Findings on physical examination often suggest the origin and likely causative organisms in patients with infections involving the skin and subcutaneous tissues.
When planning operative intervention for lymphadenitis, it is important to preoperatively plan either incision and drainage for abscess formation or lymphadenectomy for suspected mycobacterial infection.
A high index of suspicion of necrotizing fasciitis and early surgical intervention are keys to optimizing patient outcomes.
Laboratory and imaging studies are important adjuncts for the diagnosis of body cavity infections.
We live in a hostile environment. Since the beginning of life on earth, all living organisms have been under attack by would-be invaders seeking to use the nutrients within living tissues for their own survival, often to the detriment of the host. To combat the constant threat of invasion, all living organisms have developed measures to resist microbial invasion. In higher organisms, the mechanisms to resist microbial invasion have become quite complex and are usually extremely effective. However, occasionally resistance to invasion is overcome, due either to a break in host defenses or to a microbial adaptation that creates a more effective invader. When host invasion occurs, the resulting infection will frequently require medical treatment. In some cases, effective treatment also includes surgical intervention.
Unfortunately, many of the disease conditions that require surgical treatment, as well as the nature of the surgical intervention itself, alter host defense mechanisms and thus create further opportunities for microbial invasion. It is not surprising therefore that postoperative infection is the most common complication associated with many surgical procedures. Thus, instituting measures to decrease the risk of postoperative wound infections, in addition to early recognition and expeditious treatment of surgical site infections (SSIs) when they do occur, are essential aspects of optimal surgical care.
In higher organisms, all exposed body surfaces (external and internal) are covered by a layer of epithelial cells that serve as a mechanical barrier against microbial invasion, while invasion between cells is inhibited by close apposition of the cellular membranes of adjacent epithelial cells. Besides creating a mechanical impediment to microbial invasion, most epithelial surfaces also have specialized adaptations that limit bacterial attachment and invasion. For example, the epidermis of the skin is composed of multiple layers of cells, the outer layers of which are inanimate, keratinized cells creating a dry, acidic inhospitable environment. Continuous cell shedding facilitates removal of attached organisms, while glandular secretions contain free fatty acids, lysozymes, and β-defensins that inhibit microbial growth. In the respiratory tract, a continuous layer of mucous covers the epithelial surface, trapping inhaled pathogens, which are then swept toward the pharynx by the continuous movement of cilia on the luminal surface of the epithelial cells. In addition, glandular secretions contain defensins and immunoglobulins that inhibit bacterial growth and attachment to epithelial surfaces. The urinary tract maintains sterility via a continuous flow of urine which has bacteriostatic properties due to its low pH, high ammonia and urea content, and high osmolality. In the gastrointestinal system, the epithelial defense mechanisms vary at different levels of the digestive tract. The saliva is rich in antibacterial agents including lysozyme and phospholipase A2. In the stomach, low pH and proteases kill most ingested microorganisms. Beyond the pylorus, a continuous mucous layer covers the epithelial surface, trapping organisms and inhibiting contact with the epithelial cell surface. In addition, secreted immunoglobulins coat microbial surfaces thereby inhibiting attachment, while continuous peristalsis propels unattached microorganisms distally for elimination.
Destruction of microorganisms that breach epithelial barriers and enter the body is dependent on the immune system. The human immune system is often considered as 2 separate components, the innate immune response and the adaptive (acquired) immune response. However, there are multiple interactions and effector mechanisms that are shared between these 2 arms. The immune response in humans is very complex, and therefore a complete discussion is beyond the scope of this chapter.
Innate immunity is the more ancient of the immune responses and is present in all multicellular organisms. The components of the innate immune response are preformed and functional, allowing immediate recognition and destruction of invading microorganisms. Components of innate immunity include several of the epithelial adaptations noted previously (ie, defensins, lysozyme), plus cellular effectors (macrophages, dendritic cells, neutrophils, and natural killer [NK] cells) and humoral components (complement, interferons). Innate immune responses rely on detection of carbohydrates, lipids, or other cellular components (eg, double-stranded RNA) that are unique to microorganisms (ie, pathogen-associated molecular patterns or PAMPs) by pattern-recognition receptors (PRRs). Examples of PRRs include: the Toll-like receptors (TLRs), a family of intracellular and cell surface receptors that activate downstream adapter proteins resulting in induction or suppression of genes that help to orchestrate the immune response; mannan-binding lectins (MBLs), a serum protein that binds to a unique polysaccharide on the surface of many bacteria and yeasts (mannan) thereby facilitating phagocytosis and activation of the complement system; and nucleotide-binding oligomerization domain (NOD)-like receptors (a.k.a., nucleotide-binding domain, leucine-rich repeat containing proteins or nucleotide leucine rich receptors [NLR]), a family of cytoplasmic receptors that recognize microbial and stress-induced molecules causing activation of caspases that cleave and activate inflammatory cytokines, and activate NF-κB, a transcription factor for genes involved in the immune response.
