Stem cell based therapies hold promise for the obstetrician and gynecologist. This article reviews the history of stem cells and some of their current applications in gynecology. Currently, mesenchymal and muscle-derived stem cells are being explored for the treatment of urinary and anal incontinence. Potential stem cell treatments include fistula repair, vaginal tissue engineering, and graft material enhancement. Published animal and human pilot studies demonstrate improved histologic and functional outcomes in those receiving stem cells. Transplanted cells may improve function by local engraftment, trophic factors, or modulation of inflammation. Further clinical and safety studies are needed before clinical application.
Dating back to ancient times, the Greeks recognized the human body’s potential for regeneration. Their term for the liver, hepar , was derived from the word hepaomai , “to repair oneself.” Furthermore, Aristotle published observations of animals that possessed the ability to regrow body parts including: the salamander’s ability to regrow its tail and of deer to regrow antlers. Attempts to more actively partake in and control this regenerative capacity on a cellular level can be traced to the early 1900s. In 1912, Alexis Carrel developed an “immortal” cell line from a chick embryo, and continued to culture this cell line for years to follow. In 1963, Ernest A. McCulloch and James E. Till solidified the concept of stem cells in their landmark Nature publication. They transplanted bone marrow cells into irradiated mice and demonstrated that these same cells developed into colonies within the spleen. Gail Martin and Martin Evans, in 1981, developed a line of mouse cells with the capacity to become any tissue in the adult mouse. From this cellular line, the term embryonic stem cells was coined. James Thomson subsequently developed the first line of human embryonic stem cells in 1998. Exciting investigations of medical applications have since exploded and brought us into the realm of research and therapeutic potentials existing today.
The study and use of stem cells has made strides across many fields of medicine. This has included work in hematology, ophthalmology, spinal cord injury, burn therapy, cardiac ischemia, and, more recently, in pelvic floor dysfunction. In this review, we will focus on treatment potentials for urinary and anal incontinence. Much of the existing stem cell research in gynecology has focused on these aspects. Results are promising, but largely limited to animal studies. Clinical, human application remains an exciting goal, but one whose routine, widespread use is premature.
Stem cell sources
Stem cells are defined by their regenerative capacity and their ability to differentiate into 1 or more cell types. Hence, different stem cell types possess different abilities. Toti or pluripotent stem cells, typically embryonic or fetal in source, can differentiate into any cell type or germ cell layer. Multipotent, including mesenchymal stem cells (from blood, bone marrow, placenta, or adipose tissue), are capable of differentiating into multiple, but not all, cell types. Unipotent stem cells, of skin and muscle origin, are the most limited, capable of regeneration within only 1 cell type ( Figure 1 ).

Overall, stem cells can be derived from embryonic and nonembyronic tissue. Human embryonic stem cells (hESC) are obtained from the inner cell mass of blastocysts, are highly plastic and proliferate rapidly. They are theoretically totipotent and capable of differentiating into any germ cell layer. However, hESC require directed differentiation into specific determined high purity cell types. This process is technically rigorous but necessary to improve engraftment and decrease tumorigenicity. In addition, most readers are unaware that immune rejection can occur after hESC transplantation. These cell lines acquire human leukocyte antigens (HLA) as they mature and therefore recipients may need life-long immumosuppression. These special requirements and the ethical debates, surrounding embryonic stem cell limit their current applications in gynecology. Nonembryonic stem cells on the other hand, can be readily derived from sources such as bone marrow, umbilical cord blood, and adipose tissue (multipotent cells), or from muscle (multi- and unipotent populations). The majority of these cell types can be autologously derived from the gynecologic patient. Adult stem cells can be identified by a variety of markers including CD45, CD34, Pax7, and MyoD. In preparation, cells are selected from biopsied tissue (ie, bone marrow, adipose, or muscle), expanded in culture, and passaged to achieve confluency until desired cell transplant numbers are achieved. Within animal studies, an identifying marker (ie, green fluorescent protein [GFP], BrdU, or PKH-26) is typically transduced to cells to assess for survival and/or integration.
Origins of stem cell use in gynecology
Stem cell therapy for the treatment of urinary and anal incontinence is based on principles of skeletal muscle regeneration. The urethral and external anal sphincters both contain skeletal muscle that contributes to effective continence. However, age-related changes, injury, and trauma can all lead to compromise in function. Skeletal muscle possesses the ability of inherent regeneration based on the presence of satellite cells. Satellite cells exist below the basal lamina in a dormant state, and on injury are activated and act as native reparative cells. Activated satellite cells produce myoblasts—unipotent muscle progenitor stem cells ( Figure 2 ). Myoblasts fuse to form myotubes, and myotubes are packaged into sarcomeres and align to form myofibers. This process ultimately leads to muscle regeneration. Unfortunately, in the native state, the portion of satellite cells within skeletal muscle is quite small—unable to compensate for large or chronic injuries alone.

