INTRODUCTION AND DEFINITION
West syndrome (WS) is a devastating form of epilepsy defined by a triad of infantile spasms, hypsarhythmia, and developmental regression (1,2). The clinical features of spasms that occur in clusters were first poignantly described in 1841 by Dr. West of England of his infant son (3). More than 110 years later, in 1952, the characteristic EEG pattern of WS, hypsarhythmia, was described in Chicago (in honor of the Gibbs’ preference, the original Chicago spelling of hypsarhythmia is used) (1). Early studies have recognized that the most serious aspect of WS was the poor developmental outcome (4,5,6): 70% to 90% of children subsequently develop cognitive deficit (IQ<70, in most IQ 30–50 = moderate to severe mental retardation) and 30% show symptoms of autistic spectrum disorder. Unfortunately, many infants with WS also develop focal seizures later or evolve into another form of catastrophic epileptic encephalopathy, Lennox–Gastaut syndrome (7). Overall, a grave prognosis in WS is often associated with delay in treatment and the specific underlying etiology (5,7,8). For a great majority (70%) of infants who present with spasms, the cause for WS can be determined and includes genetic (tuberous sclerosis complex), structural (malformation of cortical development), and previous CNS insult (7,9,10). In 1958, the first therapeutic breakthrough was reported by Sorel and Dusaucy-Bauloye (11), who observed improvement in EEGs and amelioration of spasms in patients treated with adrenocorticotropic hormone (ACTH).
The original clinical description by Dr. West and the first description of the associated EEG pattern by Drs. Gibbs and Gibbs have stood the test of time and are relatively unchanged even now. However, our understanding of the clinical manifestations of this disorder was greatly increased by the development of long-term polygraphic-video monitoring techniques in the 1970s (12). These techniques also provided objective means of evaluating the acute effects of therapy on seizure frequency and the EEG that have proven useful for clinical trials. The introduction of computed tomography (CT), magnetic resonance imaging (MRI), and positron emission tomography (PET) scanning in the 1970s and 1980s greatly increased our understanding of associated brain abnormalities seen in these patients. Equally, if not more, important is the recent introduction of widely available genetic testing, which has not only been helpful in the classification of patients but has also aided our understanding of the possible pathophysiological mechanism(s) underlying this condition.
In this chapter, the clinical and EEG features of WS are described and the current understanding of pathophysiology and optimal therapy is reviewed. This is a revision of the excellent chapter originally produced by Drs Hrachovy and Frost for the third edition of this textbook, with notable updates, including new animal models and clinical genetic testing that has increased our understanding of the pathogenesis of WS, and the FDA approval of natural ACTH and vigabatrin for the treatment of infantile spasms in the United States.
EPIDEMIOLOGY
WS affects infants between 6 and 12 months old (peak onset of 4–6 months old) and accounts for as much as 5% of all childhood-onset epilepsy and up to 20% of new-onset epilepsy cases in children under 2 years of age (9,13). The average reported incidence of infantile spasms is approximately 0.31 (range 0.05–0.60) per 1,000 live births (14,15).
CLINICAL MANIFESTATIONS
Description of Spasms
The motor spasm typically consists of a brief, bilaterally symmetrical contraction of the muscles of the neck, trunk, and extremities. This muscle activity is typically characterized by an initial phasic contraction lasting less than 2 seconds, which may be followed by a less intense, but more sustained, tonic contraction lasting up to 10 seconds in duration. The exact character of the seizure depends on whether the flexor or extensor muscles are predominantly affected and on the distribution of the muscle groups involved (12,16). The position of the body (e.g., supine versus sitting) usually influences the type of spasm. The intensity of the spasm may vary from a massive contraction of all flexor muscles, resulting in a jack-knife at the waist, to a minimal contraction of muscles such as the abdominal recti.
Kellaway et al (16) utilized polygraphic-video EEG monitoring to analyze more than 5,000 infantile spasms recorded from 24 infants. They found that mixed spasms were the most frequent (approximately 42%), followed by flexor spasms (approximately 34%); extensor spasms were the least common (approximately 25%). Asymmetrical spasms were rare (less than 1%). Clusters of spasms occurred most commonly after an arousal from sleep. Periods of attenuated responsiveness, which have been termed arrest phenomena, may occur following a motor spasm or may occur independently. Most infants with this disorder have more than one type of spasm (16,17).