The innate immune response has several important roles, one of which is to activate the adaptive immune response as discussed in the section that follows. Other critical functions include the elaboration of signals that trigger inflammation and the killing of invading microorganisms. Both cellular and humoral components of the innate immune system contribute to microbial destruction. The cellular mediators include phagocytic cells (eg, neutrophils, macrophages, and dendritic cells) that are capable of recognizing and ingesting foreign material. Once ingested, the resulting phagosome containing the microbe fuses with a lysosome that contains acid hydrolases and enzymes (eg, nitric oxide synthase, NAPH oxidase, superoxide dismutase, and myeloperoxidase) capable of producing reactive oxygen intermediates. These enzymatic processes cause microbial destruction, and in macrophages and dendritic cells allow partial digestion of microbial proteins for presentation on the surface of the phagocyte. Another cellular mediator of microbial killing is the NK cell. NK cells are lymphocytes that possess some, but not all T-cell surface markers. Unlike other cellular killers, NK cells are not phagocytic. Instead, NK cells kill by releasing perforins (pore forming proteins) and proteases (granzymes) in close proximity to target cells resulting in cell death by apoptosis or osmotic cell lysis. NK cells are activated by interferons and macrophage-derived cytokines but are inhibited by surface receptors that recognize major histocompatibility complex (MHC) class I alleles (thus preventing killing of host cells). MHC class 1 alleles frequently are downregulated on the surface of virus infected and tumor cells, which facilitates attack by NK cells.
Humoral mediators of microbial killing include defensins, lysozyme, LPS-binding protein, pentraxins (eg, C-reactive protein, serum amyloid protein), collectins (eg, MBL, surfactant proteins A and D), and the complement system. Several of these humoral factors directly attack invading microorganisms (eg, lysozyme hydrolyzes peptoglycans in the cell wall of bacteria, defensins form pore-like membrane defects), while others contribute to phagocytosis by opsonization or activation of the complement system. The complement system consists of approximately 30 known serum and membrane-bound proteins and protein regulators that are synthesized mainly in the liver. Once activated, the complement system has 3 main roles: lysis of cells, generation or inflammatory and chemotactic mediators, and facilitation of phagocytosis by opsonization through attachment to foreign material. There are 3 known pathways for activation of the complement system, all of which ultimately lead to activation of C3 (Fig. 13-1). Once activated, C3 binds and activates additional proteins leading to cell lysis and generation of inflammatory signals and opsonins. The “classical pathway” is activated when IgG or IgM antibodies bind an antigen allowing activation of C1q by the antibody’s Fc fragment. Activated C1q autoactivates 2 associated proteins (C1s, C1r) leading to recruitment and activation of additional proteins (C4, C2) generating C3 convertase. The “alternative pathway” is activated when cell surface molecules (eg, lipopolysaccharides) directly bind C3 causing a conformational change leading to hydrolysis and activation of C3 and binding of factor B. Once bound, factor B is cleaved by a serine protease and the resultant complex (hydrated C3 + Bb) functions as the C3 convertase of the alternative pathway. Finally, the “lectin pathway” is activated when MBL binds mannan on the surface of a microbe. MBL is structurally similar to C1q and is associated with mannose-binding lectin-associated serine proteases (MASPs). Binding of MBL to mannan causes activation of MASP, which like C1r and C1s bind and activate C4 and C2 resulting in generation of C3 convertase. C3 convertases cleave C3 into a and b fragments. The C3b binds with the C3 convertase creating C5 convertase, which in turn cleaves C5 into a and b fragments. The complement cascade continues as C5b complexes with C6 and C7, which in turn interact with C8 and C9 to create a pore-forming complex that penetrates the cell membrane causing loss of osmotic equilibrium and cell lysis. Besides cell lysis, some small fragments generated during the complement cascade also serve other important functions including chemotaxis and anaphylaxis (C3a, C4a, and C5a) as well as opsonization (C3b).
The adaptive immune system is evolutionarily a relative newcomer being present only in vertebrates. Like innate immunity, the adaptive immune system has both cellular and humoral components. However, unlike the innate immune system, most of the response elements for the adaptive immune response are not preformed, but rather are generated and/or amplified following exposure to a pathogen. Thus there is a lag time between exposure to a pathogen and maximal activation of the adaptive immune response. Also, unlike the innate immune system which responds to common PAMPs, the individual components of the adaptive immune system recognize and respond to very specific antigens. Finally, unlike innate immune responses, prior exposure to a pathogen results in “immunologic memory.” Thus, subsequent invasion of the same or a similar pathogen are met by a more rapid and robust immune response.
