around 450 nm and saliva detection is poor at shorter wavelengths of 415 nm or less. Selecting “ultraviolet lamps” or alternative light sources that produce light with wavelengths of 450 nm will maximize sensitivity.
Following a positive screening test for saliva, DNA testing can be performed on the cellular component of the saliva using STR analysis. The major limitations are the relative insensitivity of available tests for identifying potential saliva stains and the relatively low density of cellular material in saliva stains.
Trace Evidence
Trace evidence includes hair, fibers, paint, polymers, glass, soil, plant materials, impressions, cosmetics, lubricants, other chemical residues, and even epithelial cells. Hair and fiber evidence are the predominant types of trace evidence analyzed in sexual assault cases. Locard’s exchange principle states that whenever two objects come into contact, a transfer of material will occur. Trace evidence that is transferred can be used to associate objects, individuals, or locations. The integrity and significance of trace material as associative evidence and as potential identity evidence relies on proper detection, collection, and preservation.
A variety of instruments and visualization tools are used to analyze trace evidence. Trace evidence analysis begins with microscopic techniques using visible, polarized, and ultraviolet light. Microspectrophotometry, infrared spectroscopy, thin-layer chromatography, liquid chromatography, and mass spectrometry supplement microscopy when appropriate.
Scalp, pubic, or body hair from the perpetrator occasionally can be found on the victim’s body. Scalp hairs have the most interpersonal variation, and facial, axillary and body hairs the least amount of interpersonal variation. Microscopic analysis of scalp and pubic hairs are often conducted, but comparisons of hair from other body regions are less significant and less frequently conducted. Exline et al studied the transfer of pubic hair during consenting sexual contact and found at least one hair transfer in 17.3% of pubic hair combings. Transfers to males (23.6%) were more common than transfers to females (10.9%). Pubic hair transfer in actual sexual assault cases, however, show a much lower rate of transfer. , Mann reported only 4% of pubic hair combings submitted for forensic analysis revealed hair transfer from suspect to victim. Examination of the undergarments of victims showed a transfer of head hair and pubic hair from the suspect in 4% and 3% of cases, respectively. Examination of the outer clothing of both victims and suspects indicated a transfer rate of head hair of approximately 14% and of pubic hair of 1% to 3%.
Hair analysis requires the collection of suspicious “foreign” hair from the body of the victim, and collection of multiple specimens from the alleged perpetrator and the victim. Hair features such as color, shaft configuration, cross-sectional shape, pigment distribution, hair diameter, and cuticle can be used to classify a hair. Significant variation occurs in scalp hairs of a single individual. The use of dyes, rinses, permanents, and other chemical applications, and environmental exposure to excessive sunlight, wind, and dryness can cause even greater changes in microscopic appearance. Animal hairs can also be transferred as trace evidence, and can be determined to belong to a specific species using microscopy.
When the identity analysis of individual hairs with nuclear or mtDNA is compared with microscopy, a false positive rate of 11% to 20% is found with microscopy. Associations between hairs using microscopy are helpful in determining which hairs to send for DNA analysis. In addition, 86% of hairs unsuitable for microscopic comparison or determined to be inconclusive had sufficient mtDNA available for analysis. Microscopic analysis has limited specificity and the laboratory can only conclude in the majority of cases that the sample is a consistent, inconsistent, or inconclusive match when compared with the perpetrator’s hair.
Scalp hair transfer is a complicated process, including primary and secondary transfer, and can be influenced by many environmental and personal variables. Individuals shed approximately 100 head hairs every day and can transfer them to other individuals. Secondary transfer from clothing or other surfaces, persistence of hair despite laundering, and known transfer during laundering, decreases the evidentiary value of hair when a suspect and victim live together or launder their clothing together, as is the case in many child sexual assaults. Most shed hairs lack nucleated cells so they are not amenable to nuclear DNA analysis. These hairs might contain sufficient mitochondrial DNA for analysis of mitochondrial DNA.
The transfer of fibers has been studied extensively, and fiber transfers or exchanges of fibers between garments occur with every contact between two pieces of clothing. Fiber transfers are dependent on a number of variables including the type of contact, the types of garments/fabrics making contact, and fiber length. Transferred fibers generally are lost rapidly, but some transferred fibers even persist on fabrics that have been washed or dry cleaned. Secondary transfer of fibers may occur from horizontal surfaces to clothing. Microscopic comparison of fibers found on the victim’s body with known fibers from the perpetrator’s house can help confirm the location of the abuse unless they live in the same house. Proper handling of fiber evidence maximizes the value of fiber analysis. Microscopic analysis has limited specificity and the laboratory can only conclude in the majority of cases that the sample is consistent, inconsistent, or inconclusive when compared with known fibers.