A variety of clinical phenomena have been reported to occur in association with the motor spasms. These include autonomic changes (heart rate alterations, cyanosis, pallor, sweating, and flushing), respiratory rate changes, vocalizations (crying, laughter, and grunting sounds), hiccups, smiling, grimacing, tongue and mouth movements, and ocular events (eye deviation, nystagmus, eye opening or closing, pupillary dilation, and tearing).
Monitoring studies have shown that there is little variation in the number of spasms recorded from the same patient in consecutive 24-hour monitoring periods; however, there is a marked variation in spasm frequency when patients are monitored at 2-week intervals (18). The number of spasms recorded during 24-hour periods has ranged from a handful to several hundred in different patients (16, 17).
Approximately the same number of spasms occurs during the day as at night; however, the spasms rarely occur when the infant is actually asleep (less than 3%) (19–21). Instead, they frequently occur immediately upon, or soon after, arousal (16,17,22–25). The spasms are not precipitated by feeding or photic stimulation, but may occasionally be elicited by tactile stimulation or unexpected loud noises, though this is uncommon (16).
Although spasms may occur in an isolated fashion, they frequently occur in clusters, with clusters being reported in 47% to 84% of cases (16,17,21,26,27). In the series reported by Kellaway et al (16), the number of spasms per cluster varied from 2 to 138. Succession rates of up to 15 spasms per minute were recorded, and the intensity of the motor spasms within a cluster would usually wax and then wane.
Spontaneous Remission
The phenomenon of spontaneous remission of infantile spasms is poorly understood. Published data concerning the duration of this disorder have been infrequent and imprecise. In 1973, Jeavons et al (28) reported that 28% of patients were free of spasms by 1 year of age, 49% before age 2, 65% before age 3, and 74% by age 4. Unfortunately, some of their patients had been treated with steroids, and EEG findings were not presented. To further investigate spontaneous remission in this disorder, Hrachovy et al. retrospectively studied 44 patients who had not been treated with hormonal drugs (29). The results indicated that spontaneous remission of spasms and disappearance of the hypsarhythmic EEG pattern can begin within 1 month of the onset of this disorder and that 25% of patients with infantile spasms experience spontaneous remission within 1 year. This phenomenon must always be remembered when interpreting results of any therapeutic trial in this disorder, and must also be considered in discussing possible pathophysiologic mechanisms underlying infantile spasms. Also bear in mind that parents underestimate the number of spasms and that some are so subtle that they may not be recognized without careful analysis of polygraphic-video EEG monitoring.
Coupling of Spasms With Other Seizures
Using concurrent polygraphic recordings can help differentiate epileptic spasms from other seizures that appear similar. Surface EMG (usually placed over the right shoulder and posterior neck muscles) signatures differ significantly for spasms (rhomboid-shape), myoclonia (single spike-shape), tonic (prolonged rectangular-shape), and myoclonic–tonic (combination of a single spike followed by prolonged rectangular-shape).
Focal seizures may appear before the onset of spasms, concurrently with spasms, or after spasms have ceased, either spontaneously or following treatment (22,23,30–33). In 1984, Hrachovy et al first described the association between focal epileptiform discharges and infantile spasms (34), and since that time several additional reports of this phenomenon in small groups of patients have been published (35–40). Although this is an interesting phenomenon, its significance remains uncertain. These observations have been used by some investigators to support the hypothesis that infantile spasms occur as a result of an interaction between a primary cortical generator and subcortical structures (see subsequent section) (41).
ELECTROENCEPHALOGRAPHIC FEATURES
Interictal Patterns
The interictal pattern most commonly associated with infantile spasms is hypsarhythmia (Figure 30.1), which was originally defined by Drs. Gibbs and Gibbs (42) as follows:
FIGURE 30.1 Hypsarhythmia (digital recording from a 6-month-old male).
random high voltage slow waves and spikes. These spikes vary from moment to moment, both in duration and in location. At times they appear to be focal, and a few seconds later they seem to originate from multiple foci. Occasionally the spike discharge becomes generalized, but it never appears as a rhythmically repetitive and highly organized pattern that could be confused with a discharge of the petit mal or petit mal variant type. The abnormality is almost continuous, and in most cases it shows as clearly in the waking as in the sleeping record.