Cellular elements of the adaptive immune system include the T (a.k.a., thymus-derived) and B (a.k.a., bursa of Fabricius or bone marrow-derived) lymphocytes. Both begin as lymphoid progenitors in the bone marrow or fetal liver and must undergo a series of maturation steps to become fully functional. One step, common to both T and B lymphocytes, is a series of genomic rearrangements that culminate in the generation of a unique surface protein receptor (T-cell receptor [TCR] in T-cells, immunoglobulins in B-cells) that accounts for the lymphocyte’s antigen binding specificity. During maturation, T and B cells also acquire additional surface receptors that dictate function and are necessary for interaction with other immunologically active cells or pathogens. In addition, lymphocytes that express receptors that react with “self” proteins or fail to recognize self MHC proteins are identified and destroyed by apoptosis.
Activation of the adaptive immune response to most pathogens begins with the innate immune system. Initially invading microorganisms are ingested by antigen presenting cells (APCs) (dendritic cells, macrophages, B-cells, and basophils) and are partially digested. Inside the APC, microbial antigens are reprocessed and presented on the surface of the APC in conjunction with “self” MHC proteins. APCs are present in large numbers in the skin and mucosa. Engulfment of foreign material induces migration of the APC to regional lymph nodes where interaction with T cells can occur. T-cell activation is initiated when the TCR recognizes a peptide/MHC complex on the surface of an APC, which results in clustering of other membrane-associated molecules (CD3 and CD4 or CD8) at the interface between the T cell and the APC. This APC–T cell interface is further stabilized by the interaction of adhesion molecules (LFA-1 on T-cells to ICAM-1 on APCs). T-cell activation also requires costimulatory signals (eg, B7 protein on the APC must interact with CD28 on the helper T cell). Failure of this secondary signaling results in a state of anergy specific to that antigen. Activated T cells produce cytokines that induce activation of downstream effectors. One of these cytokines, interleukin-2 (IL-2) produces a positive-feedback effect by inducing T-cell activation and clonal expansion of antigen-specific helper T cells. This positive feedback effect is inhibited by a different protein called cytotoxic T lymphocyte antigen-4 (CTLA-4), which also binds B7 and blocks IL-2 synthesis. CTLA-4 synthesis occurs in activated T cells and is important for inducing quiescence and generating memory cells.
T cells perform important functions which are determined by the expression of surface proteins (CD4 and CD8). Most CD4+ lymphocytes perform “helper” functions, of which there are 2 subpopulations, Th-1 cells and Th-2 cells. CD4+ cells are activated when TCRs interact with an antigen–MHC class II complex. Activated CD4+ helper cells produce cytokines that stimulate specific effector responses. Th-1 helper cells produce IL-2 and gamma interferon that activate cytotoxic T-cells and macrophages (cell-mediated responses); while Th-2 helper cells produce IL-4, IL-5, IL-6, and IL-10 which stimulate B cells (antibody-mediated response). The balance of Th-1 to Th-2 cells is regulated by IL-12, which increases the number of Th-1 cells, and gamma interferon, which inhibits the proliferation of Th-2 cells. Cytotoxic T-lymphocytes (CTLs) are CD8+ lymphocytes and are primarily involved in killing virus-infected and tumor cells. CD8+ cells recognize antigens associated with MHC class I molecules on the surface of infected cells. CTLs kill by inserting perforins and releasing degradative enzymes called granzymes into infected cells. CTLs also induce apoptosis by surface expression of Fas ligand which engages the Fas receptor (CD95) on the surface of the target cell.
Another class of T lymphocytes is the regulatory T cell. Regulatory T cells account for only 5% to 10% of CD4+ lymphocytes. They express CD4, CD25 (IL-2 receptor α chain), and CTLA-4 on their surface and produce the immunosuppressive cytokines (TGFβ and IL-10), but not IL-2. Activated regulatory T cells suppress proliferation and cytokine production of CD4+ and CD8+ T cells and thus downregulate the immune responses to foreign antigens. Regulatory T cells induce tolerance and are important for preventing autoimmune responses.
