Sulfonamides
Chokechai Rongkavilit
Nahed Abdel-Haq
Jocelyn Y. Ang
Basim I. Asmar
Introduction
Sulfonamides are some of the most common and the oldest antimicrobial agents used in children. Their mode of action, efficacy, safety, and pharmacologic properties are well known and these drugs remain to be a substantial component of antimicrobial therapies in infants beyond the immediate newborn period and in older children.
Mechanism of Action
Sulfonamides are synthetic analogues of para-aminobenzoic acid (PABA). It contains a benzene ring, with a sulfonamide group and a primary amino group next to the sulfur side chain of the sulfonamide group. The sulfonamides act by preventing the bacterial utilization of PABA for the synthesis of folic acid. PABA is essential for bacterial folic acid biosynthesis and with pteridine, it is incorporated to dihydropteroic acid, the immediate precursor of folic acid by dihydropteroic synthase. Sulfonamides competitively inhibit dihyropteroic synthase. Therefore, those microorganisms that must synthesize their own folic acid are vulnerable to the effect of sulfonamide, and those that can utilize preformed folic acid are not affected by these drugs. Moreover, the bacteriostatic effects of sulfonamides can be counteracted by the use of PABA. The sulfonamides are used often with trimethoprim (TMP), which blocks the conversion of dihydrofolic acid to tetrahydrofolic acid. Mammalian cells are not affected by sulfonamides, since they cannot synthesize folic acid and utilize only preformed folic acid.
Sulfamethoxazole
Sulfamethoxazole (SMZ) is an intermediate-acting antibacterial sulfonamide that is also available as a component of the trimethoprim–sulfamethoxazole (TMP–SMZ) 5:1 fixed-ratio combination product.
SMZ is rapidly absorbed following oral administration. It undergoes N4-acetylation and N4-glucuronide conjugation mainly in the liver. The free form is considered to be the microbiologically active form. The acetylated derivative (major metabolite) is not microbiologically active. Seventy percent of SMZ is bound to plasma proteins; of the unbound portion 80% to 90% is in the nonacetylated form.
Free blood concentrations of 5 to 15 mg per dL are considered therapeutically effective for most infections, with blood concentrations of 12 to 15 mg per dL optimal for serious infections. The maximum blood level of total SMZ should not exceed 20 mg per dL. SMZ is widely distributed into most body tissues. It diffuses into cerebrospinal fluid (CSF) with a peak concentration at 8 hours, reaching about 14% of the simultaneous plasma concentration. The drug diffuses into aqueous humor, vaginal fluid, and middle ear fluid. SMZ crosses the placental barrier and is excreted into breast milk.
SMZ and its metabolites are excreted primarily in the urine. The elimination half-life is 10 hours. The unconjugated forms are excreted by tubular secretion, whereas the acetylated drug is excreted by glomerular filtration. In urine, approximately 20% of SMZ present is unchanged drug, 50% to 70% is acetylated derivative, and 15% to 20% is the glucuronide conjugate. Concentrations of SMZ in urine are approximately 3 times concurrent drug–blood concentrations. The half-life of SMZ is prolonged in patients with renal insufficiency (creatinine clearance less than 20 to 30 mL per minute), and reduced doses should be administered in these patients (1).
Clinical use and Dosage
SMZ is indicated for uncomplicated acute and recurrent urinary tract infections caused by susceptible organisms including Escherichia coli, Klebsiella, Enterobacter, and Proteus mirabilis. Currently, the increasing frequency of resistant organisms limits the usefulness of several antibacterial agents including the sulfonamides for the treatment of chronic and recurrent urinary tract infections. The fixed combination TMP–SMZ is preferred over SMZ for most infections (see section on Trimethoprim–Sulfamethoxazole).
Sulfonamides were successfully used, in the past, for the prevention of meningococcal disease in contacts and for the eradication of the chronic meningococcal carrier state. However, because sulfonamide-resistant meningococci are now common, these drugs are usually no longer
suitable for these clinical situations. Rifampin, ceftriaxone, or ciprofloxacin are currently recommended as the chemoprophylactic agents for meningococcal infections in United States. In localized epidemics of sulfonamide-sensitive meningococcal disease, chemoprophylaxis with sulfonamides can be used (2).
suitable for these clinical situations. Rifampin, ceftriaxone, or ciprofloxacin are currently recommended as the chemoprophylactic agents for meningococcal infections in United States. In localized epidemics of sulfonamide-sensitive meningococcal disease, chemoprophylaxis with sulfonamides can be used (2).