This prototypic pattern is usually seen in the early stages of the disorder and most often in younger infants (younger than 1 year of age). The pattern has been reported in 7% to 75% of patients with infantile spasms (22,23,43–47). In addition, variations or modifications of this pattern may be seen in many patients. In 1984, Hrachovy et al identified five variations of the originally described pattern after reviewing the 24-hour EEG-video monitoring studies in 67 infants with infantile spasms (34). These variations include hypsarhythmia with a consistent focus of abnormal discharge, hypsarhythmia with increased interhemispheric synchronization, hypsarhythmia comprising primarily high-voltage, slow-wave activity with very little spike or sharp wave activity, asymmetrical or unilateral hypsarhythmia, and hypsarhythmia with episodes of generalized, regional, or localized voltage attenuation, which, in its maximal expression, is referred to as the “suppression-burst variant.” These variations were subsequently confirmed by Alva-Moncayo et al (43) in 100 cases.
In addition to demonstrating these basic variations, 24-hour EEG-video monitoring studies have shown that hypsarhythmia is a highly dynamic pattern, with transient alterations in the pattern occurring throughout the day. The hypsarhythmic activity tends to be most pronounced and to persist to the latest age in slow-wave (non-rapid-eye movement [NREM]) sleep. During NREM sleep there is a tendency for grouping of the multifocal spike and sharp wave discharges and/or frequent brief epochs of attenuation resulting in a quasi-periodic, or discontinuous, appearance of the background activity (34,48,49). The hypsarhythmic pattern is least evident or completely absent during REM sleep, when the background activity may appear normal (48). Transient disappearance or reduction of the hypsarhythmic activity is usually seen upon arousal from sleep or during a cluster of spasms (16).
Although hypsarhythmia or one of its variants is most commonly seen in patients with infantile spasms, several other interictal patterns may occur (14,31). These include diffuse slowing of the background activity, focal slowing, focal or multifocal spikes and sharp waves, generalized slow-spike-and-slow-wave activity, focal depression, paroxysmal slow or fast bursts, or continuous spindling. These patterns may occur in isolation or in various combinations. In a small number of infants, the background activity may appear normal.
Ictal Patterns
A variety of ictal EEG patterns have been identified (16). These include generalized slow-wave transients, sharp-and-slow-wave transients, and attenuation episodes, occurring alone or with superimposed faster frequencies. These patterns occur singly or in various combinations. However, the most common ictal EEG change is a generalized slow-wave transient, followed by an abrupt attenuation of background activity in all regions (Figure 30.2). The duration of the ictal EEG event may range from less than 1 second to more than 1 minute, with the longer episodes being associated with arrest phenomena. Also, episodes of generalized voltage attenuation may occur in the absence of clinical spasms. These observations have been confirmed by many authors (17,38,50–61). There is no close correlation between the character of the ictal EEG event and the type of spasm, with the exception that an asymmetric ictal pattern usually correlates with focal or lateralized brain lesions (53,62).
PATHOPHYSIOLOGY
The pathophysiologic mechanism underlying infantile spasms is not known. However, suitable animal models exhibiting the major clinical and electroencephalographic features of this disorder have recently been developed, adding to our knowledge. This brief overview describes some of the hypotheses that have been proposed, but cannot be considered comprehensive given the complexity and quickly evolving nature of the subject.
Considerable evidence implicates the brainstem as the area in which epileptic spasms and the hypsarhythmic EEG pattern originate (48,63–67). Hravochy et al previously described a pathophysiologic model of infantile spasms, based on long-term polygraphic-video monitoring experience (48,68), which suggested that dysfunction of certain monoaminergic or cholinergic regions of the brainstem involved in the control of sleep cycling may be responsible for the generation of the spasms and the EEG changes seen in this disorder (68). According to this model, the clinical spasms would result from phasic interference of descending brainstem pathways that control spinal reflex activity, whereas the hypsarhythmic EEG pattern, and perhaps the cognitive dysfunction seen in these patients, would result from activity occurring in ascending pathways projecting from these brainstem regions to the cerebral cortex. Various other investigators have also suggested that dysfunction of monoaminergic neurotransmitter systems may be responsible for the generation of epileptic spasms (69–74). It has also been reported that corticosteroids (71) and ACTH (75) suppress central serotonergic activity, a finding consistent with this brainstem hypothesis. However, this model did not exclude the possibility that these critical brainstem region(s) might be affected by distant sites, because the brainstem sleep system receives input from many other areas (68). Several years later, Chugani et al (66,76) expanded this hypothesis. Primarily on the basis of PET scan studies, these authors suggested that the brainstem dysfunction causing infantile spasms was produced by an abnormal functional interaction between the brainstem (raphe nuclei) and a focal or diffuse cortical abnormality. According to this hypothesis, the cortical abnormality exerts a noxious influence over the brainstem from where the discharges spread caudally and rostrally to produce spasms and the hypsarhythmic EEG pattern. The association of focal seizures with infantile spasms (described previously) was further evidence used to support the hypothesis that a primary cortical generator interacts with subcortical structures, resulting in infantile spasms. This model provides for the observation that a subset of infantile spasm patients with localized lesions in the cortex may have cessation of seizures and improved EEGs after resection of focal cortical lesions (76–79). A similar model proposing that spasms arise from subcortical structures was provided by Dulac et al (80). This group hypothesized that the epileptic spasms result from a functional deafferentation of subcortical structures such as the basal ganglia caused by abnormal cortical activity, but the hypsarhythmic EEG pattern directly reflects the cortical dysfunction. A cortical-subcortical interaction was also postulated by Avanzini et al (81) and Lado and Moshe (82).