The humoral arm of the adaptive immune response requires activation of B lymphocytes which results in the production of antibodies (immunoglobulins) by plasma cells. Immunoglobulins serve a number of important functions including neutralization of toxins and viruses, opsonization of microbes to facilitate phagocytosis, complement activation, and blocking attachment of microbes to mucosal surfaces. B cells recirculate through secondary lymphoid organs (lymph nodes, spleen, and mucosal-associated lymphoid organs) where contact with foreign antigens is most likely to occur. In lymph nodes, B cells are concentrated in the cortex in contact with follicular dendritic cells. Foreign antigens may enter B-cell rich regions of the lymph node by diffusion or by being carried on the surface of macrophages, follicular dendritic cells, or other B cells. In addition, B-cell activation can occur via T-cell dependent or independent pathways, although most antibody responses require the participation of T cells. T-independent antigens include protein or polysaccharide molecules with repeating molecular patterns that are capable of cross-linking multiple immunoglobulin receptors on the surface of the B lymphocyte. T-cell dependent B-cell activation begins with binding of antigen by the immunoglobulin (IgM or IgD) component of the B-cell receptor (BCR). B-cell activation requires cross-linking of BCRs, phosphorylation of BCR-associated proteins, and the assembly of an intracellular signaling complex (signalosome). The signalosome leads to internalization of the antigen, which is then processed, loaded onto MHC proteins, and presented on the surface of the B cell for recruitment and activation of CD4+ Th-2 helper cells. Once activated, B cells may become short-lived plasma cells that release large amounts of IgM, or they may undergo further maturation. B-cell maturation includes class-switching (ie, change in antibody production from IgM or IgD to IgG, IgA, or IgE), and affinity maturation (a.k.a., somatic hypermutation) via a series of nucleotide substitutions in the heavy and light chain variable regions resulting in the generation of antibodies with higher affinity. B-cell maturation processes occur in response to cytokine signals. Ultimately, some activated lymphocytes become quiescent long-lived memory T and B cells, which are capable of being rapidly reactivated during subsequent exposures to an antigen. Thus, subsequent exposures to a pathogen are met by the more rapid appearance of high affinity IgG, IgA, or IgE immunoglobulins (secondary response), while first encounters with a pathogen (primary response) initially generate lower affinity IgM antibodies and require a lag time of 2 weeks or more to generate high affinity IgG, IgA, or IgE antibodies.
Globally, more than 2 million children die annually due to infectious diseases. Multiple developmental deficiencies in the immune system contribute to the increased risk of death due to infection in infants and children. In the neonate, the skin is fragile at birth and more susceptible to trauma. Tracheal secretions at birth are devoid of IgA and antimicrobial peptides including β-defensins increase with gestational age. In the gastrointestinal tract, gastric pH of the neonate is higher and intestinal peristalsis is less efficient. The neonate, however, does have some unique epithelial defense adaptations. These include the vernix caseosa, which limits desiccation of the skin, lowers surface pH, and contains a number of antimicrobial substances (lysozyme, α-defensins, ubiquitin, psoriasin, and free fatty acids). In addition, human breast milk contains antimicrobial peptides including lactoferrin and lysozyme. Human breast milk also contains factors that modulate TLR signaling, which are thought to support the establishment of beneficial bacteria as part of the intestinal microflora.
At birth many components of the innate immune system are deficient. Serum levels of most of the complement system proteins (C1q, C2, C3, C4, C5, factor B, and properdin) are significantly lower in neonates than in adults. C3 levels decrease initially after birth, but recover by 3 weeks of age and reach adult levels by about 6 months of age. Levels of several of the acute phase proteins (MBL, C-reactive protein [CRP], and lipopolysaccharide binding protein [LBP]) are also low at birth, but rise during the first weeks of life. Circulating neutrophil counts are higher in newborns than in adults, but neonates have decreased numbers of granulocyte and monocyte precursors. As a result, neonates frequently have an inadequate pool of neutrophils to respond to infection. Furthermore, the neutrophils in neonates exhibit functional impairments in chemotaxis, rolling adhesion, transmigration and lamellipodia formation (due to decreased expression of complement receptor 3 and L-selectin), and microbial killing (due to a decrease in granule content of antimicrobial peptides and impaired oxidase activity). APC function in the neonate is also impaired due to decreased surface expression of MHC class II molecules and decreased numbers of resident dendritic cells in secondary lymphoid organs. The latter alteration contributes to inefficient antigen presentation. Finally, the production of some cytokines by APCs in the neonate is decreased (eg, IFNγ, TNF, and IL-12p70), while the production of other cytokines (eg, IL-6, IL-10, and IL-23) exceeds that of adults.