DNA containing materials including skin cells, blood, saliva, or semen from a perpetrator can be collected from fingernails, particularly if the victim has scratched the perpetrator during the assault. This material is often collected as part of the sexual assault forensic evidence kit by scraping under the nails, swabbing under the nails, or clipping the nails. Individual clinical casework commonly yields a mixture of perpetrator and victim DNA. However, experimental studies suggest clippings are minimally helpful and gentle scraping or swabbing beneath the nails is more likely to detect useful evidence. Gentle techniques appear to be superior to thorough scraping with a sharp instrument.
Epithelial cells can also be transferred through physical contact, providing another potential source of DNA for analysis. The estimated detectible primary transfer between individuals or from an individual to a surface is approximately 20 to 1000 cells. A secondary transfer theoretically is possible but is considered unlikely. The average human sheds approximately 400,000 skin cells every day, with each nucleated skin cell containing about 5 pg of nuclear DNA. Multiplex PCR DNA analysis can produce full DNA profiles to identify individuals at or below 100 pg of purified DNA, therefore as few as 20 cells may be sufficient to produce an individual’s DNA profile. Direct physical contact, however, is not the only way epithelial cells are transferred. Indoor dust is composed largely of epithelial cells, containing more than 10 µg (10 million pg) of total DNA per gram of dust, and the DNA is stable in the dry state. Environmental dust transfer can produce one or more DNA profiles from individuals not related to the crime being investigated. Therefore, epithelial cell analysis is usually not done in sexual assault, other than from fingernail scrapings.
Clinical Considerations
Protocols have long recommended forensic evidence collection to detect sperm, seminal fluid, and serological markers, when the examination occurs within 72 hours of acute sexual assault or sexual abuse. The increasing sensitivity of DNA analysis and the increasing importance of DNA evidence in prosecution of acute sexual assault of adults and adolescents has prompted some centers to extend evidence collection timeframes from 72 to 96 up to 168 hours following a sexual assault. There is little scientific evidence to support obtaining anal/rectal samples unless history or trauma support anal penetration, and when these samples can be collected within 24 hours of the sexual penetration (see Chapter 13 ). Studies do not support an extended timeline for collection of evidence in young children, and there is no other published data assessing the utility of any DNA testing in prepubertal or young pubertal children. The reasons that young children have significantly lower rates of positive tests for forensic evidence than adults might include differences in the dynamics of the assault and physiological differences. Certainly, the collection of clothing and linens should be performed beyond 24 hours in children.
Common reasons for unprocessed materials or uninterpretable results from clinical specimens from sexual assault cases include insufficient sample quantity, sample degradation (through action of bacteria, enzymes, and environmental factors), and sample purity (including impurities present within the sample and contamination during or subsequent to obtaining the sample). The primary reason for unprocessed materials, however, is the limited availability of proper equipment and technicians for processing of DNA evidence, leaving public laboratories with the resources to analyze only “the most serious cases.” The ability to introduce findings in court is greatly impacted by the evidence collection process itself and documentation of evidence collection and evidence handling. Improper collection techniques, lack of proper documentation of collection and processing, and not maintaining a chain of custody of the samples from time of collection through completion of laboratory analysis can result in uninterpretable or unreliable results.
Legal Issues
Before 1985, legal issues related to identity evidence concerned the relative lack of specificity of serological testing methods. , During the initial years of DNA analysis, the admissibility of DNA as an accepted scientific methodology was a major question. As DNA evidence has become widely accepted in the legal setting, the challenges have shifted from admissibility to reliability of collection, preservation, and handling of the biological evidence on which DNA analysis is performed. DNA evidence is accepted as being reliable in theory, but legal challenges now focus on suggesting critical mistakes were made from the time of collection through completion of the analysis that invalidate the results. Other authors suggest the principal problems in forensic science in general involve issues of judgment, ethics, and attitude, not inadequate technology or poor quality control. The real challenge is finding ways to preserve the impartiality and independence of both private and public laboratories within an intentionally adversarial legal system. Meeting this challenge could require a cultural change.
