Short stature (usually defined as height >2 standard deviations [SD] below the mean for age) and growth failure (a subnormal height velocity that leads to a decline in growth percentiles, usually a height velocity >1.5 SD below the mean for age) are symptoms, not diseases . Of the 2, short stature is more likely to be noticed but growth failure is more likely to be pathologic.
Short stature may represent a normal variant or may be a signal of serious physical or emotional illness. Because linear growth is a crucial component of childhood, growth is in many ways an index of childhood well-being. Illnesses, even those not involving aberrations of growth-regulating hormones, often interfere with growth. Therefore, the measurement and charting of heights sequentially on standardized growth charts constitute a central part of a child’s medical evaluation and the finding of short stature, particularly if associated with a subnormal height velocity, deserves close attention and appropriate evaluation.
(See Nelson Textbook of Pediatrics, p. 2642.)
Definition
Short stature is defined as height more than 2 SD below the mean for age, which is at the 2.3 percentile ( Fig. 43.1 A, B ). On growth charts (and in clinical practice) it is more common to define short stature as height below the 3rd percentile for age, which is −1.88 SD. Implicit in this definition is the understanding that height is a normally distributed characteristic; therefore, a proportion of normal individuals have heights >2 SD from the mean. Since height is strongly heritable (i.e., a large proportion of the observed variation between individuals is hereditary), stature that is inappropriately low for the child’s genetic endowment may also be a cause for concern.

Growth failure is defined as a subnormal rate of growth, or a height velocity that is below the norm for that age. Since sustained height velocity below the 25th percentile is insufficient to maintain a child’s position on the growth chart, growth failure is sometimes defined as a height velocity below the 25th percentile for age. Other authorities prefer to use >1.5 SD below the average height velocity for that age as the cutoff.
Short stature should be distinguished from “failure to thrive.” The latter term refers primarily to poor weight gain in infants and young children (although linear growth may be secondarily affected), whereas short stature refers primarily to subnormal linear growth in childhood and adolescence.
Normal Growth
Height is highly heritable, but the genetic program underlying this regulation is not fully understood. The growth of individual organs as well as the growth of the skeleton as a whole is regulated by negative feedback mechanisms that slow and eventually stop growth as organs and the organism reach their final size. This gradual deceleration of growth with age does not appear to be driven primarily by changes in the endocrine system since levels of growth hormone (GH) and the insulin-like growth factors remain elevated even as growth slows in the latter part of puberty. The final height and growth pattern of any given individual are affected by subtle variations in large numbers of many genes, while more significant variants in individual genes cause the various genetic forms of short stature and skeletal dysplasias .
Fetal Growth and Birth Size
A human being experiences his or her most rapid linear growth in the prenatal period (growing from near zero to about 50 cm in length in just 9 months). While genetic factors play a major role in postnatal growth, fetal growth and birth size mainly reflect maternal and placental factors, including maternal or uterine size, parity, multiparity, nutrition, and placental function. Many congenital disorders such as Turner syndrome and congenital GH deficiency that markedly stunt postnatal growth have only minimal effects on prenatal growth and birth size. Therefore, birth size is generally a poor predictor of the eventual growth pattern in most children. An exception is the neonate who is small for gestational age (SGA) as a result of intrauterine growth restriction (IUGR) . While most infants with IUGR (caused by nutritional problems or poor placental function) show catch-up growth (a period of rapid growth that occurs spontaneously after relief from the adverse intrauterine condition that had suppressed the rate of growth), 10-20% remain shorter than expected beyond infancy and early childhood, making IUGR 1 of the possible causes of childhood short stature.
Postnatal Growth Patterns
Infancy is also a period of relatively rapid growth (faster than at any other time in postnatal life). Growth then gradually slows as the infant gets older, declining to its lowest point just before puberty, before accelerating again during the pubertal growth spurt, and finally ending with the completion of linear growth about 5 years after the onset of puberty ( Table 43.1 and Fig. 43.2 ).