The adaptive immune system of the neonate is naïve. Thus, responses to foreign antigens have the characteristics of a “primary responses.” In addition, T-helper cell function in the neonate is generally depressed and altered production of cytokines by neonatal APCs results in a bias toward Th-2 responses and against Th-1 responses. Diminished Th-1 responsiveness contributes to greater vulnerability to certain microbial infections, while increased Th-2 responsiveness is believed to increase susceptibility to allergic reactions. Th-1 mediated responses are also impaired by regulatory T-cells, which are relatively more abundant and more potent in the fetus and in the newborn.
Antibody responses to both thymus-dependent and thymus-independent antigens are also impaired in neonates and infants. In part this is due to the architectural immaturity of the secondary lymphoid structures where foreign antigens are processed. Specifically, lymph node germinal centers do not appear until around 4 months after birth, and marginal zone B-cell numbers in the spleen do not reach adult levels until around 2 years of age. Absence of lymphoid germinal centers, alterations in the function of APCs, and decreased expression of the costimulatory molecules by neonatal B-cells all contribute to decreased responses to thymus-dependent antigens. Decreased numbers of marginal-zone B cells in the spleen and low serum levels of the complement protein C3, coupled with decreased expression of the corresponding receptor (CD21) on the surface of neonatal B cells, contribute to impaired responses to T-cell independent antigens. Even after activation, B cells in neonates and infants generate fewer antibody secreting plasma cells, which accounts for decreased antibody titers of shorter duration. In addition, impaired antibody maturation results in the production of lower affinity antibodies and limited expression of certain classes of immunoglobulins. Thus, serum levels of IgM reach adult levels at approximately 1 year of age, while adult levels of IgG are not attained until approximately 5 to 7 years of age and serum IgA levels do not reach adult levels until 10 to 14 years of age (although salivary levels of IgA reach adult levels by around 2 months of age). The IgG2 subclass of immunoglobulin is known to be particularly effective at recognizing polysaccharide antigens. Low levels of IgG2 combined with impaired responses to T-cell independent antigens are believed to contribute to the increased susceptibility of infants and neonates to infections caused by encapsulated bacteria.
The virulence of a microbial pathogen is generally defined as the ability of the organism to cause disease. The virulence of a given microbial pathogen is reliant in part on the relative susceptibility of the host. Virulence can be thought of as the gene products of an organism that facilitate colonization, growth, and survival within the host organism and allow spread to a new host. The key steps are initial colonization and attachment, multiplication and nutrition, evasion of host defenses (in some cases this also includes invasion of cells involved in the immune response), and lastly exit to spread to a new host. Microbial examples include the PapG P-pili of uropathogenic Escherichia coli, which allows attachment and colonization of the kidney and subsequent pyelonephritis. Another example is the Type III secretion system (TTSS) of Pseudomonas aeruginosa, which mediates the delivery of toxins directly into host eukaryotic cells and allows invasion and subsequent serious infections such as pneumonia. Bacterial capsules have long been recognized as important virulence factors. For example, E. coli that express the K5 capsule are not associated with significant infections in neonates while K1 capsule E. coli are associated with meningitis and disseminated sepsis. The K1 capsule camouflages the bacteria because of similarity with host tissues. Group B streptococcus (GBS) capsule is the most well-defined virulence factor of GBS. The capsule protects GBS from opsonization by C3 through inhibition of the alternative complement pathway in the absence of type-specific antibodies to capsular antigens. However, GBS may also express other factors that allow it to resist opsonophagocytosis and cause life-threatening neonatal bacterial infection.
Defects in immune function can be classified as primary immunodeficiencies (ie, a defect in immune function not attributable to another illness) and secondary immunodeficiencies (ie, immunodeficiency arising in a previously normal individual attributable to an associated condition). Secondary immunodeficiencies are much more common than primary immunodeficiencies. In fact most serious or prolonged illnesses have a suppressive effect on immune function.
The primary immunodeficiencies comprise more than 130 different disorders. Most are rare with prevalence rates of approximately 1:10,000 births. Many primary immunodeficiencies are due to a defect in a single gene; however, variations in penetrance and interactions between genetic and environmental factors can result in wide phenotypic variability. Commonly applied classification systems divide these conditions into: combined T- and B-cell deficiencies; predominantly antibody deficiencies; immune dysregulation; defects of phagocyte number, function, or both; defects in innate immunity; autoinflammatory disorders; and complement deficiencies. All primary immunodeficiencies are characterized by increased susceptibility to recurrent or severe infections caused by specific pathogens. Responsible pathogens and types of infectious complications will depend on the nature of the immune defect.
