Background
Telomeres are nucleoprotein structures, essential for chromosome stability and cell survival. Telomeres are progressively shortened with each cell division and by environmental factors. Telomere loss has been linked to age and stress-induced premature senescence. Dysfunctional telomeres tend to form aggregates, which consist of the end-to-end fusion of telomeres. Telomere elongation is carried out by telomerase, which is a specific reverse transcriptase capable of adding telomeric repeats to chromosome termini. The TERC gene encodes the RNA template of the telomerase. Another compensatory mechanism that is enhanced in response to telomere shortening and senescence is the telomere capture (TC). Telomere shortening and elevated aggregate formation have been observed in trophoblasts from pregnancies complicated with preeclampsia (PE).
Objective
We opted to study mechanisms of telomere shortening in trophoblasts from pregnancies complicated with PE and to assess telomere length and homeostasis in fetal cord blood cells from PE pregnancies.
Study Design
Placental specimens and cord blood samples from uncomplicated pregnancies and from pregnancies complicated with PE were collected. Staining with 4′,6-diamidino-2-phenylindole was used to assess nuclear fragmentation: senescence-associated heterochromatin foci (SAHF). Fluorescence in situ hybridization was used to evaluate TERC gene copy number and TC. Telomere length and aggregate formation were assessed in cord blood using quantitative fluorescence in situ hybridization. Nonparametric Kruskal-Wallis and Mann-Whitney U tests were applied to test the differences between the study groups.
Results
Nine samples from pregnant patients with PE without intrauterine growth restriction and 14 samples from uncomplicated pregnancies that served as controls were collected. In cord blood cells, no differences were observed in telomere length, aggregate formation, TERC copy number, TC, or SAHF between PE and controls. In PE trophoblasts the percentage of cells with SAHF was higher in PE trophoblasts compared to controls (56.8 SD = 10.5% vs 35.2 SD = 10.7%, P = .028). The percentage of cells with abnormal TERC copy number was increased in PE trophoblasts compared to controls (31 ± 3.6% vs 12.97 SD = 5%, P = .004) as well as the percentage of cells with TC (27.4 SD = 9.4% vs 16 SD = 4.67%, P = .028).
Conclusion
We suggest that telomere shortening in PE trophoblasts is linked to cellular increased senescence. Alterations in telomere homeostasis mechanisms are present in such cases. These findings support the role of telomeres in the pathogenesis of trophoblastic dysfunction in PE. The lack of telomere shortening, modified telomere homeostasis mechanisms, and increased senescence in cord blood from pregnancies complicated with PE suggests that these processes are probably restricted primarily to the placenta.
Introduction
Telomeres are nucleoprotein structures, essential for chromosome stability and cell survival. Telomeres are progressively shortened with each cell division and by environmental factors. Telomere elongation is carried out by telomerase, which is a specific reverse transcriptase capable of adding telomeric repeats to chromosome termini. The TERC gene located on the human chromosome band at 3q26.3 encodes the RNA template of the telomerase. In normal human somatic cells, telomerase activity is undetectable or present at low levels. In conditions in which telomere shortening occurs, such as in cancer cells, telomerase is sometimes expressed at substantially higher levels. This mechanism attenuates telomere shortening and provides cells with a growth advantage. In addition, when telomere shortening occurs, a repair mechanism known as telomere capture (TC) can take place, through which a shortened telomere acquires a new telomeric sequence from a sister chromatid or from another chromosomal end.
Accelerated telomere shortening is caused by different physiological and pathological environmental factors, including oxidative stress. Telomeres are considered a biomarker for aging and age-related diseases, which have been linked to telomere loss and age and stress-induced premature senescence. Nuclei in senescent human cells are often granulated, containing senescence-associated heterochromatin foci (SAHF). Additionally, dysfunctional telomeres tend to form aggregates, which consist of the end-to-end fusion of telomeres, independent of telomere size or telomerase activity.
