As the notochord and neural tube form in the third week, the intraembryonic mesoderm lateral to these structures thickens to form two longitudinal columns of paraxial mesoderm ( Fig. 14.1 A and B ). Toward the end of the third week, these dorsolateral columns, located in the body (trunk), become segmented into condensed blocks of mesoderm (somites) (see Fig. 14.1 C ). Externally, the somites appear as bead-like elevations along the dorsolateral surface of the embryo (see Chapter 5 , Fig. 5.6 A to D ). Each somite differentiates into two parts (see Fig. 14.1 D and E ):
- •
The ventromedial part is the sclerotome. Its cells form the vertebrae and ribs.
- •
The dorsolateral part is the dermomyotome. Cells from its myotome region form myoblasts (primordial muscle cells), and those from its dermatome region form the dermis (fibroblasts) .

Development of Bone and Cartilage
At the end of the fourth week, the sclerotome cells form a loosely woven tissue called mesenchyme (embryonic connective tissue), which has bone-forming capacity. Bones first appear as condensations of mesenchymal cells that form bone models. C ondensation (dense packing) marks the beginning of selective gene activity, which precedes cell differentiation ( Fig. 14.2 ). Most flat bones develop in mesenchyme within preexisting membranous sheaths; this type of osteogenesis is called membranous ( intramembranous ) bone formation . Mesenchymal models of most limb bones are transformed into cartilage bone models, which later become ossified by endochondral bone formation .

Proteins encoded by the Hox genes, bone morphogenetic proteins (BMP5 and BMP7), growth factor GDF5, members of the transforming growth factor-β (TGF-β) superfamily, vascular endothelial growth factor (VEGF), and other signaling molecules are endogenous regulators of chondrogenesis and skeletal development. Lineage commitment of skeletal precursor cells to chondrocytes and osteoblasts is determined by β-catenin levels . β-Catenin in the canonical Wnt signaling pathway plays a critical role in the formation of cartilage and bone .
Histogenesis of Cartilage
Cartilage develops from mesenchyme during the fifth week. In areas where cartilage is programmed to develop, the mesenchyme condenses to form chondrification centers . The mesenchymal cells differentiate into prechondrocytes and then into chondroblasts , which secrete collagenous fibrils and ground substance (extracellular matrix) . Subsequently, collagenous or elastic fibers, or both, are deposited in the intercellular substance or matrix. Three types of cartilage are distinguished according to the type of matrix that is formed:
- •
Hyaline cartilage , the most widely distributed type (e.g., synovial joints)
- •
Fibrocartilage (e.g., intervertebral discs)
- •
Elastic cartilage (e.g., auricles of the external ears)
Histogenesis of Bone
Bone primarily develops in two types of connective tissue, mesenchyme and cartilage, but it can also develop in other connective tissues (e.g., patella develops in a tendon). Like cartilage, bone consists of cells and an organic intercellular substance (bone matrix) that comprises collagen fibrils embedded in an amorphous component. Studies of the cellular and molecular events during embryonic bone formation suggest that osteogenesis and chondrogenesis are programmed early in development and are independent events under the influence of vascular changes.
Intramembranous Ossification
Intramembranous ossification occurs in mesenchyme that has formed a membranous sheath ( Fig. 14.3 ) and produces osseous tissue without prior cartilage formation. The mesenchyme condenses and becomes highly vascular. Precursor cells differentiate into osteoblasts (bone-forming cells) and begin to deposit unmineralized matrix rich in type I collagen (osteoid). Wnt signaling is a key factor in osteoblast differentiation. Calcium phosphate is then deposited in osteoid tissue as it is organized into bone. Bone osteoblasts are trapped in the matrix and become osteocytes .