Secondary deficiencies in immune function can result from a multitude of preexisting conditions. Common etiologies include severe burns and trauma, severe malnutrition, specific viral infections (eg, cytomegalovirus [CMV], Epstein-Barr virus [EBV], and human immunodeficiency virus [HIV]), neoplasias (eg, leukemias, lymphomas), medical therapies (eg, immunosuppressive or chemotherapeutic drugs, irradiation), and chronic disease states (eg, renal failure, diabetes mellitus). Extremes of age, including newborn and premature infants, are also associated with alterations in immune function as described previously. While some of these etiologic agents are known to affect the function of specific cellular components of the immune system (eg, HIV infects CD4+ T helper cells, EBV encodes an IL-10 like protein [BRCF-1] that inhibits Th1 responses), most cause global deficiencies due to impairment of cellular division and/or maturation of 1 or more elements of the immune response. Affected patients are at increased risk of developing infections due to opportunistic organisms, or may experience increased frequency or severe complications associated with infections caused by common pathogens.
An underlying immune deficiency should be considered in any patient with a history of chronic or recurrent infections or a history of infections due to unusual pathogens. A record of the types, locations and frequency of infections, as well as the causative organisms should be sought. Recurrent gastrointestinal or respiratory symptoms or a history of growth failure or chronic weight loss may suggest a primary etiology or may be attributable to recurrent infections. A history of chronic medical illnesses as well as any history of recent or current drug exposures or medical treatments may suggest a possible etiology. A family history of frequent or recurrent infectious complications in an ancestor might suggest a primary immunodeficiency; however, it is important to remember that most patients have no family history since many of these conditions arise from de novo mutations or they may be the first occurrence of an autosomal recessive disease. Finally, findings on physical examination (eg, absent tonsils, partial albinism, telangiectasias, petechiae, and microcephaly) may also suggest a primary immunodeficiency.
While the laboratory evaluation of a patient with a suspected immunodeficiency can become quite complex, the initial studies should include at least a complete blood count (CBC), with differential, skin tests to check for cellular immune responses to common antigens and viral serologies (ie, HIV). Additional studies including measurements of total and specific antibody or complement component titers and tests of lymphocyte and granulocyte function may be indicated depending on the patient’s history and results of preliminary laboratory studies.
Treatment for patients with primary immunodeficiencies includes antibiotic prophylaxis to decrease the risk of infections caused by common and opportunistic organisms, replacement of specific deficiencies (ie, immunoglobulins) where available, and possible gene therapy or bone marrow transplantation depending on the diagnosis. The treatment for secondary immunodeficiencies is primarily directed at identifying and correcting the underlying etiology. However, patients often receive antibiotic prophylaxis and may benefit from specific replacement therapy (eg, intravenous immunoglobulin infusions) or cytokine treatment (eg, granulocyte-macrophage colony stimulating factor) to stimulate production of immune cells until normal immune function is restored.
The human body comprises 10 times more microbial cells than human cells due to the large number of microbes that inhabit the gastrointestinal tract. In the first year of life, the infant intestinal tract progresses from sterility to an adult-like colonization of 1012 microbes per milliliter in the colon. The gastrointestinal microflora of an infant is initially composed of organisms from environmental exposures such as maternal vaginal, fecal, or skin microbiota. The rate at which the gastrointestinal tract of the newborn infant is colonized and the types of organisms in the newborn infant’s intestinal microflora are influenced by the route of delivery, diet, maternal flora, and the neonatal environment.
Newborns delivered vaginally are colonized by anaerobic bacteria, predominantly Bacteroides, within 1 week of age. In infants delivered by C-section, anaerobic colonization is delayed, and the infant’s microflora is initially more reflective of the hospital’s flora than that of their mother. Beginning approximately 1 week postnatally, mode of feeding becomes the most important determinant of gastrointestinal flora in otherwise healthy neonates. In breast-fed infants lactobacilli and bifidobacteria outnumber Enterobacteriaceae in the gastrointestinal tract by nearly 1000-fold. In contrast, Enterobacteriaceae predominate in formula-fed infants. However, a complex bacterial microflora is established more rapidly in formula-fed infants than in breast-fed infants, possibly due to the iron content of infant formulas which fosters bacterial growth. The introduction of formula feedings in breast-fed infants causes a change in the bacterial profile to one that is more typical of formula-fed infants. Ultimately, the transformation of the intestinal microbial flora to a pattern that is adult-like is associated with the transition from a milk-based diet to the typical toddler diet. This transition is marked by a decrease in the relative numbers of E. coli and Clostridium spp., and an increase in Bacteroides and gram-positive anaerobic cocci.