Age Interval | Average Height Velocity |
---|---|
Prenatal | 66 cm/yr |
0-1 yr | 25 cm/yr |
1-2 yr | 12 cm/yr |
2-3 yr | 8 cm/yr |
3-5 yr | 7 cm/yr |
5–onset of puberty | 5-6 cm/yr |
Pubertal growth spurt | Girls 8-12 cm |
Boys 10-14 cm |

Since birth length is mostly determined by maternal and placental factors, size at birth may not reflect the infant’s genetic growth potential. It is during the 1st 2 years of life that infants gradually transition from their birth size to their own genetically determined height potential. Therefore, it is normal for infants to shift linear growth percentiles during this period; 65% of infants will exhibit such shifts, moving up or down on the growth chart. By 24 months, these shifts are complete and most children have entered a specific “growth channel” or linear growth percentile in relation to peers and any significant deviation from this channel should evince concern.
After a period of slow but steady growth during childhood, many children experience a further “prepubertal dip” in height velocity, reaching a nadir just before the onset of puberty. Height velocity then accelerates once again as puberty advances. This acceleration of growth during puberty reaches a peak known as the “pubertal growth spurt.” The timing of the pubertal growth spurt differs between girls and boys. Females generally begin pubertal development at 10-11 years of age and their pubertal growth spurt starts coincidentally with breast development and peaks before menarche. For females with an average tempo of puberty, peak growth velocity (8-9 cm/yr) is reached at 11-12 years of age. After menarche (which generally occurs 2-2.5 years after the onset of puberty), the growth rate declines and growth finally stops about 2 years after menarche, with girls gaining an average of 7 cm in height after menarche.
In males, testicular enlargement is generally the 1st sign of puberty and occurs at approximately 11.5 years on average (range, 9-14.3 years). In males with an average tempo of pubertal development, peak growth velocity occurs about 2 years after the onset of puberty (so, later than girls in absolute terms, as well as in terms of the stage of puberty) at approximately 13-14 years, with an average rate of 10.3 cm (4 inches)/yr. It is worth noting that prepubertal males and females grow at very similar rates; the ultimate taller stature of males relative to females is mostly the result of a longer period of growth and a higher peak growth velocity during puberty.
These are the average timings of puberty and pubertal growth, but it should be kept in mind that there are large variations in the timing of puberty—and therefore, in growth rates—among individuals of the same age during the period of adolescence.
Because of these characteristic patterns of growth during childhood and adolescence, the rate of growth (centimeters per year or inches per year) is a key variable in evaluating a short child. Growth rates may vary somewhat with season and can be affected by transient illness, but a child should maintain a relatively set growth channel on the linear growth percentile charts after 2-3 years of age. A persistently slow rate of growth in relation to age-appropriate norms is alarming and is likely to reflect an underlying medical disorder.
Measuring a Child
Stature is evaluated as supine length until 2 years of age and as standing height thereafter ( Fig. 43.3 ). For measurement of supine length, an infant lies on an inflexible ruled horizontal surface, at 1 end of which 1 person holds the infant’s head in contact with a fixed board; a 2nd person extends the infant’s legs as much as possible and brings a movable plate in contact with the infant’s heels. Recumbent measurements average 1 cm (0.4 inch) more than standing height.

After 2 years of age, children are measured standing and barefoot with a device such as a Harpenden stadiometer; a vertical metal bar is affixed to an upright board or wall, and height is measured at the top of the head by a sliding perpendicular plate or block. Measurements of length using pen marks on the examining table at the head and foot of an infant are often grossly inaccurate, as are height measurements using a flexible metal rod atop a standard weight scale.
With the use of optimal techniques, the variation in measurement among observers is less than 0.3 cm (0.1 inch). It is then possible to determine changes in height over 3- to 4-month intervals to estimate the annualized growth rate. However, because of normal seasonal variations in growth rates, a longer interval between measurements (6-12 months) is more reliable in the calculation of height velocity.