At first, new bone has no organized pattern. Spicules of bone soon become organized and coalesce into lamellae (layers). Concentric lamellae develop around blood vessels, forming osteons (Haversian systems). Some osteoblasts remain at the periphery of the developing bone and continue to lay down lamellae, forming plates of compact bone on the surfaces. Between the surface plates, the intervening bone remains spiculated or spongy. This spongy environment is somewhat accentuated by the action of osteoclast cells that reabsorb bone. Osteoclasts are multinucleated cells with a hematopoietic origin. In the interstices of spongy bone, the mesenchyme differentiates into bone marrow . Hormones and cytokines regulate remodeling of bone by the coordinated action of osteoclasts and osteoblasts.
Endochondral Ossification
Endochondral ossification (cartilaginous bone formation) is a type of bone formation that occurs in preexisting cartilaginous models ( Fig. 14.4 ). In a long bone, for example, the primary center of ossification appears in the diaphysis (part of a long bone between its ends), which forms the shaft of a bone (e.g., humerus). At this center of ossification, chondrocytes (cartilage cells) increase in size (hypertrophy) and create a matrix of high-X-collagen concentration, the matrix becomes calcified, and the cells die. The hypertrophic chondrocytes also express VEGF, which acts as a chemotactic factor, attracting early hematopoietic progenitor cells and vascular endothelial cells.

Concurrently, a thin layer of bone is deposited under the perichondrium surrounding the diaphysis, and the perichondrium becomes the periosteum . Invasion by vascular connective tissue from blood vessels surrounding the periosteum also breaks up the cartilage. Osteoblasts reach the developing bone from these blood vessels. This process continues toward the epiphyses (ends of the bones). The spicules of bone are remodeled by the action of osteoclasts and osteoblasts. The transcription factor SOX9 and the coactivator-associated arginine methyltransferase 1 (CARM1) regulate osteochondral ossification.
Lengthening of long bones occurs at the diaphyseal-epiphyseal junction . Lengthening of bone depends on the epiphyseal cartilage plates (growth plates), whose chondrocytes proliferate and participate in endochondral bone formation (see Fig. 14.4 E ). Toward the diaphysis, the cartilage cells hypertrophy (increase in size), and the matrix becomes calcified. The spicules of the bone are isolated from each other by vascular invasion from the marrow or medullary cavity of long bone (see Fig. 14.4 E ). Bone is deposited on these spicules by osteoblasts; resorption of the bone keeps the spongy bone masses relatively constant in length and enlarges the medullary cavity.
Ossification of limb bones begins at the end of the embryonic period (approximately 56 days). Thereafter, it makes demands on the maternal supply of calcium and phosphorus. Pregnant women are advised to maintain an adequate intake of these elements to preserve healthy bones and teeth.
At birth, the diaphyses are largely ossified, but most of the epiphyses are still cartilaginous. Secondary ossification centers appear in the epiphyses in most bones during the first few years after birth. The epiphyseal cartilage cells hypertrophy, and there is invasion by vascular connective tissue. Ossification spreads radially, and only the articular cartilage and a transverse plate of cartilage (epiphyseal cartilage plate ) remain cartilaginous (see Fig. 14.4 E ). On completion of growth, the cartilage plate is replaced by spongy bone, the epiphyses and diaphysis are united, and no further elongation of the bone occurs.
In most bones, the epiphyses fuse with the diaphysis by the age of 20 years. Growth in the diameter of a bone results from the deposition of bone at the periosteum (see Fig. 14.4 B ) and from resorption on the internal medullary surface. The rate of deposition and resorption is balanced to regulate the thickness of the compact bone and the size of the medullary cavity. The internal reorganization of bone continues throughout life. The development of irregular bones is similar to that of the epiphyses of long bones. Ossification begins centrally and spreads in all directions.
Rickets is a disease in children attributable to vitamin D deficiency . It is a major public health concern in many parts of the world. Vitamin D is required for calcium absorption by the intestine. The resulting deficiency of calcium and phosphorus causes disturbances of ossification of the epiphyseal cartilage plates because they are not adequately mineralized, and there is disorientation of cells at the metaphysis . The wrist joint and costochondral junctions are usually enlarged. The limbs are shortened and deformed, with severe bowing of the limb bones. Rickets may also delay closure of the fontanelles of the cranial bones in infants (see Fig. 14.9 A and B ). Hereditary vitamin D–resistant rickets results from mutations in the vitamin D receptor.