The recommended oral dose of SMZ in children older than 2 months of age is 50 to 60 mg per kg initially, followed by 25 to 30 mg per kg every 12 hours (maximum, 75 mg per kg per day). SMZ is not indicated in infants younger than 2 months of age, except in the treatment of congenital toxoplasmosis as an adjunct to pyrimethamine.
Adverse Reactions
SMZ shares the toxic potentials of the sulfonamides. Hemolytic effects include agranulocytosis, aplastic anemia, leukopenia, thrombocytopenia, hemolytic anemia, eosinophilia, and methemoglobinemia. Any sulfonamide may cause hemolysis in patients with glucose 6-phosphate dehydrogenase deficiency. Allergic reactions include anaphylaxis, serum sickness, and conjunctival and scleral injection. Nephrotoxic effects include crystalluria that may cause pain and hematuria. Anuria can occur if the renal pelvis or the ureter becomes completely occluded. Alkalinization of the urine increases solubility as well as enhances the urinary excretion of SMZ and should be used when high doses of SMZ are given. Dermatologic effects include erythema multiforme, Stevens–Johnson syndrome, exfoliative dermatitis, photosensitivity, pruritus, urticaria, and generalized skin eruptions. Gastrointestinal adverse effects include hepatitis, hepatocellular necrosis, pseudomembranous colitis, pancreatitis, nausea, vomiting, diarrhea, anorexia, and abdominal pain. Neurologic complications may include peripheral neuritis, ataxia, vertigo, tinnitus, and headache.
Drug Interactions
SMZ may prolong the prothrombin time in patients who are receiving the anticoagulant warfarin. SMZ may inhibit the hepatic metabolism of phenytoin.
Trimethoprim–Sulfamethoxazole
Clinical Pharmacokinetics
The optimal ratio of TMP and SMZ concentrations for synergy has been determined to be 1 part of TMP and 20 parts of SMZ (3). Thus, available preparations are manufactured in a 1:5 fixed ratio of TMP to SMZ. TMP–SMZ is rapidly and well absorbed from the gastrointestinal tract and may be administered without regard to food. Peak serum concentrations of 1 to 2 μg per mL of TMP and 40 to 60 μg per mL of unbound SMZ are reached at 1 to 4 hours after a single dose containing 160 mg of TMP and 800 mg of SMZ. Steady-state peak serum concentrations after multiple-dose administration are usually 50% higher than those obtained after a single dose. The drug is widely distributed in body tissues and fluids, including CSF, middle ear cavity, sputum, bile, and aqueous humor. Half-life is approximately 8 to 14 hours. TMP is excreted mostly unchanged in urine, with approximately 10% to 30% metabolized to an inactive form. SMZ is primarily metabolized in the liver, with approximately 30% excreted unchanged in urine. Because most drug excretion occurs via the kidney, the dosage of TMP–SMZ should be adjusted for a creatinine clearance of less than 30 mL per minute (4).
Spectrum of Activities
The combination of TMP–SMZ is usually synergistic and bactericidal (5,6). Both drugs affect bacterial folic acid synthesis. SMZ inhibits dihydropteroate synthase, which catalyses the formation of dihydrofolate from PABA. In the subsequent step of the pathway, TMP inhibits dihydrofolate reductase, which catalyses the formation of tetrahydrofolate from dihydrofolate. By inhibiting tetrahydrofolic acid formation, the active form of folic acid, TMP–SMZ inhibits bacterial thymidine synthesis and results in a bactericidal action. Although these steps follow one another and cause a sequential blockade, this does not necessarily explain the aforementioned synergy. A combination of two drugs that have slightly different bacterial spectrums and different resistance profiles among pathogenic bacteria improves the usefulness of the drug combination.