Measurements are then plotted on standard growth charts; it is recommended that World Health Organization (WHO) growth charts be used for infants from birth to 2 years of age and Centers for Disease Control and Prevention (CDC) growth charts for children from ages 2-18 years (see Fig. 43.1 ). These CDC and WHO growth charts are available at: http://www.cdc.gov/growthcharts/ and http://www.who.int.easyaccess2.lib.cuhk.edu.hk/childgrowth/standards/en/ .
Calculated growth rates (centimeters per year or inches per year) should be evaluated in relation to age-related norms with growth velocity charts for North American children for children over 2 years of age (see Fig. 43.2 ).
Body Proportions
Apart from linear height, it is also useful to assess the upper-to-lower segment ratio (U/L) ( Fig. 43.4 ) and the arm span. The U/L is determined by measuring the lower segment (vertical distance between the symphysis pubis and the floor, with the child standing) and the upper segment (the difference between the lower segment and height). Arm span is the distance between the outstretched middle fingertips with the child standing against a flat board or wall. The U/L and arm span are used to determine whether the child is normally proportioned or not (in Europe, it is more common to assess sitting height versus standing height for the same purpose).

The U/L gradually declines with age throughout childhood. Since infants have relatively short legs, the U/L is high (an average of 1.7) at birth. It then declines throughout childhood as the legs increase in length relative to the upper body, decreasing to a mean of 0.9 by late puberty (in males; in females, the ratio may be closer to 0.95). The arm span as compared to the height is another measure of body proportions and is normally shorter than the height in younger children and increases to become slightly longer than the height by late puberty (about 5 cm more than height in boys, 1.2 cm more than height in girls). Deviations from the norm in the U/L and the arm span may point to conditions such as skeletal dysplasias, Turner syndrome, or long-standing hypothyroidism (increased U/L segment ratio, i.e., relatively short extremities) and radiation-induced spinal damage or genetic disorders such as Klinefelter or Marfan syndromes and homocystinuria (low U/L segment ratio, due to a relatively short trunk or unusually long extremities).
Weight should also be considered in relation to a child’s stature. Undernutrition is generally caused by nonendocrine factors (poor nutritional intake, malabsorption, systemic illness) and typically leads to a decrease in weight before a decrease in linear growth. Obesity in childhood is usually exogenous; exogenous obesity is generally associated with an accelerated growth rate. In contrast, endocrine disorders that cause poor growth and short stature are often associated with weight gain and obesity (Cushing syndrome, hypothyroidism, and in some instances, GH deficiency). Therefore, the obese child who has a slow growth velocity is more likely to have an endocrine cause of short stature, while the undernourished child with short stature likely has short stature secondary to poor weight gain and is unlikely to have an endocrine disorder.
Familial and Genetic Factors
Both parental height and parental pattern of growth are key determinants of a child’s growth pattern. This strong familial influence on height is not detectable at birth but is manifested by 2-3 years of age. Final adult height is strongly heritable with heritability estimates ranging from 0.8-0.95 (i.e., 80-95% of the observed variation is explained by hereditary rather than environmental factors). The midparental height (MPH) is used as a measure of the child’s genetic growth potential and is an average of the height of both parents after correcting for sex . Since the average adult male is taller than the average adult female by 13 cm (5 inches), 13 cm is added to the mother’s height in the case of a male child, while 13 cm is subtracted from the father’s height in the case of a female child; the parental heights, after correction for sex, are then averaged to obtain the MPH.
Thus MPH is determined in the following manner:
Males: [father’s height in cm + (mother’s height in cm + 13 cm)]/2
Females: [(father’s height in cm − 13 cm) + mother’s height in cm]/2
The MPH is a good index of the child’s genetic height potential, but tends to become less accurate if 1 parent is unusually tall or short; that is, the offspring of an exceptionally tall parent or exceptionally short parent will be closer to the average height than is predicted by the MPH because of the phenomenon of regression to the mean .
In addition to the influence on final adult height, parents’ patterns of growth are also often repeated in their children. In particular, many men who were “late bloomers” with delayed onset of puberty and delayed but normal growth spurts have sons with similar growth patterns.