Development of Joints
Joints begin to develop with the appearance of the joint interzone within the continuous cartilage bone model during the sixth week. The cells in the interzone begin to flatten and form a separation at the joint location. Factors involved in the early formation of the interzone include Wnt-14 and Noggin. By the end of the eighth week, the joints resemble the adult structures ( Fig. 14.5 ). Joints are classified as fibrous joints , cartilaginous joints , and synovial joints . Joints with little or no movement are classified according to the type of material holding the bones together; for example, the bones of fibrous joints are joined by fibrous tissue. Molecular studies show that a distinct cohort of progenitor cells expressing TGF-β receptor 2 at prospective joint sites contributes to formation of the synovial joints and articular cartilages .

Fibrous Joints
During the development of fibrous joints, the interzonal mesenchyme between the developing bones differentiates into dense fibrous tissue (see Fig. 14.5 D ). For example, the sutures of the cranium are fibrous joints (see Fig. 14.9 ).
Cartilaginous Joints
During the development of cartilaginous joints, the interzonal mesenchyme between the developing bones differentiates into hyaline cartilage (e.g., costochondral joints) or fibrocartilage (pubic symphysis; see Fig. 14.5 C ).
Synovial Joints
During the development of synovial joints (e.g., knee joint), the interzonal mesenchyme between the developing bones differentiates as follows (see Fig. 14.5 B ):
- •
Peripherally, the interzonal mesenchyme forms the joint capsule and other ligaments.
- •
Centrally, the mesenchyme undergoes cavitation, beginning in late embryogenesis to the postnatal period, and disappears, and the resulting space becomes the fluid filled joint cavity (synovial cavity).
- •
Where it lines the joint capsule and articular surfaces, the mesenchyme forms the synovial membrane , which secretes synovial fluid and is a part of the joint capsule (fibrous capsule lined with synovial membrane).
Probably as a result of joint movements, the mesenchymal cells subsequently disappear from the surfaces of the articular cartilages. An abnormal intrauterine environment restricting embryonic and fetal movements may interfere with limb development and cause joint fixation.
Development of Axial Skeleton
The axial skeleton is composed of the cranium (skull), vertebral column, ribs, and sternum. During the fourth week, cells in the sclerotomes surround the neural tube (primordium of spinal cord) and notochord , the structure around which the primordia of the vertebrae develop ( Fig. 14.6 A ). This positional change of the sclerotomal cells is caused by the differential growth of the surrounding structures and not by active migration of sclerotomal cells. The protocadherins Fat4 and Dchs 1 signaling mediate planar cell polarity and control early chondrogenesis in the developing vertebrae. TBX6 , Hox , and PAX genes regulate the patterning and regional development of the vertebrae along the anterior-posterior axis.

Development of Vertebral Column
During the precartilaginous or mesenchymal stage , mesenchymal cells from the sclerotomes are found in three main areas (see Fig. 14.6 A ): around the notochord, surrounding the neural tube, and in the body wall. In a frontal section of a 4-week embryo, the sclerotomes appear as paired condensations of mesenchymal cells around the notochord (see Fig. 14.6 B ). Each sclerotome consists of loosely arranged cells cranially and densely packed cells caudally.
Some densely packed cells move cranially, opposite the center of the myotome (muscle plate), where they form the intervertebral disc ( Fig. 14.6 C and D ). The remaining densely packed cells fuse with the loosely arranged cells of the immediately caudal sclerotome to form the mesenchymal centrum, the primordium of the body of a vertebra. Thus, each centrum develops from two adjacent sclerotomes and becomes an intersegmental structure.
The nerves lie close to the intervertebral discs, and the intersegmental arteries lie on each side of the vertebral bodies. In the thorax, the dorsal intersegmental arteries become the intercostal arteries .