FIGURE 30.2 Digital recording from a 6-month-old male showing ictal EEG change associated with infantile spasms. Note the period of voltage attenuation associated with superimposed fast activity.
Another major hypothesis is that infantile spasms are the result of a defect in the immunologic system (68,83,84). Supportive evidence for this hypothesis includes the presence of antibodies to extracts of normal brain tissue in the sera of patients with infantile spasms (85,86), the presence of increased numbers of activated B cells and T cells in the peripheral blood of patients with infantile spasms (87), and abnormal leukocyte antigen studies in patients with infantile spasms compared with control subjects (88–90). Although these findings indicate abnormal immune function in patients with infantile spasms, there is no direct evidence that an immunologic defect causes this disorder.
Another hypothesis is that corticotropin-releasing hormone (CRH) may play a mechanistic role in infantile spasms (91–93). According to this model, stress or injury during early infancy results in the release of excess amounts of CRH, which in the presence of an abundance of CRH receptors produces epileptogenic alterations in the brainstem pathways that result in spasms. The therapeutic benefit of corticosteroids and ACTH in this disorder would be secondary to the suppression of CRH synthesis by these hormones. However, although injection of CRH into the brains of infant rodents does produce seizures, the ictal behaviors and EEG features are not typical of those seen in the human condition (94). In addition, CRH levels are not elevated in the cerebrospinal fluid (CSF) of patients with infantile spasms (92). Furthermore, treatment of patients with infantile spasms with a competitive antagonist of CRH did not alter spasm frequency or significantly change the EEG pattern (95).
Several additional pathophysiologic mechanisms underlying this disorder have also been proposed. It has been suggested that infantile spasm results from a failure or delay of normal developmental processes (96). This theory is based largely on the assumption that ACTH and corticosteroids accelerate certain normal developmental processes in immature animals (97–101).
Also, several biochemical and metabolic disturbances have been reported in patients with infantile spasms. These include dysfunction of metabolic pathways for neuropeptides, pyridoxine, and amino acids, such as aspirate, glutamate, and gamma-aminobutyric acid (14).
There are multiple genetically based conditions associated with infantile spasms (14), some of which involve the same region of the X chromosome. For example, Aicardi syndrome has been associated with X chromosome abnormalities near Xp22 (102). Patients with incontinentia pigmenti type I have abnormalities in the same region, with evidence of X/autosomal trans-location at Xp11 (103). Patients with X-linked infantile spasms have mutations involving the ARX gene located on the X chromosome at Xp22 (104–108) and the CDKL5 (STK9) gene (109–115). Pyruvate dehydrogenase complex deficiency, a metabolic defect associated with infantile spasms, has been localized to Xp22.1–Xp22.2, a region similar to that associated with X-linked infantile spasms (116). These findings suggest that defects in the involved region of the X chromosome and products of the involved gene (or genes) may play a role in the pathophysiology of this disorder (14,107,117). Animal models of infantile spasms involving mutations in ARX, a transcription factor involved in interneuron development, were recently developed and will likely not only shed light on the pathogenesis of West syndrome, but also lead to new treatments (118).