In general, many Enterobacteriaceae, including E. coli, Klebsiella pneumoniae, and P. mirabilis, are susceptible to TMP–SMZ. Salmonella and Shigella species and enterotoxigenic E. coli were previously susceptible to TMP–SMZ. However, the resistant strains have rapidly increased recently worldwide (7,8,9,10). TMP–SMZ is active against Stenotrophomonas maltophilia, which is typically resistant to other classes of broad-spectrum antibiotics (11). Other nonfermentative organisms, including Burkholderia cepacia, Acinetobacter, and Alcaligenes, which often cause nosocomial infections, are frequently susceptible to TMP–SMZ. However, TMP–SMZ has poor activity against Pseudomonas aeruginosa. Staphylococcus aureus, the most common skin pathogen, is also susceptible to TMP–SMZ. TMP–SMZ is also active against Pneumocystis jiroveci (formerly known as P. carinii), Nocardia, Tropheryma whippelii, Isospora species, Cyclospora species, and Toxoplasma gondii.
Mechanism of Resistance
Bacteria may become resistant to TMP–SMZ by several mechanisms, including the development of permeability barriers, efflux pumps, naturally insensitive target enzymes, and genetic alterations in the genes encoding target enzymes. Resistance is transferable. Resistance to SMZ in gram-negative organisms is usually plasmid mediated. Resistance to TMP has been shown to occur by several mechanisms, most often chromosomally mediated, but also involving mutations of bacteria to thymidine-dependent strains, or plasmid-mediated resistance involving altered production or sensitivity of bacterial dihydrofolate reductase. Marked geographic variation in resistance has been demonstrated, with higher incidence typically found in developing countries (12).
Adverse Reactions
The most frequent adverse effects are gastrointestinal intolerance and cutaneous reactions, each occurring in
approximately 3% to 5% of patients. Multiple skin reactions have been described, including a maculopapular rash, urticaria, diffuse erythema, morbilliform rash, erythema multiforme, purpura, and photosensitivity (13,14). These reactions tend to be mild, dose-related, and reversible, and can occasionally be obviated by reduction in dosage without discontinuation of therapy. Fatal hypersensitivity reaction, including Stevens–Johnson syndrome and toxic epidermal necrolysis, rarely occurs. A drug fever with delayed (1 to 2 weeks) onset, often accompanied by a morbilliform rash, may occur. The more severe form of this with multisystem involvement has sometimes been termed the DRESS (drug rash with eosinophilia and systemic symptoms) syndrome. The frequency of adverse effects is substantially higher in immunocompromised patients, particularly in HIV-infected individuals. The rate of adverse reactions to TMP–SMZ in HIV-infected children is approximately 15% (15). Although this is higher than the rate seen in uninfected children, it is substantially lower than the rate seen in HIV-infected adults. The exact mechanisms of increased risk for adverse reactions in this population have not been determined.
approximately 3% to 5% of patients. Multiple skin reactions have been described, including a maculopapular rash, urticaria, diffuse erythema, morbilliform rash, erythema multiforme, purpura, and photosensitivity (13,14). These reactions tend to be mild, dose-related, and reversible, and can occasionally be obviated by reduction in dosage without discontinuation of therapy. Fatal hypersensitivity reaction, including Stevens–Johnson syndrome and toxic epidermal necrolysis, rarely occurs. A drug fever with delayed (1 to 2 weeks) onset, often accompanied by a morbilliform rash, may occur. The more severe form of this with multisystem involvement has sometimes been termed the DRESS (drug rash with eosinophilia and systemic symptoms) syndrome. The frequency of adverse effects is substantially higher in immunocompromised patients, particularly in HIV-infected individuals. The rate of adverse reactions to TMP–SMZ in HIV-infected children is approximately 15% (15). Although this is higher than the rate seen in uninfected children, it is substantially lower than the rate seen in HIV-infected adults. The exact mechanisms of increased risk for adverse reactions in this population have not been determined.
TMP–SMZ may decrease the tubular secretion of creatinine and cause a mild elevation of serum creatinine at standard doses without decreasing the glomerular filtration rate (16,17). This is reversible with drug discontinuation. TMP–SMZ has only rarely been associated with direct nephrotoxicity. Hyperkalemia has been observed in patients taking high-dose TMP–SMZ or in patients with preexisting renal insufficiency taking standard TMP–SMZ dosages (18,19,20). The mechanism could be explained by TMP-induced alteration of transepithelial voltage in distal renal tubule, which results in decreased potassium excretion (21). Hematologic adverse events including anemia, granulocytopenia, megaloblastosis, agranulocytosis, and thrombocytopenia have been reported in children (22,23). Recovery is gradual after the drug is discontinued.