Some of the earliest research using myoblasts was in the field of Duchenne Muscular Dystrophy. The concept of muscle biopsy, in vitro culture, and subsequent myoblast transplant to treat this degenerative disorder was proposed in 1978. Multiple animal studies ensued through the 1980s, and in 1990 the first human myoblast transplant occurred. Unfortunately, success was limited, possibly because of the nature of this systemic degenerative disorder and large burden of muscular dysfunction. In addition, transplanted cells presented an overwhelming challenge of rapid cell death and migration. Incontinence models built on this concept, and possibly because they involve a smaller muscle burden, have demonstrated greater promise. Proof of concept of myoblast transplantation in a rat stress incontinence model was published in 2000. Chancellor et al demonstrated histologic evidence of cell survival and fusion of myoblasts to myotubes after periurethral injection. Translation to anal incontinence models ensued. Kang et al and Lorenzi et al published some of the earliest work transplanting stem cells into injured rat anal sphincters. Improved function was demonstrated.
Origins of stem cell use in gynecology
Stem cell therapy for the treatment of urinary and anal incontinence is based on principles of skeletal muscle regeneration. The urethral and external anal sphincters both contain skeletal muscle that contributes to effective continence. However, age-related changes, injury, and trauma can all lead to compromise in function. Skeletal muscle possesses the ability of inherent regeneration based on the presence of satellite cells. Satellite cells exist below the basal lamina in a dormant state, and on injury are activated and act as native reparative cells. Activated satellite cells produce myoblasts—unipotent muscle progenitor stem cells ( Figure 2 ). Myoblasts fuse to form myotubes, and myotubes are packaged into sarcomeres and align to form myofibers. This process ultimately leads to muscle regeneration. Unfortunately, in the native state, the portion of satellite cells within skeletal muscle is quite small—unable to compensate for large or chronic injuries alone.

Some of the earliest research using myoblasts was in the field of Duchenne Muscular Dystrophy. The concept of muscle biopsy, in vitro culture, and subsequent myoblast transplant to treat this degenerative disorder was proposed in 1978. Multiple animal studies ensued through the 1980s, and in 1990 the first human myoblast transplant occurred. Unfortunately, success was limited, possibly because of the nature of this systemic degenerative disorder and large burden of muscular dysfunction. In addition, transplanted cells presented an overwhelming challenge of rapid cell death and migration. Incontinence models built on this concept, and possibly because they involve a smaller muscle burden, have demonstrated greater promise. Proof of concept of myoblast transplantation in a rat stress incontinence model was published in 2000. Chancellor et al demonstrated histologic evidence of cell survival and fusion of myoblasts to myotubes after periurethral injection. Translation to anal incontinence models ensued. Kang et al and Lorenzi et al published some of the earliest work transplanting stem cells into injured rat anal sphincters. Improved function was demonstrated.
Potential benefits of stem cell use in gynecology
Use of stem cells for the treatment of urinary and anal incontinence holds great promise especially when considering that existing options for both remain limited. Stress urinary incontinence (SUI) can be attributed to either loss of urethral support and resulting hypermobility, intrinsic deficiency within the urethral sphincter (ISD), or a combination of both. To date SUI can be effectively treated with surgical placement of a midurethral sling. Treatment of sphincteric dysfunction presents a greater challenge. Urethral bulking can help to restore coaptation, but long-term efficacy is lacking and local tissue reaction can occur. Effective treatment options for anal incontinence are even more sparse. Physical therapy can provide modest benefit. Sphincteroplasty, bulking agents, and/or artificial sphincters have poor long-term outcomes and can pose significant risks of infection.
As both SUI and anal incontinence (AI) can result from loss of muscular integrity and function, rebuilding muscle from a cellular level represents an ideal treatment concept. Numerous animal, along with a few human studies, have, in fact, demonstrated functional measures of success. As stem cell research has expanded, theories accounting for the mechanism of action have expanded as well. Initially, the theory was that transplanted cells would engraft, differentiate, and directly lead to functional regeneration. Potential explanations now include: (1) direct stem cell engraftment and muscle regeneration; (2) localized bulking impact; (3) trophic effect because of stem cell growth factor secretion; and (4) immune modulation affording improved tissue healing. These will be addressed in further detail below.
SUI
Stem cell research for the treatment of SUI is rapidly expanding with overall encouraging results. Bone marrow (BMSC) and adipose-derived mesenchymal stem cells (ADSC) are the most common cell populations used in murine transplants, although muscle-derived stem cells (MDSC) (cultured from patient thigh or deltoid biopsies) have typically been used in human studies. The ideal cell population has yet to be determined.