Altered placentation and relative placental hypoxia have a major component in the pathophysiology of preeclampsia (PE). Previous studies have shown that alterations in telomere homeostasis are present in conditions in which the placenta has a major pathophysiological role, such as PE and in pregnancies with intrauterine growth restriction (IUGR). While IUGR can be present in pregnancies complicated with PE, it is not clear whether IUGR in these cases reflects a more severe form in the spectrum of PE, or whether these are 2 different, coexisting conditions that are related to each other. Shorter telomeres were found in PE as well as in IUGR trophoblasts, and increased levels of telomere aggregates were observed in PE placentas. Also, decreased expression of human telomerase reverse-transcriptase, which is a component of telomerase, was found in PE and IUGR trophoblasts compared to controls. Other observations regarding the role of telomere homeostasis in the pathogenesis of placenta-related conditions have been shown in IUGR placentas. A decreased TERC copy number was observed in IUGR placentas by Biron-Shental et al, but not by Toutain et al. Undetected telomerase activity in IUGR pregnancies was reported by Izutsu et al. In addition, an increase in SAHF and increased TC were observed in IUGR placentas, as well as decreased human telomerase reverse-transcriptase messenger RNA levels.
The aims of this study were to assess telomere length and aggregate formation in umbilical cord blood cells collected from pregnancies complicated with PE, to investigate mechanisms of telomere homeostasis, and to evaluate SAHF in trophoblasts from PE placentas and in cord blood cells.
Materials and Methods
Study groups and sample collection
The study was approved by the institutional ethics review board and all patients provided signed informed consent. Biopsies from placentas and cord blood samples from third-trimester pregnancies were examined. All samples were collected in the delivery room of Meir Medical Center in Kfar Saba, Israel. Placental samples were taken from the midpoint between the insertion of the cord and the placental margin. Nine samples from pregnant patients with PE without IUGR and 14 samples from uncomplicated pregnancies that served as controls were collected. PE was defined as blood pressures >140/90 mm Hg and 24-hour proteinuria >300 mg. Seven of 9 cases in the PE group had severe features: 2 with blood pressure >160/110 mm Hg; 3 with HELLP syndrome (hemolysis, elevated liver enzymes, low platelet count); and 2 with both blood pressure >160/110 mm Hg and elevated liver enzymes (above twice the upper normal limit). Our study and control groups consisted of Caucasian patients only.
Specimens from pregnancies complicated with chorioamnionitis or with thick meconium were excluded. Placental biopsies were collected within 20 minutes of delivery and frozen at –80°C. Cord blood samples were cultured and preserved at 4°C. Telomere length and aggregate formation were performed in cord blood cells. Fluorescence in situ hybridization (FISH) for TERC copy number and for TC and SAHF were evaluated both in trophoblasts as well as in cord blood cells. FISH was done on 4-μm sections of placental tissue samples and on slides with cultured cord blood cells.
FISH for telomere length
PNA FISH Kit/Cy3 kit (Dako, Glostrup, Denmark) was used. Slides were incubated for 2 minutes in TRIS-buffered saline (TBS) followed by fixation in formamide 3.7% diluted 1:10 in phosphate-buffered saline (PBS) for 2 minutes. Slides were placed in TBS for 5 minutes twice, in pretreatment solution for 10 minutes, in TBS solution for 5 minutes twice, dehydrated in a graded ethanol series, and left to air dry. This was followed by placement of 10 μL of CY3-labeled telomere-specific peptide nucleic acid probe (vial 2 in K 532; Dako) and hybridization at 80°C for 5 minutes. Slides were then placed at room temperature for 30 minutes in a dark environment followed by placement in rinse solution for 1 minute, in wash solution at 65°C for 5 minutes, and dehydration in a graded ethanol series. Slides were counter-stained in 4′,6-diamidino-2-phenylindole (DAPI)-II (Abbott) antifade solution and analyzed for simultaneous viewing of fluorescein isothiocyanate, Texas red, and DAPI with an imaging processing system (Applied Spectral Imaging, Migdal Haemek, Israel).