Drug Interaction
SMZ in general can inhibit metabolism of many drugs including warfarin, phenytoin, and sulfonylureas, and it can compete with these agents for binding sites on albumin. Clinically significant exaggeration of anticoagulant effect, CNS toxicity of phenytoin, and hypoglycemia can occur. SMZ use is associated with the increased nephrotoxicity of cyclosporin despite reduction of serum cyclosporin concentrations. SMZ increases the free serum methotrexate fraction. TMP increases serum levels and increases elimination half-life of phenytoin and digoxin.
Clinical Use
TMP–SMZ is used as empiric treatment and prophylaxis for urinary tract infection in children. However, the recent use of antibiotics, hospitalization, and immunosuppression has been implicated as factors contributing to increasing TMP–SMZ resistance among urinary tract isolates in adults (24,25,26). The prevalence of TMP–SMZ resistance among urinary tract isolates in children varies depending on geographic locations. However, limited data indicated an increasing trend of TMP–SMZ resistance up to more than 30% in children with community-acquired urinary tract infection (27,28,29).
Community-associated methicillin-resistant S. aureus (CA-MRSA) has emerged rapidly as a commonly identified cause of skin and soft tissue infections. This has led to consideration for the use of TMP–SMZ in the treatment of CA-MRSA infection. TMP–SMZ is rapidly bactericidal against CA-MRSA in vitro when compared with other orally available antimicrobials (30). The efficacy of TMP–SMZ therapy for CA-MRSA skin and soft tissue infection was reported in a retrospective study (31). It must be emphasized, however, that incision and drainage remains the mainstay treatment of skin and soft tissue infections. In addition, TMP–SMZ is not active against Streptococcus pyogenes, another common cause of skin and soft tissue infections. Given the inability to differentiate microbiologic causes reliably on clinical grounds, monotherapy with TMP–SMZ is not recommended empirically unless CA-MRSA is proven by the culture.
Previously, TMP–SMZ was an effective treatment for otitis media, sinusitis, and community-acquired pneumonia. Emerging resistance among respiratory pathogens, especially S. pneumoniae, has raised serious concerns regarding the use of TMP–SMZ in respiratory tract infections (32). Thus, the use of TMP–SMZ for respiratory tract infections in children requires consideration of local resistance patterns and individual patient factors such as severity of disease and risk for resistance.
TMP–SMZ is useful in the treatment of gastroenteritis caused by Shigella, Salmonella typhi, and traveler’s diarrhea due to enterotoxigenic E. coli. However, it may not be effective in some parts of the world where resistant strains of these organisms are common. Yersinia enterocolitica, Vibrio cholerae, and Aeromonas hydrophila remain susceptible to TMP–SMZ.
TMP–SMZ is the agent of choice for treatment and prophylaxis for P. jiroveci infection in children with malignancy, defects in cell-mediated immunity, and HIV infection. Mutations in the P. jiroveci dihydropteroate synthase gene have been identified in patients taking TMP–SMZ prophylaxis, and this may play a major role in TMP–SMZ treatment failure (33).
Prophylactic use of TMP–SMZ to prevent recurrent bacterial infections in patients with chronic granulomatous disease and other neutrophil-associated immunodeficiencies has been well documented in children (34,35). Selected patients with Wegener granulomatosis may benefit from TMP–SMZ, although the mechanism of action and degree of clinical efficacy in these patients are uncertain (36).
Pediatric Dosage
The pediatric recommended dose is 8 to 12 mg per kg per day of TMP component, or 40 to 60 mg per kg per day of SMZ component, in two divided doses. The daily adult dose is 320 mg of TMP or 1,600 mg of SMZ. For P. jiroveci treatment, the dose should be increased to 20 mg per kg per day of TMP component, or 100 mg per kg per day of SMZ component, in four doses intravenously for 21 days. For prophylaxis in immunocompromised patients, the recommended dose is 5 mg of TMP and 25 mg of SMZ per kg per day in two divided doses.