In 2005, Frost and Hrachovy proposed an updated model concerning the pathophysiology of this disorder based on developmental desynchronization (119). According to this model, infantile spasms result from a particular temporal desynchronization of two or more developmental processes, resulting in a specific disturbance of brain function. As shown in Figure 30.3, the developmental desynchronization could be produced by (1) a mutation or inherited abnormality affecting the primary genes governing ontogenesis, (2) a mutation or inherited abnormality affecting the genes specifying transcription factors (or other genetic modulators), or (3) an injurious external environmental factor affecting the maturational processes of brain tissues, neurochemical systems, or both. Each mechanism (or combination of mechanisms) could be manifested at different locations and at different points of development. As a result, at least one developmental process would lag behind other processes, resulting in a loss of integration of brain function. This model accounts for the observation that multiple, seemingly unrelated, conditions and insults occurring at different points of development (prenatal, perinatal, or postnatal) could result in the same functional deficit. Also, this hypothetical model is consistent with the response of patients with infantile spasms to a diverse group of therapeutic agents with different modes of action. All agents would not be effective in all patients because of the different fundamental impairments responsible for the common functional deficit resulting in spasms. Also, the phenomenon of spontaneous remission could result from internal control mechanisms detecting the developmental desynchronization that caused spasms and responding to it by the activation or modulation of other gene regulatory systems. In support of this theory, there is an animal model of unilateral cortical damage utilizing tetrodotoxin (TTX) that creates a phenotype with seizures resembling infantile spasms and an EEG pattern similar to hypsarhythmia (120).
FIGURE 30.3 Developmental desynchronization model of infantile spasms pathogenesis, showing schematically the interaction of developmental processes controlled by primary genes (e.g., neurogenesis, myelination, synaptogenesis, apoptosis, neurotransmitter systems) (horizontal lines) with regulatory gene effects (vertical lines from bottom) and environmental factors (vertical lines from top). Vertical dashed lines indicate hypothetical maximal extent of desynchronization consistent with normal function at 6 months.
Source: From Ref. (117). Reprinted from Frost JD Jr., Hrachovy RA. Pathogenesis of infantile spasms: a model based on developmental desynchronization, J. Clin Neurophysiol. 2005;22:25–36. Figure 1, page 28; copyright 2005, with kind permission from Wolters Kluwer/Lippincott Williams & Wilkins.
DIAGNOSTIC EVALUATION AND TREATMENT
Diagnostic Evaluation
The diagnosis of infantile spasms is suggested on the basis of a good clinical history. Thorough general physical and neurologic examinations must be performed. This should include a careful ophthalmic evaluation and close examination of the skin using a Wood’s lamp to rule out such conditions as tuberous sclerosis. A routine EEG, recorded with the infant awake and asleep, is then obtained, which helps confirm the diagnosis. If the routine EEG does not reveal hypsarhythmia and if the typical ictal EEG patterns (described previously) or spasms are not recorded, a prolonged video-EEG monitoring study should be performed to establish the presence of the disorder. Neuroimaging studies, preferably MRI, should be obtained to search for structural brain abnormalities. Magnetic resonance spectroscopy (MRS) may be considered if inborn errors of metabolism are suspected. If ACTH or corticosteroids are to be started, the neuroimaging studies should be obtained before institution of such therapy, because these agents produce enlargement of CSF spaces that cannot be easily distinguished from preexisting cerebral atrophy. Routine laboratory studies including complete blood count with differential, renal panel with electrolytes and glucose, liver panel, serum calcium, magnesium, and phosphorus, and urinalysis should be obtained in all cases before institution of therapy. If an associated etiology is not identified on the basis of the previous information, the optimal next step is currently evolving with advances in genetic testing. In 2010, a patient would have a pyridoxine trial and a metabolic workup including serum lactate and pyruvate, plasma ammonia, urine organic acids, serum and urine amino acids, and serum biotinidase. In addition, the CSF would be evaluated for cell count, glucose, protein, viral and bacterial culture, lactate and pyruvate, amino acids, and neurotransmitters (121). Following the 2015 results of a recent large multicenter prospective study of the evaluation of new onset infantile spasms in the United States, an alternative approach following an unrevealing clinical and MR neuroimaging evaluation is to proceed with array comparative genomic hybridization (aCGH) next, and if the microarray is negative, then proceed with an epilepsy gene panel. The aforementioned metabolic testing would be the next appropriate step prior to consideration of whole exome sequencing (122).