Analysis of telomere length and aggregates
The slides were scored according to signal intensity and telomere number. The cells were categorized as having high (strong) or low (weak) fluorescence, and by number of telomere signals (dots per cell): <10, 10-30, or >30 ( Figure 1 ). Short telomeres are consistent with fewer signals and lower intensity signals, while long telomeres with more signals, with higher intensity. In previous studies performed in our laboratory, validation of FISH manual counts was done by computer-assisted analysis of digital images. We calculated the percentage of cells with telomere aggregates.
FISH for TERC and for TC
We used a Kreatec probe Cat. No. KBI-10110 hTERC (3q26) (red)/3q11 (green). The hTERC (3q26)-specific DNA probe was optimized to detect copy numbers of the human telomerase RNA gene ( hTERC ) at region 3q26. The 3q11 region probe was included to facilitate chromosome identification. The probes used for TC were SNRPN and 13q 14.3: orange, the “normal disomic loci” and their subtelomeric regions (15qter or 13qter: green).
Slides were placed in a plastic jar containing 40 mL of fixative solution (acetic acid and ethanol at a ratio of 1:3) for 5 minutes twice and allowed to air dry. Slides were then incubated for 10 minutes in 2 × standard saline citrate at 37°C, fixated in formaldehyde for 15 minutes, washed in PBS for 5 minutes, and incubated in pepsin solution (75 g lyophilized pepsin dissolved in 50 mL of 0.01N HCl), followed by PBS wash for 5 minutes, then dehydrated in a graded ethanol series and dried on a hotplate at 40°C for 3 minutes. The probe mix was applied for denaturation at 73°C for 5 minutes and hybridized for 18 hours at 37°C in a moist chamber. Following hybridization, the slides were washed in 0.4 standard saline citrate/0.3NP 40 for 2 minutes at 72°C, followed by a wash in 2 SSC/0.1NP 40 for 1 minutes at room temperature. Slides were counter-stained with DAPI II antifade solution. We used ×1000 magnification on BX50 Olympus microscope.
Analysis of SAHF
The percentage of fragmented DAPI-stained nuclei was evaluated in placental samples and cord blood samples.
Analysis of TERC copy number
Since most cells were in interphase, the analysis was performed on interphase nuclei and on a few cells that were in metaphase. We compared the number of signals of the probe targeted to the specific loci of TERC gene (orange) and the number of signals from the control probe (green). Cells containing >2 copies of TERC gene (orange signal) were considered amplified. We calculated the percentage of cells with abnormal signals. Although some of the TERC amplification could have arisen from background noise or from nonspecific binding, and TERC copy loss could be a technical issue, the same phenomenon probably occurred in both the study and the control group and should not affect the results.
We compared the average percentage of cells with TERC amplification between the study and control groups.
Analysis of TC
In the nuclei, the number of the specific loci of the specific chromosome (SNRPN or 13q 14.3: orange, the normal disomic loci) and its telomere (15qter or 13qter: green) were compared. The number of signals of the SNRPN or 13q 14.3 locus was compared to the number of signals of the subtelomeric region of the specific chromosome (green signals). For example, the normal appearance is 2 orange and 2 green signals, while an abnormal appearance, which represents TC, is 2 orange compared with 1, 3, or more green signals (the rearranged-captured subtelomeric regions), previously described by Amiel et al.
Only cells in which 2 orange signals (normal disomic loci) appeared were analyzed. Positive and negative controls were used to ensure the reliability of the assay. We compared the average percentage of cells with TC amplification between the study and control groups.
Statistical analysis
Nonparametric Kruskal-Wallis and Mann-Whitney U tests were applied to test the differences between the study groups, with P < .05 considered statistically significant. Software (SPSS; IBM Corp, Armonk, NY) was used for statistical analysis.