For prophylaxis against P. jiroveci, the recommended regimen is 150 mg per m2 per day of TMP with 750 mg per m2 per day of SMZ orally, in two divided doses, three times per week on consecutive days (37). The maximum daily dose is 160 mg of TMP and 800 mg of SMZ. The drug may also be given as:
a single daily dose, three times per week on consecutive days,
two divided doses, 7 days per week, or
two divided doses, three times per week on alternate days.
Special Considerations
The indications for P. jiroveci chemoprophylaxis in HIV-infected children are (a) all HIV-infected children younger than 12 months of age regardless of their immunologic status, (b) HIV-infected children older than 12 months of age with severe immune suppression (CDC classification 3), and (c) prior P. jiroveci infection (37). This prophylactic regimen may provide additional protection against toxoplasmosis and recurrent bacterial infections, which are common among HIV-infected individuals with severe immune suppression.
Both TMP and SMZ cross placenta and appear in breast milk, with detectable concentrations found in fetal serum in patients taking the drug. TMP–SMZ is listed in Pregnancy Category C by the US Food and Drug Administration.
Sulfasalazine
Pharmacokinetics
Following oral administration, small intestinal absorption accounts for an absolute sulfasalazine bioavailability of 15%. This fraction is highly protein bound and is subsequently excreted in the urine. However, the majority of an orally administered sulfasalazine dose reaches the colon, where it is metabolized by intestinal flora to sulfapyridine and 5-aminosalicylic acid (5-ASA) or mesalazine (38,39). Plasma concentrations of sulfapyridine and 5-ASA peak at about 10 hours after dosing. The long time to peak concentrations is a function of the transit time to the lower intestine. Sulfapyridine is relatively well absorbed from the colon with bioavailability of 60%. Absorbed sulfapyridine undergoes extensive metabolism in the liver via acetylation, hydroxylation, and glucuronidation. Peak concentrations of sulfapyridine depend on the acetylator status of the patient with slow acetylators having higher concentrations and increased likelihood of adverse events (39). In contrast, 5-ASA is less well absorbed with bioavailability of 10% to 30%. 5-ASA is metabolized by the liver and the intestine to N-acetyl-5-aminosalicylic acid. Acetylation is phenotype independent. Sulfapyridine and 5-ASA and their metabolites are excreted in the urine (38). However, because the majority of 5-ASA stays in the colon, excretion is primarily in the feces as unchanged 5-ASA or as acetyl-5-ASA (40).
Clinical Use
In adults, sulfasalazine is indicated for the treatment of mild to moderate ulcerative colitis and is effective in maintaining disease remission. In addition, it is used as adjunctive therapy with corticosteroids for treatment of severe ulcerative colitis (41). Sulfasalazine may also be active in treating Crohn’s disease of the colon, although it is not effective in maintaining remission. Sulfasalazine has also been used to treat adults with collagenous colitis (42).
Sulfasalazine is considered a disease-modifying antirheumatic drug for rheumatoid arthritis. Sulfasalazine is also used in the treatment of rheumatoid arthritis in patients who fail or are unable to tolerate an adequate trial of nonsteroidal anti-inflammatory drugs (43).
In children younger than 2 years of age, the safety and efficacy of sulfasalazine have not been established. Sulfasalazine is used as a second-line treatment of pauciarticular and polyarticular juvenile rheumatoid arthritis in children (44,45). The use of sulfasalazine in the treatment of patients with systemic-onset juvenile rheumatoid arthritis has been associated with increased incidence of adverse effects, particularly serum sickness. Therefore, sulfasalazine use for those patients should be avoided (45).