Transplantation method of cells has also varied, and has included intraurethral, periurethral, and intravenous (IV) routes. Localized injections make inherent sense, IV injection methods, on the other hand, less so. The method of IV transplantation is based on stem cell homing ability. For example, after simulated injury with pelvic floor distention, IV injected stem cells were found to migrate and ultimately accumulate within the injured pelvis. Benefits of IV administration could include: a less invasive route when compared with multiple direct tissue injections, and/or could potentially maximize cell delivery to the location of greatest impact if they are consistently found to, in fact, home to the site of injury. This has yet to be clarified in the literature. In addition, studies have reported successful immune modulation with IV injected mesenchymal cells. Several clinical trials are examining IV mesenchymal infusions to treat a wide range of autoimmune disorders. Although incontinence research has not specifically studied immune modulation, decreases in inflammation could improve tissue healing.
Another point of variation among SUI studies is the method of injury used to create animal models of urinary incontinence. This includes pudendal or sciatic nerve transection, crush nerve injury, urethral cyro- or chemoinjury, and vaginal distention. Precise comparisons are difficult; still, studies have shown definite trends toward improved outcome measures.
For this review, we attempt to evaluate studies with similar methodologies in conjunction. To begin, 2 studies, Xu et al in 2010 and Kim et al in 2011, demonstrated full restoration of leak point pressures (LPP) in a rat model after urethral injections of BMSC postpudendal nerve transection. Kinebuchi et al further demonstrated a trend toward LPP restoration when BMSC were injected into rat urethras after urethrolysis and localized toxic injection. Although results did not reach significance, it is important to note that this last model of injury was extensive and may represent a more severe presentation of incontinence. Furthermore on histologic evaluation, Kinebuchi et al did demonstrate significantly more striated muscle in the transplantation group than in controls.
Three recent studies that used ADSC for transplant have also shown benefit. In the first, 20 rats were transplanted after pudendal crush injury. Follow-up measures demonstrated increased LPP, closure pressure, and functional urethral lengths compared with controls. Lin et al demonstrated that rodents receiving stem cell transplants had reduced voiding dysfunction (33% vs 80% in controls) after vaginal distension. Zhao et al found that inclusion of encapsulated nerve growth factor (NGF) with ADSC transplant successfully increased cell survival, differentiation, and neuronal density.
Other researchers have begun to bridge the gap to human studies. In the earliest publication, 8 women received autologous MDSCs (thigh muscle biopsy) in up to 4 circumferential periurethral injections. Of the 5 women available for follow up, all had improvement (although 2 eventually underwent midurethral sling placement), and 1 reported total continence. Lee et al demonstrated >50% subjective improvement in 26 of 39 women with SUI who were injected transurethrally at 4 and 8 o’clock with human umbilical cord stem cells. And, most recently, 12 women with severe SUI and a fixed urethra received injection of muscle progenitor cells derived from autologous deltoid biopsy. Cells were transplanted via single injection under endoscopic guidance. Three women were dry at 12 months based on self-reported diary and pad test measures. Seven women had improved pad tests, but not improved diary measures. Two women demonstrated slight worsening of incontinence. There were no adverse events encountered in any human study. Limitations of human and animal studies include small sample sizes and/or lack of control groups and randomization ( Table 1 ).