Materials and Methods
Study groups and sample collection
The study was approved by the institutional ethics review board and all patients provided signed informed consent. Biopsies from placentas and cord blood samples from third-trimester pregnancies were examined. All samples were collected in the delivery room of Meir Medical Center in Kfar Saba, Israel. Placental samples were taken from the midpoint between the insertion of the cord and the placental margin. Nine samples from pregnant patients with PE without IUGR and 14 samples from uncomplicated pregnancies that served as controls were collected. PE was defined as blood pressures >140/90 mm Hg and 24-hour proteinuria >300 mg. Seven of 9 cases in the PE group had severe features: 2 with blood pressure >160/110 mm Hg; 3 with HELLP syndrome (hemolysis, elevated liver enzymes, low platelet count); and 2 with both blood pressure >160/110 mm Hg and elevated liver enzymes (above twice the upper normal limit). Our study and control groups consisted of Caucasian patients only.
Specimens from pregnancies complicated with chorioamnionitis or with thick meconium were excluded. Placental biopsies were collected within 20 minutes of delivery and frozen at –80°C. Cord blood samples were cultured and preserved at 4°C. Telomere length and aggregate formation were performed in cord blood cells. Fluorescence in situ hybridization (FISH) for TERC copy number and for TC and SAHF were evaluated both in trophoblasts as well as in cord blood cells. FISH was done on 4-μm sections of placental tissue samples and on slides with cultured cord blood cells.
FISH for telomere length
PNA FISH Kit/Cy3 kit (Dako, Glostrup, Denmark) was used. Slides were incubated for 2 minutes in TRIS-buffered saline (TBS) followed by fixation in formamide 3.7% diluted 1:10 in phosphate-buffered saline (PBS) for 2 minutes. Slides were placed in TBS for 5 minutes twice, in pretreatment solution for 10 minutes, in TBS solution for 5 minutes twice, dehydrated in a graded ethanol series, and left to air dry. This was followed by placement of 10 μL of CY3-labeled telomere-specific peptide nucleic acid probe (vial 2 in K 532; Dako) and hybridization at 80°C for 5 minutes. Slides were then placed at room temperature for 30 minutes in a dark environment followed by placement in rinse solution for 1 minute, in wash solution at 65°C for 5 minutes, and dehydration in a graded ethanol series. Slides were counter-stained in 4′,6-diamidino-2-phenylindole (DAPI)-II (Abbott) antifade solution and analyzed for simultaneous viewing of fluorescein isothiocyanate, Texas red, and DAPI with an imaging processing system (Applied Spectral Imaging, Migdal Haemek, Israel).
Analysis of telomere length and aggregates
The slides were scored according to signal intensity and telomere number. The cells were categorized as having high (strong) or low (weak) fluorescence, and by number of telomere signals (dots per cell): <10, 10-30, or >30 ( Figure 1 ). Short telomeres are consistent with fewer signals and lower intensity signals, while long telomeres with more signals, with higher intensity. In previous studies performed in our laboratory, validation of FISH manual counts was done by computer-assisted analysis of digital images. We calculated the percentage of cells with telomere aggregates.