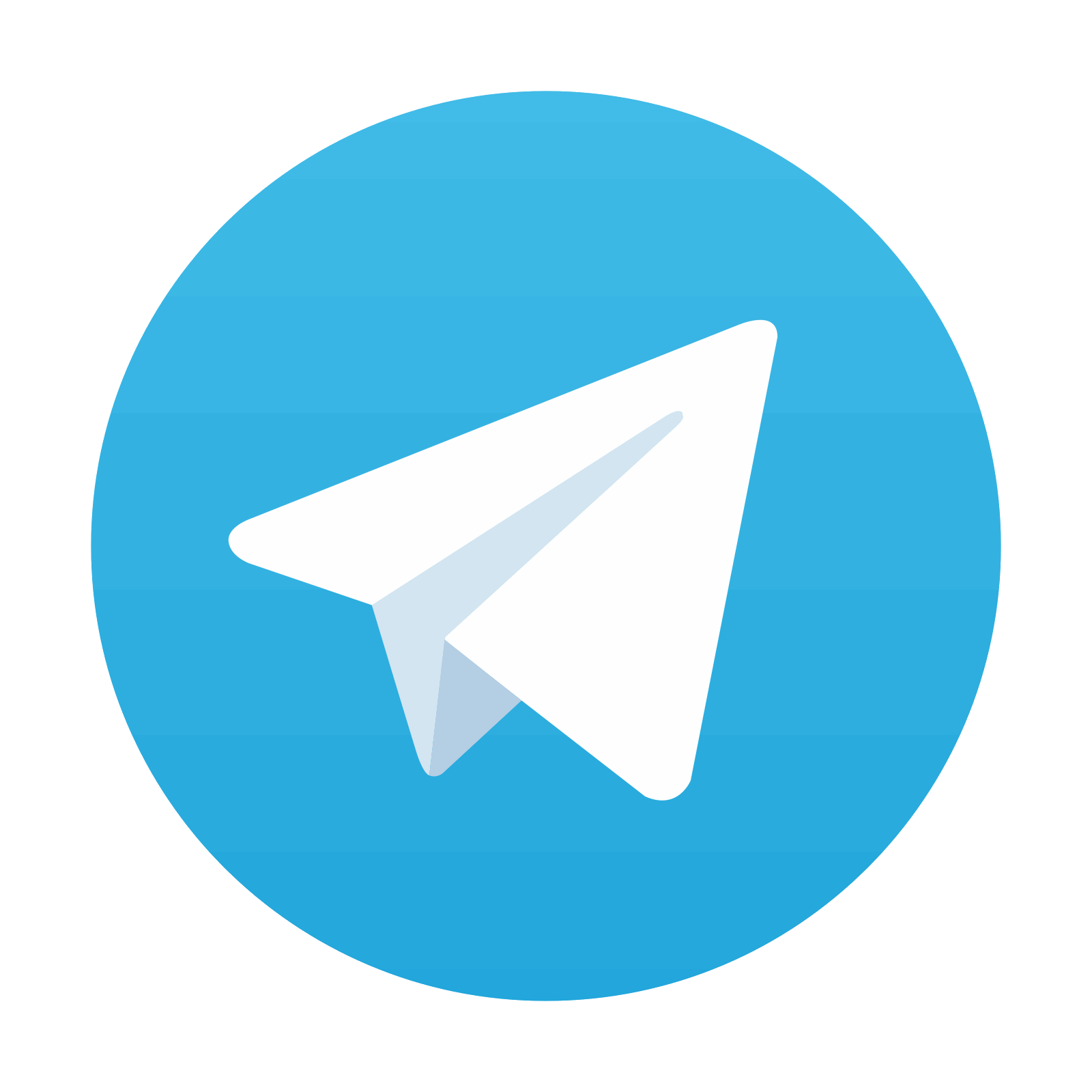
Stay updated, free articles. Join our Telegram channel

Full access? Get Clinical Tree
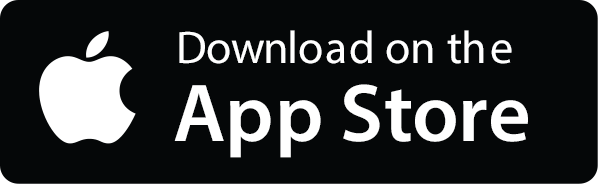
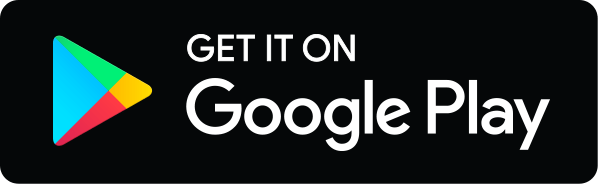