The notochord degenerates and disappears where it is surrounded by the developing vertebral bodies. Between the vertebrae, the notochord expands to form the gelatinous center of the intervertebral disc, the nucleus pulposus (see Fig. 14.6 D ). This nucleus is later surrounded by circularly arranged fibers that form the annulus fibrosus . The nucleus pulposus and annulus fibrosus form the intervertebral disc . The mesenchymal cells that surround the neural tube form the neural arch , which is the primordium of the vertebral arch (see Fig. 14.6 C ). The mesenchymal cells in the body wall form costal processes , which form the ribs in the thoracic region.
Remnants of the notochord may persist and form a chordoma , a rare neoplasm (tumor). Approximately one third of these slow-growing malignant tumors occurs at the base of the cranium and extends to the nasopharynx. Chordomas infiltrate bone and are difficult to remove. Chordomas also develop in the lumbosacral region. Surgical resection has provided long-term, disease-free survival for many patients.
Cartilaginous Stage of Vertebral Development
During the sixth week, chondrification centers appear in each mesenchymal vertebra ( Fig. 14.7 A and B ). The two centers in each centrum fuse at the end of the embryonic period to form a cartilaginous centrum. Concomitantly, the centers in the neural arches fuse with each other and the centrum. The spinous and transverse processes develop from extensions of chondrification centers in the neural arch. Chondrification spreads until a cartilaginous vertebral column is formed.

Bony Stage of Vertebral Development
Ossification of typical vertebrae begins during the seventh week and ends by the 25th year. There are two primary ossification centers , ventral and dorsal, for the centrum ( Fig. 14.7 C ). These centers soon fuse to form one center. Three primary centers are present by the eighth week: one in the centrum and one in each half of the neural arch.
Ossification becomes evident in the neural arches during the eighth week. Each typical vertebra consists of three bony parts connected by cartilage: a vertebral arch, a body, and transverse processes (see Fig. 14.7 D ). The bony halves of the vertebral arch usually fuse during the first 3 to 5 years. The arches first unite in the lumbar region, and union progresses cranially. The vertebral arch articulates with the centrum at cartilaginous neurocentral joints , which permit the vertebral arches to grow as the spinal cord enlarges. These joints disappear when the vertebral arch fuses with the centrum during the third to sixth years.
Five secondary ossification centers appear in the vertebrae after puberty:
- •
One for the tip of the spinous process
- •
One for the tip of each transverse process
- •
Two anular epiphyses , one on the superior and one on the inferior rim of the vertebral body (see Fig. 14.7 E and F )
The vertebral body is a composite of the anular epiphyses and the mass of bone between them. The vertebral body includes the centrum, parts of the vertebral arch, and the facets for the heads of the ribs. All secondary centers unite with the rest of the vertebrae at approximately 25 years of age. Exceptions to the typical ossification of vertebrae occur in the atlas or C1 vertebra, axis or C2 vertebra, C7 vertebra, lumbar vertebrae, sacrum, and coccyx.
The Notch signaling pathways are involved in the patterning of the vertebral column. Severe congenital birth defects, including VACTERL syndrome ( v ertebral, a nal, c ardiac, t racheal, e sophageal, r enal, and l imb birth defects) and CHARGE syndrome (coloboma of the eye, heart defects, including tetralogy of Fallot, patent ductus arteriosus, and ventricular or atrial septal defect), are associated with mutation in Notch-pathway genes. Minor defects of the vertebrae are common but usually have little clinical importance.