A second legal issue is the potential for misinterpreting trace evidence from the environment. Theoretically, low copy-number or low-template DNA processing could replicate a limited size DNA sample from a single cell into samples that allow DNA analysis. Despite the high sensitivity of this process, the resulting DNA profiles have lower specificity than conventional DNA analysis. The high number of replication cycles increase the risk of creation of false alleles and allele drop out if one allele of a heterozygote locus in amplified while the other is not. Unfortunately, the increased sensitivity of this process also increases the potential for analyzing trace DNA from a person unrelated to the crime or the crime scene, or from contamination of evidence following the crime. Furthermore, it is impossible to determine of the type of cell from which the DNA originated, when that sample was deposited, or when or how the DNA became a part of the sample. Since many children are sexually abused by individuals who are well known to them, those same individuals are likely to contribute trace DNA to the environment if they live in the same house or frequently visit, sleep in the same bed, sit on the same couches, and use the same or adjacent towels in the bathroom. The same concern can be raised about the presence of other trace evidence from household or other frequent contacts collected in cases involving child victims.
A third issue is the interpretation of the results in the courtroom. A full conventional DNA profile (13 STR loci) will have a match probability to a randomly selected member of the general population of approximately 1 in 1 billion to 1 in 1 trillion. Partial profiles from degraded or limited cellular material will not allow comparison of as many STR loci, decreasing the match probability. Matching a sample from the crime scene with a smaller, selected population, such as those individuals contained in the CODIS data base also decreases the match probability because the size of the comparison population is much smaller. Using likelihood ratios rather than a profile probability in these cases more accurately represents the probability of the sample DNA coming from an individual in a data base. ,
A final legal issue is the expectations of the judge or jury regarding the presence of forensic evidence, particularly in cases of sexual assault involving children. The limited clinical data on young children suggest they have significantly lower rates of sperm or seminal fluid identification from forensic examination than adults, and there is little data on DNA evidence in children. Popular media suggests forensic evidence is everywhere and forensic evidence is essential, raising inappropriate expectations in juries hearing cases involving children.
Strength of the Medical Evidence
Eyewitness testimony was a factor in three quarters of cases involving 158 previously convicted individuals proven innocent using postconviction DNA testing. About two thirds of these convictions were also related to some misapplication of forensic science, with 40% of the convictions related in part to identification using conventional serology and about 20% related in part to hair comparisons. The transfer of trace evidence including hair, fibers, or foreign materials can suggest possible or probable association but usually provide only weak evidence for the identity of the perpetrator. Identification evidence, primarily body fluids, can provide stronger evidence of sexual acts and the identity of the perpetrator. The presence of sperm, acid phosphatase, PSA, and screening tests for saliva and blood are helpful in supporting the history of sexual contact and in identifying possible substances to submit for DNA analysis. Conventional serology can play a limited role in identity, but it is more helpful in excluding possible suspects than confirming actual perpetrators. DNA analysis provides a more sensitive and more specific approach to the identity of the offender than conventional serology, seminal fluid markers, and trace evidence testing, but there is little published data about the performance of DNA analysis in child and adolescent sexual assault cases.
Suggested Directions for Future Research
Two major areas of research are anticipated: one basic science and one clinical in nature. The first area will focus on improvements in DNA technology with increased use of Y chromosomal STR loci, mtDNA loci, SNP loci, and phenotypic DNA profiling. , This research will involve both improving the specificity of these techniques and improving speed and automation using biochemical tools including DNA microarrays, miniaturization, and “expert systems.” , A major clinical area of research is the utility of forensic evidence collection in both adults and children on obtaining usable DNA materials. There have been no large studies of DNA evidence in sexual assault victims of any age. Studies are needed on the effect of evidence collection methods used in adults on DNA analysis results. In addition, prepubertal children should be studied to determine whether the time limits and techniques for evidence collection used for adults and adolescents are valid in children, or whether modifications are needed in the forensic evidence protocols for children.