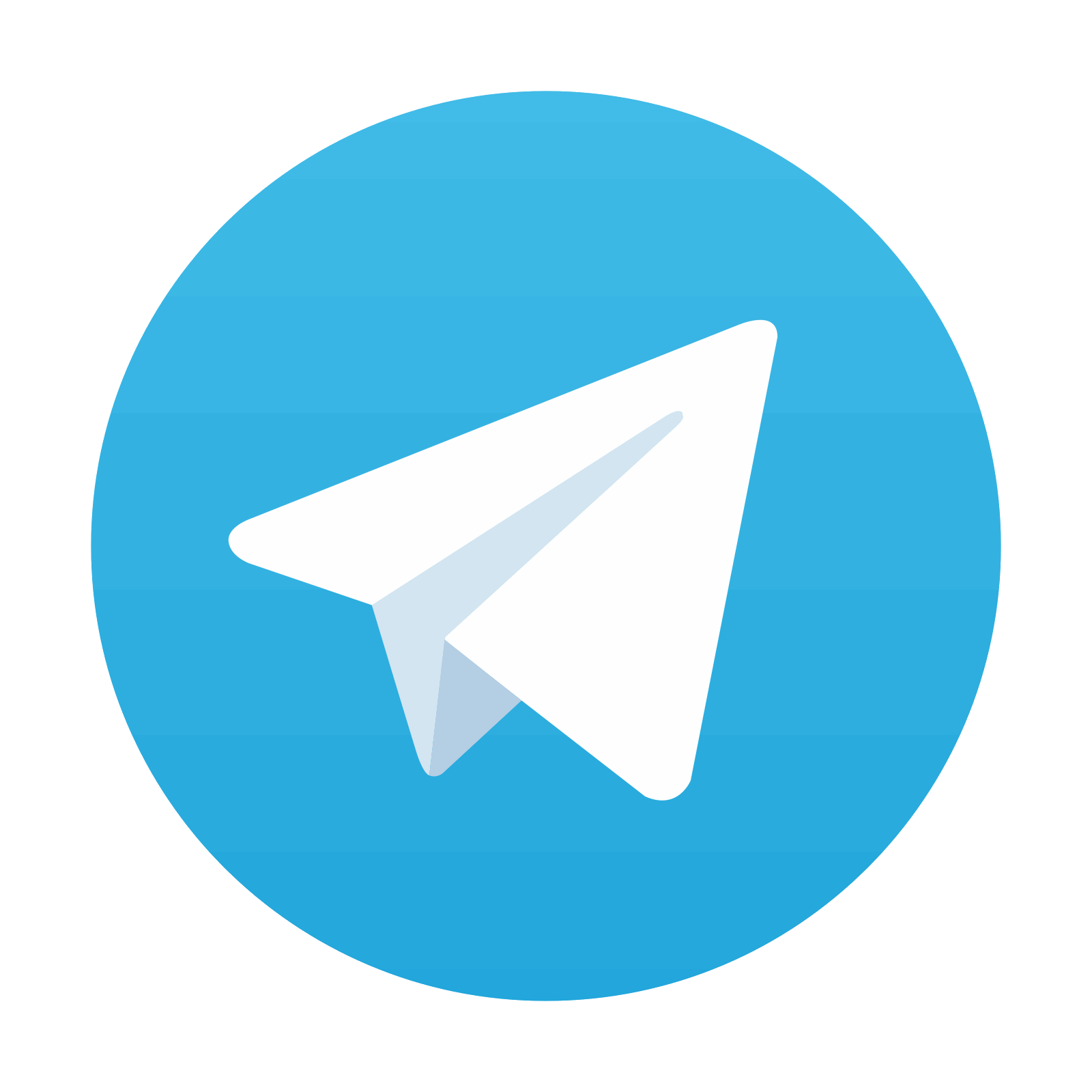
Stay updated, free articles. Join our Telegram channel

Full access? Get Clinical Tree
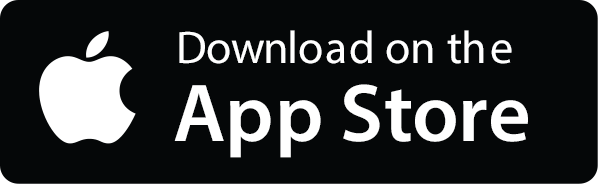
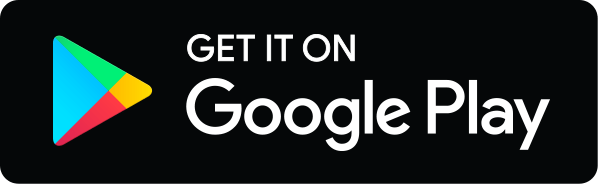