Normal commensal bacteria have a number of positive effects on gastrointestinal physiology and immune function. The presence of bacteria in the gastrointestinal tract improves mucosal barrier function by activating genes responsible for epithelial cell turnover and mucus secretion resulting in the growth of intestinal villi and crypts and an overall increase in gut mass. Some intestinal microbes also synthesize essential vitamins and generate short-chain fatty acids that are absorbed and metabolized by the colonic epithelium. Commensal organisms also contribute to development of the neonatal immune system by releasing antigens that induce a low-grade inflammatory response that stimulates the growth and maturation of mucosa associated lymphoid tissues (Peyer patches and lymphoid follicles) in the neonatal intestine. The presence of normal microbial flora in the gut also inhibits the growth of enteric pathogens by competing for nutrients, secreting antibacterial substances, and interfering with adherence to mucosal surfaces. Finally, the resident microflora may also provide a protective effect by degrading bacterial toxins. Paucity of immune stimulation in infancy associated with alterations in the normal neonatal intestinal microbial flora has proposed as an etiology for the increasing prevalence of allergies and autoimmune disorders in many western countries. However, this association has not been proven.
The development of gastrointestinal microflora in preterm neonates is frequently disrupted by multiple environmental influences in the neonatal intensive care unit (ICU). Some of these factors include frequent exposure to broad-spectrum antibiotics, gavage feeding, formula feeding, and alterations in intestinal motility. The hostile environment of the neonatal ICU increases the likelihood that the intestinal tract will become colonized with: (1) gram-negative enteric pathogens such as E. coli, Enterobacter, and Klebsiella, (2) coagulase-negative Staphylococcus, and (3) Candida species. Recently there has been an increasing interest in the use of nonpathogenic commensal bacteria (probiotics) to promote colonization of the gut with beneficial organisms in an attempt to improve the maturity and function of the gut mucosal barrier. A recent meta-analysis of 11 previous studies that used probiotics for prevention of necrotizing enterocolitis (NEC) in preterm neonates reported significant decreases in death and the development of NEC, with a 30% reduction in NEC overall. Multiple strains of probiotics have been studied including: Bifidobacterium breve, Lactobacillus GG, Saccharomyces boulardii, Bifidobacterium bifidus, Lactobacillus BB-L, Streptococcus thermophilus, Bifidobacterium infantis, Lactococcus acidophilus, Bifidobacterium lactis, and Bifidobacterium longum. Additional randomized studies are required to determine the most efficacious bacterial agent(s), as well as optimal timing, dosage, and contraindications. Identifying the environmental and genetic factors that determine the characteristics of our gut microflora and how this microflora influences our physiology and health are promising areas for future research.
Mediators produced as part of the immune response, as well as toxins released by bacteria result in a constellation of symptoms that clinicians recognize as an inflammation. Inflammatory responses reflect the body’s response to injury and are necessary for controlling the causative agent and for initiating wound repair. However, inflammation is not necessarily synonymous with infection, since inflammatory responses can be induced by injurious agents other than invading microbial pathogens.
Inflammatory responses may be acute or chronic, local, regional, or systemic. Inflammation progresses from local to systemic via a stepwise progression depending on the magnitude and persistence of the inciting event. Stage 1 or local inflammatory responses result when chemical mediators including histamine, prostaglandins, leukotrienes, and bradykinin are released by resident tissue cells (histiocytes, macrophages, dendritic cells, and mast cells). These mediators result in the 4 classical signs of inflammation: rubor (redness due to vasodilation), calor (warmth due to vasodilation), dolor (pain due to nerve stimulation), and tumor (swelling due to local tissue edema). These initial mediators also induce an influx of humoral and cellular response elements. Subsequently, a regional response (stage II) develops when cytokines and products of complement system activation (C3a and C5a) are released into the local circulation and recruit cellular elements of the immune system to the site of injury. Early responders include macrophages and neutrophils which kill invading microorganisms and eliminate injured tissue. Early responders also release cytokines, enzymes, and nitric oxide that further contribute to local signs of inflammation. If the initial response is able to contain and eradicate the stimulus, the local production of proinflammatory stimuli decreases and endogenous antagonists are released causing a downregulation of the inflammatory response. However, if the injurious agent exceeds local control, inflammatory cytokines (eg, IL-1, IL-6, IL-8, interferon-γ, and TNF-α) and bacterial toxins enter the central circulation resulting in systemic signs of inflammation (stage III). Unfortunately, if systemic inflammation persists serious negative consequences will result leading due end-organ dysfunction as a result of effects on metabolism, circulatory integrity, and hemostasis.