Ethnic Factors and Secular Trend
The average height of various populations across the globe is not the same; in the current era, Northern European populations are taller than many (but not all) other ethnic groups, and it therefore seems to make sense to use population-specific standards of growth because a child from a generally short ethnic group may be labeled as “short stature” by Northern European standards, but may be normal for his or her own ethnic group. Two additional observations need to be kept in mind:
- 1.
Migrants from countries where the average height is lower tend to have children who are taller than their parents when they move to a country with a higher standard of living. This indicates that at least some of the observed height difference may be environmental (most likely related to nutrition and childhood disease burden) and that this height difference may shrink or disappear in subsequent generations.
- 2.
The Northern European populations were themselves much shorter in the 18th and 19th centuries and average heights have steadily increased as living standards improved (reflecting improvements in nutrition and other public health measures). This “secular trend” in height slows down and plateaus over time, but is much more marked in populations that have recently seen an improvement in living standards.
Thus practitioners should make allowances for ethnic differences in height when evaluating children from different ethnic backgrounds, but should not automatically assume such differences as the sole explanation for short stature in children from historically shorter populations. Growth velocity in particular should not be abnormal even in historically shorter populations, and a subnormal growth velocity should trigger an evaluation in the same way as it would in children from a Northern European background.
General Well-Being
Because growth is a barometer of a child’s health, general well-being and freedom from serious illness are necessary for a child to achieve his or her genetic growth potential. Chronic illnesses that are not primarily problems of stature often interfere with growth secondarily, and short stature may be the presenting feature of such conditions as inflammatory bowel disease, celiac disease, and renal disease.
Psychologic Factors
Under normal circumstances, emotional and psychologic factors do not have a great effect on growth. However, in certain cases, emotional deprivation can lead to very significant growth failure (sometimes labeled “deprivation dwarfism” or “psychosocial dwarfism”) via mechanisms that are still poorly understood.
Endocrine Regulation of Growth
GH is produced by the anterior pituitary under the control of GH-releasing hormone (GHRH) from the hypothalamus, and is essential for normal growth in childhood and adolescence (though it appears to play a relatively small role in prenatal growth). GH is secreted in brief pulses and peak secretion occurs during sleep, so random serum levels have little utility in the evaluation of GH deficiency except in the newborn period (when random GH levels are usually elevated, and a level below 7 ng/mL is very likely to reflect GH deficiency).
GH exerts its growth-promoting effect through stimulating the production of insulin-like growth factor 1 (IGF-1) (primarily in the liver, but also in some target tissues) as well as via direct actions of GH on bone. Most circulating IGF-1 is bound to IGF-binding proteins, with the largest proportion being bound in a ternary complex with IGF-binding protein-3 (IGFBP-3) and a protein named the acid-labile subunit (ALS). Because IGF-1 levels in the blood are stable throughout the day and reflect the integrated effect of GH secretion, measurement of IGF-1 is often used as a surrogate measure of GH secretion.
Thyroid hormone also has a relatively limited role in prenatal growth, but is absolutely essential for normal postnatal linear growth, both via direct actions on the epiphyseal growth plate and via a permissive effect on GH secretion. Hypothyroidism can therefore lead to very profound growth failure and an evaluation of thyroid hormone status is essential in the investigation of growth failure.
Glucocorticoids do not play any significant role in promoting growth, but are powerful inhibitors of growth when present in excess. Persistent exposure to excess corticosteroids (whether endogenous or exogenous) can lead to very severe growth failure (along with significant weight gain). Deficiency of glucocorticoids generally does not adversely affect growth if the child is otherwise healthy.