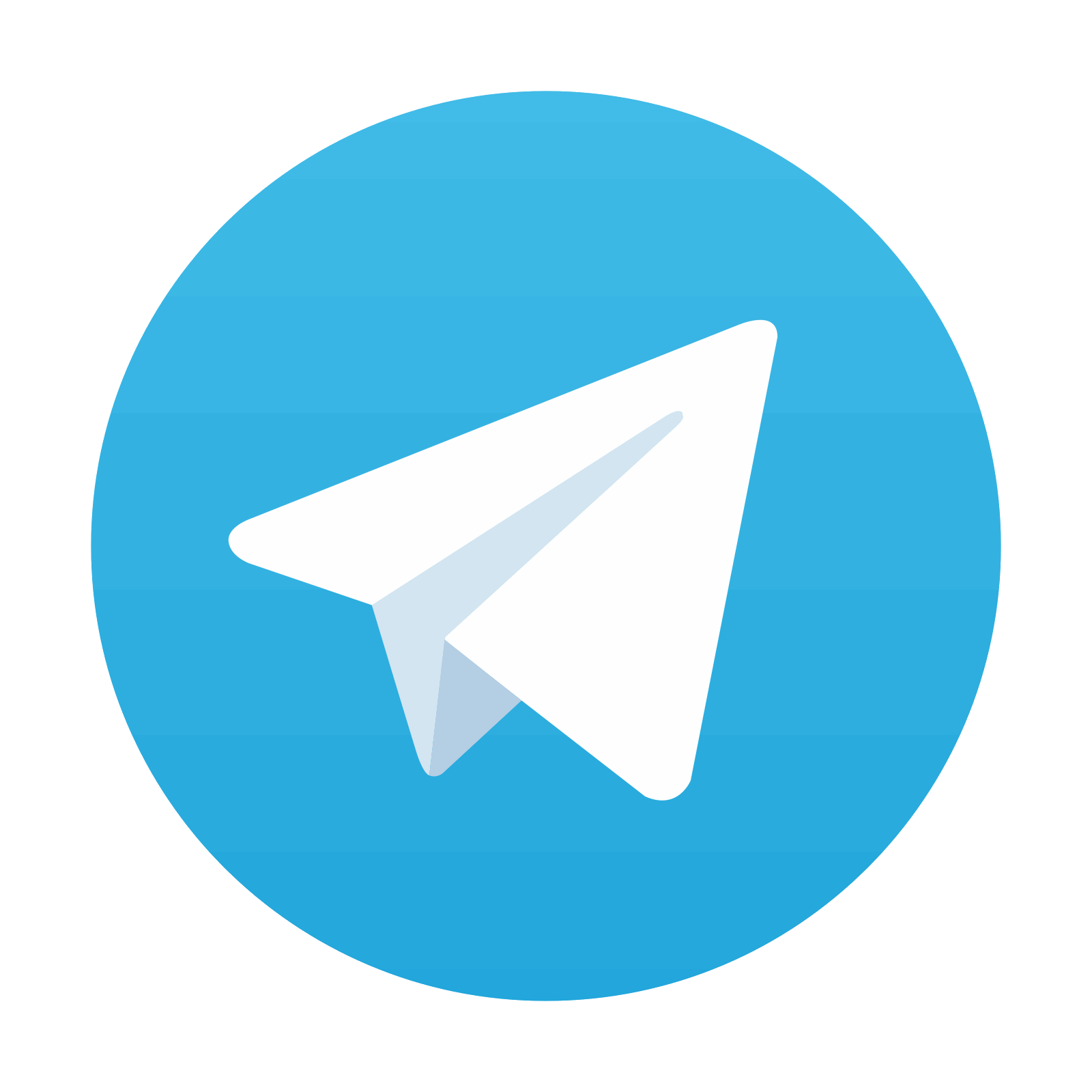
Stay updated, free articles. Join our Telegram channel

Full access? Get Clinical Tree
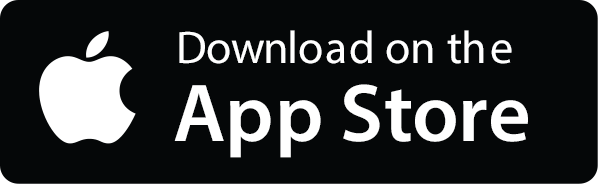
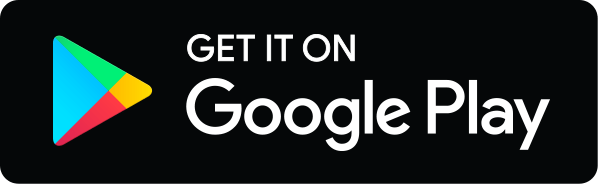