Associated Etiological and Clinical Factors
In approximately 40% of patients, no associated etiological factor can be clearly identified. In the other 60%, various prenatal, perinatal, and postnatal factors have been implicated. In a review of the more than 400 published reports concerning etiology (14), more than 200 associated conditions were identified. These include such prenatal conditions as cerebral dysgenesis (e.g., lissencephaly), intrauterine infection, hypoxia–ischemia, prematurity, and genetic disorders (e.g., tuberous sclerosis); perinatal conditions such as traumatic delivery and hypoxia–ischemia; and postnatal conditions such as inborn errors of metabolism (e.g., nonketotic hyperglycinemia), head injury, central nervous system (CNS) infection, hypoxia–ischemia, and intracranial hemorrhage. Approximately 80% of patients with infantile spasms show some degree of mental and developmental retardation, and approximately the same percentage of patients have neurologic deficits (14). Diffuse and focal abnormalities on MRI and CT scans may be seen in 70% to 80% of cases. In addition, MRI may also reveal evidence of delayed myelination (123–125). PET reportedly detects focal or diffuse hypometabolic changes in up to 97% of patients with infantile spasms (126). However, these changes do not necessarily persist over time, suggesting that cortical hypometabolism in some patients with infantile spasms does not represent a structural lesion, but only a functional change (125,127,128).
In the past several years, the availability of genetic testing has changed the landscape of known etiologies for this disorder. One study of 44 children with infantile spasms of unknown cause used whole-exome sequencing and identified pathogenic mutations in multiple genes including STXBP1, CASK, ALG13, PNPO, and ADSL (129). In a prospective multicenter study of new-onset infantile spasms, the epilepsy gene panel resulted in an etiologic diagnosis in 31%; however, this was only performed in less than 25% of patients aCGH was diagnostic in 11% of the 55% of patients tested (122).
Patient Classification
In the past, the classification of infantile spasm patients was variable and inconsistent (14,31). Currently, patients are best classified on the basis of medical history, developmental history, neurologic examination, and MR neuroimaging studies. Based on these criteria, patients can be divided into two main groups: cryptogenic or symptomatic. Those with no abnormality on neurologic examination, no known associated etiological factor, normal development before onset of the spasms, and normal neuroimaging studies are categorized as cryptogenic. Currently, approximately 20% of patients with infantile spasms are classified as cryptogenic (14), with the remaining 80% classified as symptomatic. This classification scheme can be helpful in the management of these patients because patients in the cryptogenic category have the best prognosis for spasm control and long-term developmental outcome (see following discussion). However, it should be noted that the terms cryptogenic and symptomatic were replaced by “unknown” and “structural/metabolic/genetic,” respectively, in the 2010 proposed revised terminology and concepts for organization of seizures and epilepsies from the ILAE (130). Utilizing a more specific diagnosis based on new and future diagnostic modalities may prove even more helpful in the management of WS.
Differential Diagnosis
The diagnosis of infantile spasms is often delayed for weeks or months because parents, and even physicians, do not recognize the motor phenomena as seizures. Colic, Moro reflexes, and startle responses are diagnoses frequently made by pediatricians. Parents also may confuse infantile spasms with hypnagogic jerks occurring during sleep, head banging, transient flexor-extensor posturing of trunk and extremities of nonepileptic origin, and other types of myoclonic activity.
Infants with benign myoclonic epilepsy in infancy (BMEI) may have repetitive jerks, but the seizures are much briefer than spasms, and the EEG during the seizures reveals 3 Hz spike-and-wave or polyspike-and-wave activity. The background EEG activity is usually normal. These myoclonic seizures are treated with standard anticonvulsants, and the long-term prognosis in these patients is favorable. Another type of myoclonic movement that has been reported to be confused with infantile spasms is the so-called benign myoclonus of early infancy (131). Infants with this disorder reportedly have tonic and myoclonic movements involving either the axial or limb musculature, which, like infantile spasms, may occur in clusters. The age at onset of this disorder (3 to 8.5 months) coincides with the age at onset of infantile spasms. In none of the reported cases did these movements persist beyond the age of 2 years. Unlike patients with infantile spasm, infants with benign myoclonus of early infancy have normal development, normal neurologic examinations, and normal EEGs. The motor movements are not accompanied by EEG changes, thus suggesting a nonepileptiform basis for the events. Patients with severe myoclonic epilepsy in infancy (SMEI), a disorder that may be confused with infantile spasms, usually have a family history of seizures and the disorder often begins following a prolonged febrile seizure. Unilateral clonic seizures, generalized tonic–clonic seizures, and myoclonic seizures, but not epileptic spasms, typically occur in these patients. The EEG reveals generalized spike-and-wave or polyspike-and-wave activity (132–134). The seizures are typically refractory to anticonvulsants and mental retardation and neurological deficits are common. Epilepsy with myoclonic–astatic seizures (EMAS) may also be confused with infantile spasms. However, these patients experience brief myoclonic seizures, not epileptic spasms. The EEG typically shows spike-and-wave or polyspike-and-wave activity (135). Most patients are developmentally normal before onset of this disorder, which tends to be at a later age (7 months to 10 years) than infantile spasms.