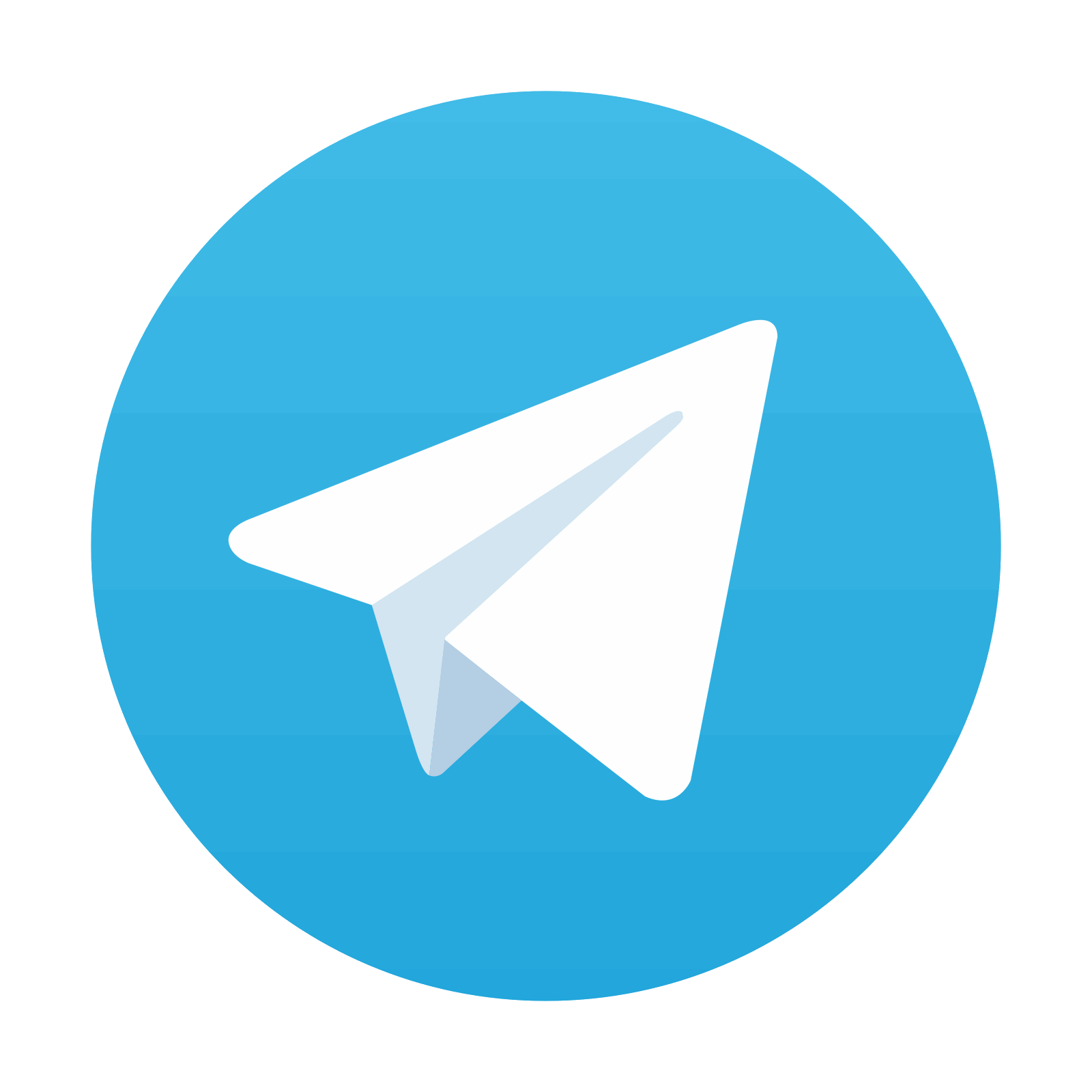
Stay updated, free articles. Join our Telegram channel

Full access? Get Clinical Tree
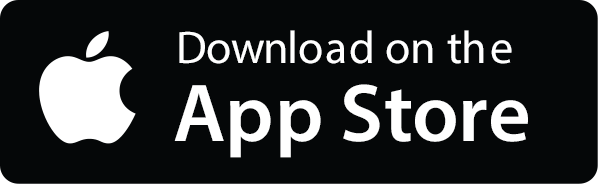
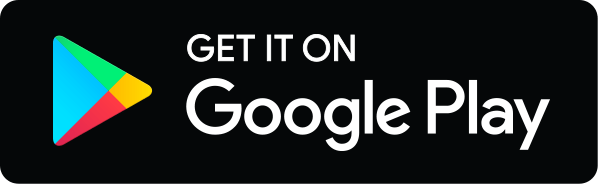