Author | Cell source | Methods | Results/conclusions | Complications |
---|---|---|---|---|
Kim et al |
|
|
|
|
Cruz et al | Rat BMSC labeled with GFP |
|
|
None |
Xu et al | Rat MDSC labeled w GFP ± Fibrin Glue |
|
|
|
Corcos et al | Rat BMSC |
|
Resolution of LPP to baseline in Transplant group | None |
Wu et al | Rat ADSC |
|
|
None |
Lim et al | HUCB with fluorescent label |
|
|
None |
Lin et al | Rat ADSC, labeled |
|
|
None |
Zhao et al | Rat ADSC rat ± nerve growth factor (NGF) ± encapsulated polylactic-coglycolic acid |
|
|
None |
Kinebuchi et al |
|
|
|
None |
Imamura et al | Rabbit BMSC |
|
LPP higher in transplant vs control at 2 wk | None |
Fu et al | Rat ADSC |
|
Max bladder capacity, LPP, and muscle thickness increased in transplant group | None |
Carr et al | Autologous MDSC |
|
|
None |
Lee et al | Human cord blood |
|
|
None |
Sebe et al | Autologous muscle progenitor cells |
|
|
None |
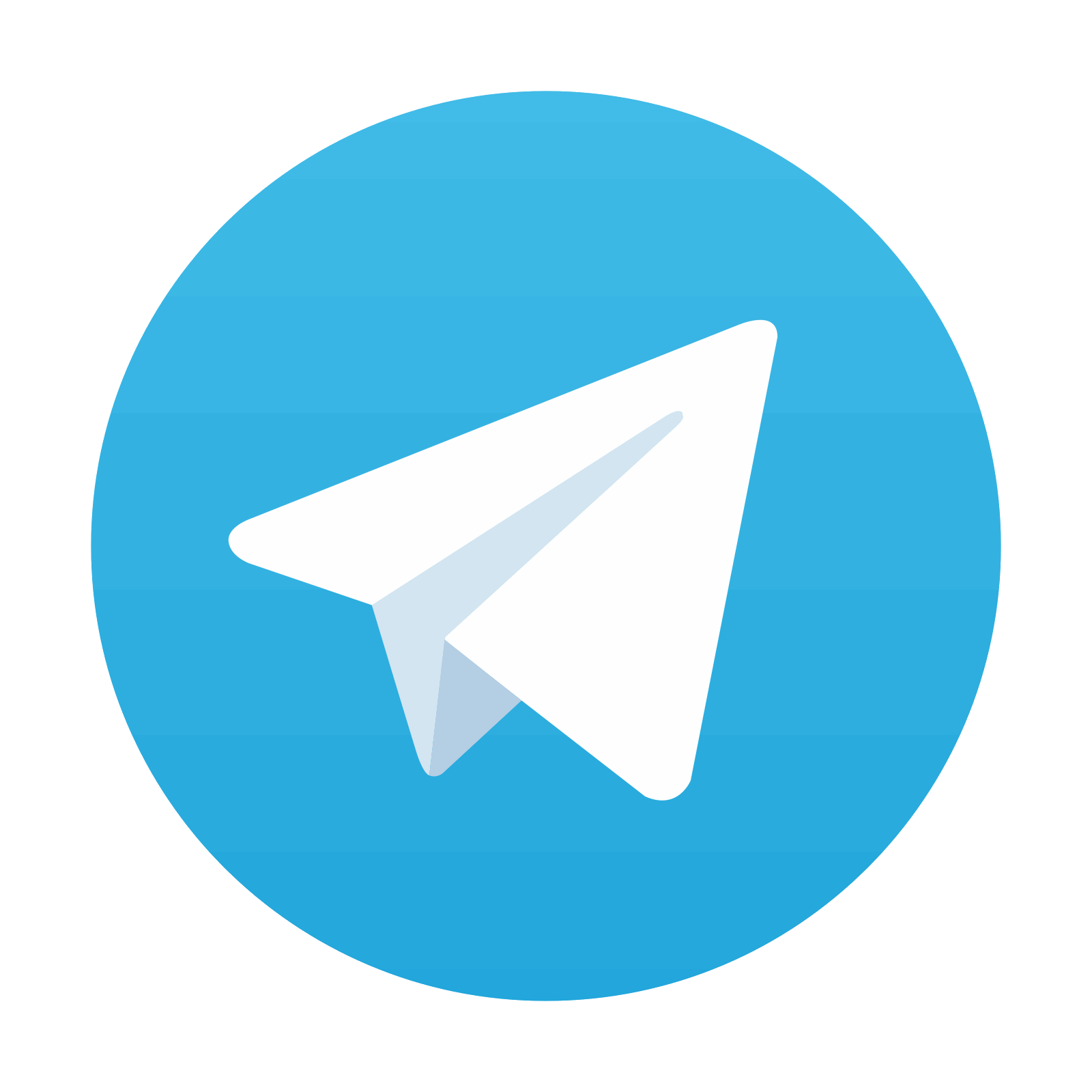
Stay updated, free articles. Join our Telegram channel

Full access? Get Clinical Tree
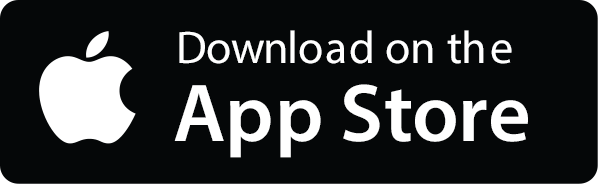
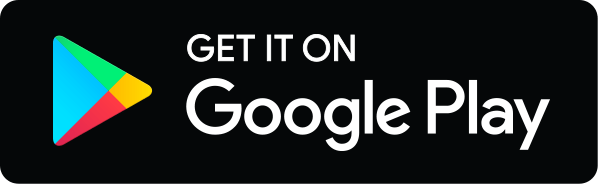