Related Syndromes
Three different epilepsy syndromes—early myoclonic encephalopathy, early infantile myoclonic epilepsy, and Lennox–Gastaut syndrome—may be difficult to differentiate from infantile spasms. They may share a common pathophysiologic basis, with each disorder being expressed at a different age. A comparison of the important ictal and interictal features of these disorders, as well as the other myoclonic epilepsies described previously, is provided in Table 30.1.
Ohtahara (136) proposed that the syndrome of infantile spasms, suppression-burst activity in the EEG, and developmental retardation, when seen in the first few months of life, represents a disorder different from that seen in older infants, and he termed this disorder early infantile epileptic encephalopathy (EIEE). This disorder, which has been reported by many authors (134,137–144), has a high mortality rate. The major reported difference between EIEE and infantile spasms is that the suppression-burst pattern seen with EIEE is continuous during wakefulness and sleep, whereas infantile spasms are associated with hypsarhythmia. However, as discussed previously, a suppression-burst variant of hypsarhythmia may be seen in patients with infantile spasms. Without knowing the age and clinical history of the patient, it is not possible to differentiate between these two suppression-burst patterns. Another reported difference between EIEE and infantile spasms is that in infantile spasms the spasms occur almost entirely while the patient is awake, whereas the spasms associated with EIEE reportedly occur both during wakefulness and sleep (139,143).
A similar syndrome, early myoclonic encephalopathy (EME), has an onset within the first few weeks of life (133,134,145). This syndrome differs from EIEE and infantile spasms chiefly by the main type of clinical seizure observed. EME patients reportedly have fragmentary myoclonus, whereas patients with infantile spasms and EIEE have epileptic spasms. However, EME patients reportedly begin to experience epileptic spasms as they grow older. Also, the EEG in EME shows a suppression-burst pattern, but it is reportedly less persistent than the suppression-burst pattern seen in EIEE. The various etiologies associated with these three disorders overlap, although EME has been reported to be associated primarily with metabolic disorders, whereas EIEE is more likely to be associated with structural brain abnormalities (134,138,143,145–147).
It is usually not difficult to differentiate Lennox–Gastaut syndrome from infantile spasms in the infant younger than 1 year of age. However, in older patients, the myoclonic, brief tonic, and atonic seizures seen in the patient with Lennox–Gastaut syndrome may be confused with infantile spasms, particularly on the basis of clinical description alone.
The fact that these syndromes transition from one to the other also complicates the issue. For example, an average of 71% of patients with EIEE transition to infantile spasms. Some of these patients then transition to Lennox–Gastaut syndrome. Some cases of EME reportedly transition to EIEE, and some cases of EIEE may evolve directly to Lennox–Gastaut (14,137,139,148). In addition, an average of 17% of patients with infantile spasms will evolve to Lennox–Gastaut syndrome. If these disorders do share a common pathophysiologic mechanism, with the stage of brain maturation being the only factor affecting the appearance of each disorder, it is difficult to explain why the following evolution is not seen in all or most patients: EME —> EIEE —> infantile spasms —> Lennox–Gastaut syndrome. From this brief discussion, it is clear that much additional work is needed to clarify the relationship of these disorders.
TABLE 30.1
Differentiation of infantile spasms from nonepileptic events, other types of myoclonic activity, and the three syndromes just described frequently requires that video-EEG monitoring studies be performed to capture the questionable episodes and thus provide a definitive diagnosis.