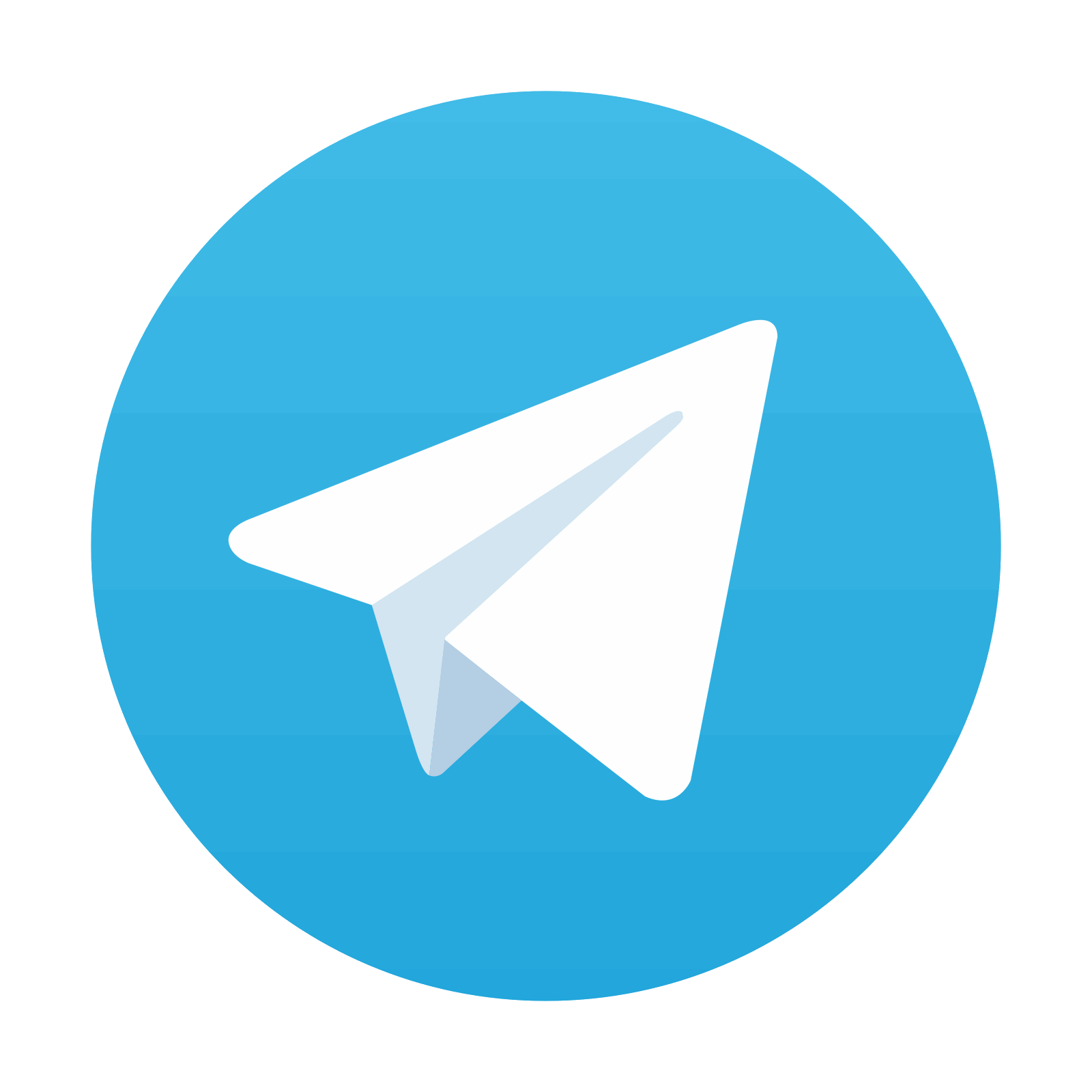
Stay updated, free articles. Join our Telegram channel

Full access? Get Clinical Tree
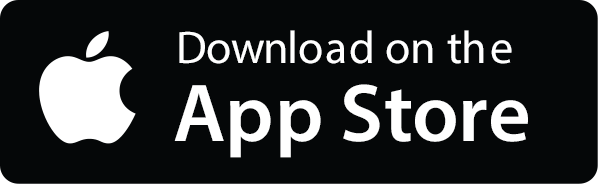
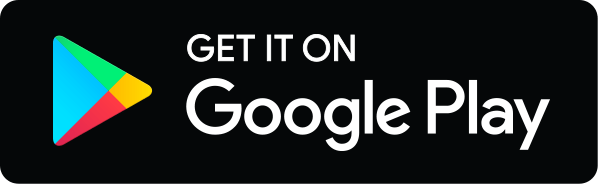