TREATMENT
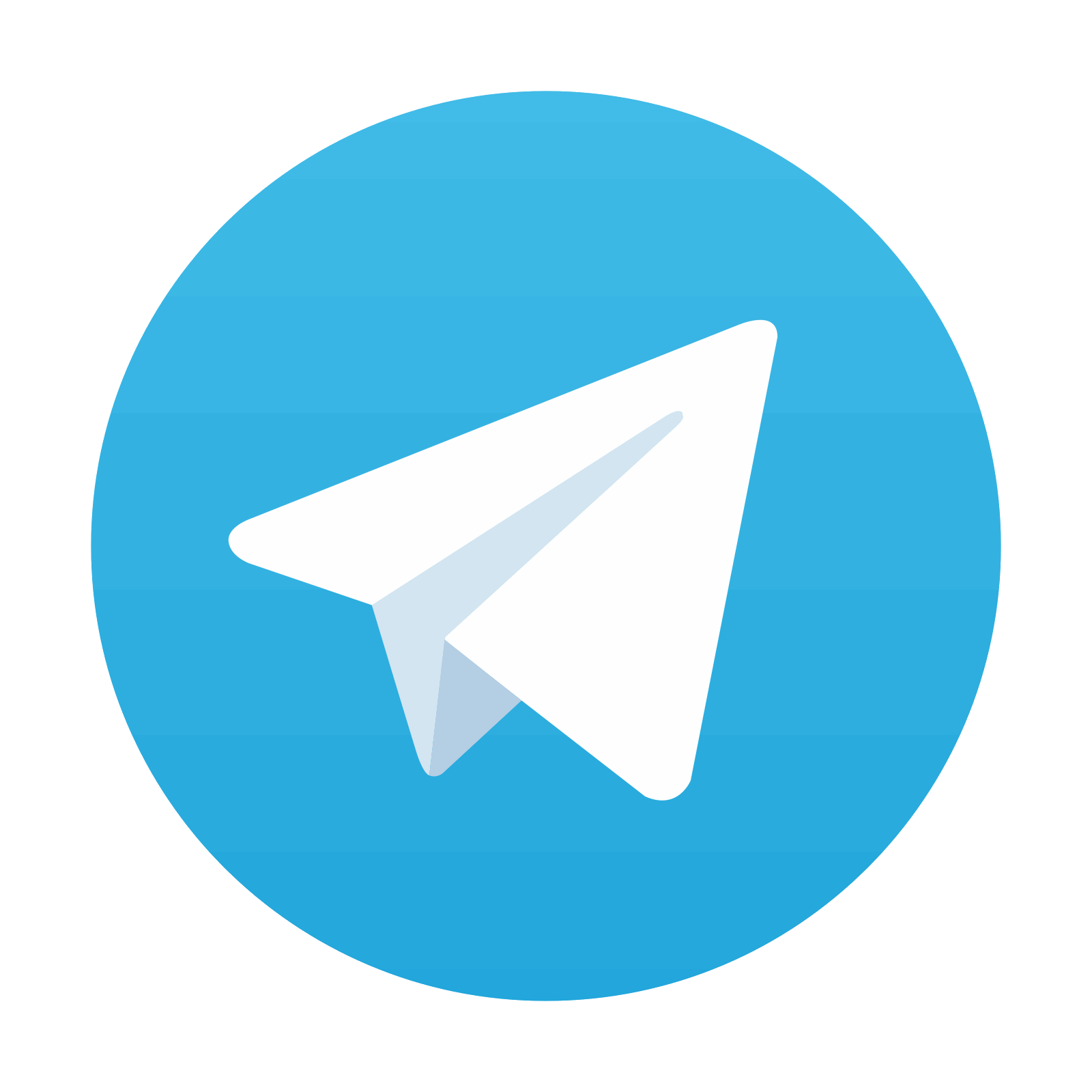
Stay updated, free articles. Join our Telegram channel

Full access? Get Clinical Tree
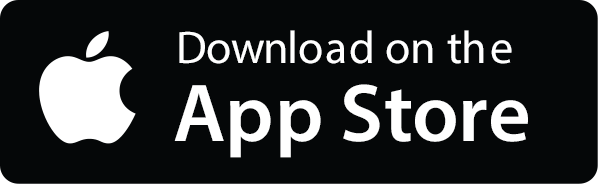
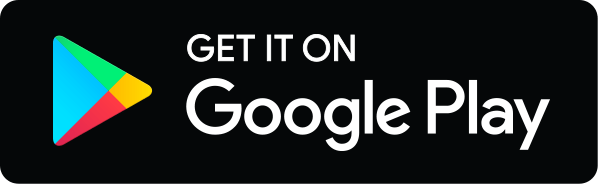