Sex steroids mediate the pubertal growth spurt (see Chapter 42 ). This involves direct effects of sex steroids on bone growth as well as steroid-induced amplification of GH secretion. Most of the bone-maturing action of sex steroids is mediated by estrogen in both sexes; while testosterone has some direct effects on bone strength and thickness, most of the effects of testosterone on linear growth and the maturation of growth plates in males occur via the action of estrogen produced by the peripheral conversion of testosterone. Consequently, even in males, bone maturation can be affected by genetic defects in the production or action of estrogen, as well as by pharmacologic inhibition of this pathway (e.g., by using aromatase inhibitors that block the conversion of testosterone to estrogen). Sexual precocity (true precocious puberty, exogenous exposure, or congenital adrenal hyperplasia) tends to accelerate linear growth transiently as a result of premature or excessive production of sex steroids (or both) (see Chapter 42 ). But if left untreated, these conditions advance osseous maturation, leading to premature epiphyseal fusion and a short final adult height. The absence of sex steroids (hypogonadism) in the absence of other abnormalities blunts the pubertal growth spurt, but tends not to limit final height, as bone maturation and epiphyseal fusion are also delayed by the lack of estrogen in these patients.
Bone Age
Osseous maturation follows a very predictable pattern during the growth and development of the child, and a radiograph of the nondominant hand can be used to assess bone age (i.e., the degree of maturation of the bones compared to age-matched standards). Bone age is usually estimated by comparing the child’s radiologic findings with a standard set of radiologic images, or by using various scoring algorithms. These readings are subject to observer bias and error but are still useful as long as this inherent subjectivity is kept in mind. Bone age is very closely correlated with pubertal maturation and an assessment of bone age can be especially useful in cases of precocious puberty, delayed puberty, and constitutional growth delay. Except in cases of precocious puberty, bone age is rarely useful in the evaluation of short stature in a child less than 5 years of age.
Causes of Short Stature
Understanding the factors that influence childhood growth leads directly to an understanding of the causes of short stature and to the differential diagnosis of short stature for an individual child ( Tables 43.2, 43.3, 43.4, 43.5, and 43.6 ). While a very large number of conditions can potentially lead to short stature and growth failure, the vast majority of children with short statures are either normal variants (familial short stature, constitutional delay of growth and puberty) or have no discernible cause (idiopathic short stature [ISS]). It is also important to remember that the child with growth failure is far more likely to have an underlying pathology than a child who happens to be short but has a normal growth velocity.
|
|
Mutant Gene | Inheritance | Phenotype |
---|---|---|
HPA | Developmental abnormalities | |
HESX1 | AR | Septo-optic dysplasia; variable involvement of pituitary hormones |
PROP1 | AR | GH, PRL, TSH, LH, FSH deficiencies; variable ACTH deficiency |
POU1F1 (Pit1) | AR, AD | GH, PRL deficiency; variable degree of TSH deficiency |
RIEG | AD | Rieger syndrome |
LHX3 | AR | GH, TSH, LH, FSH, prolactin deficiencies |
LHX4 | AD | GH, TSH, ACTH deficiencies |
SOX3 | XL | GH deficiency, intellectual disability |
GLI2 | AD | Holoprosencephaly, hypopituitarism |
GLI3 | AD | Pallister-Hall syndrome, hypopituitarism |
Isolated Growth Hormone Deficiency | ||
GHRHR | AR | IGHD, type IB form of IGHD |
GHS-R | AD | GHD and ISS |
GH1 | AR | Type IA form of IGHD |
AR | Type IB form of IGHD | |
AD | Type II form of IGHD | |
X-linked | Type III form of IGHD; hypogammaglobulinemia | |
AD | Bioinactive GH molecule | |
Growth Hormone Insensitivity | ||
Growth Hormone Receptor | ||
Extracellular domain | AR, AD | IGF-1 deficiency; decreased or normal GHBP |
Transmembrane | AR | IGF-1 deficiency; normal or increased GHBP |
Intracellular domain | AD | IGF-1 deficiency; normal or increased GHBP |
IGF | ||
IGF1 | AR | IGF-1 deficiency; IUGR and postnatal growth failure |
STAT5B | AR | IGF-1 deficiency, variable immune defect, hyperprolactinemia, chronic pulmonary infections, recurrent eczema |
ALS | AR | IGF-1 deficiency; variable postnatal growth failure, delayed puberty |
Primary GH Insensitivity (Hereditary Defects) |
|
Secondary GH Insensitivity (Acquired Defects) |
|
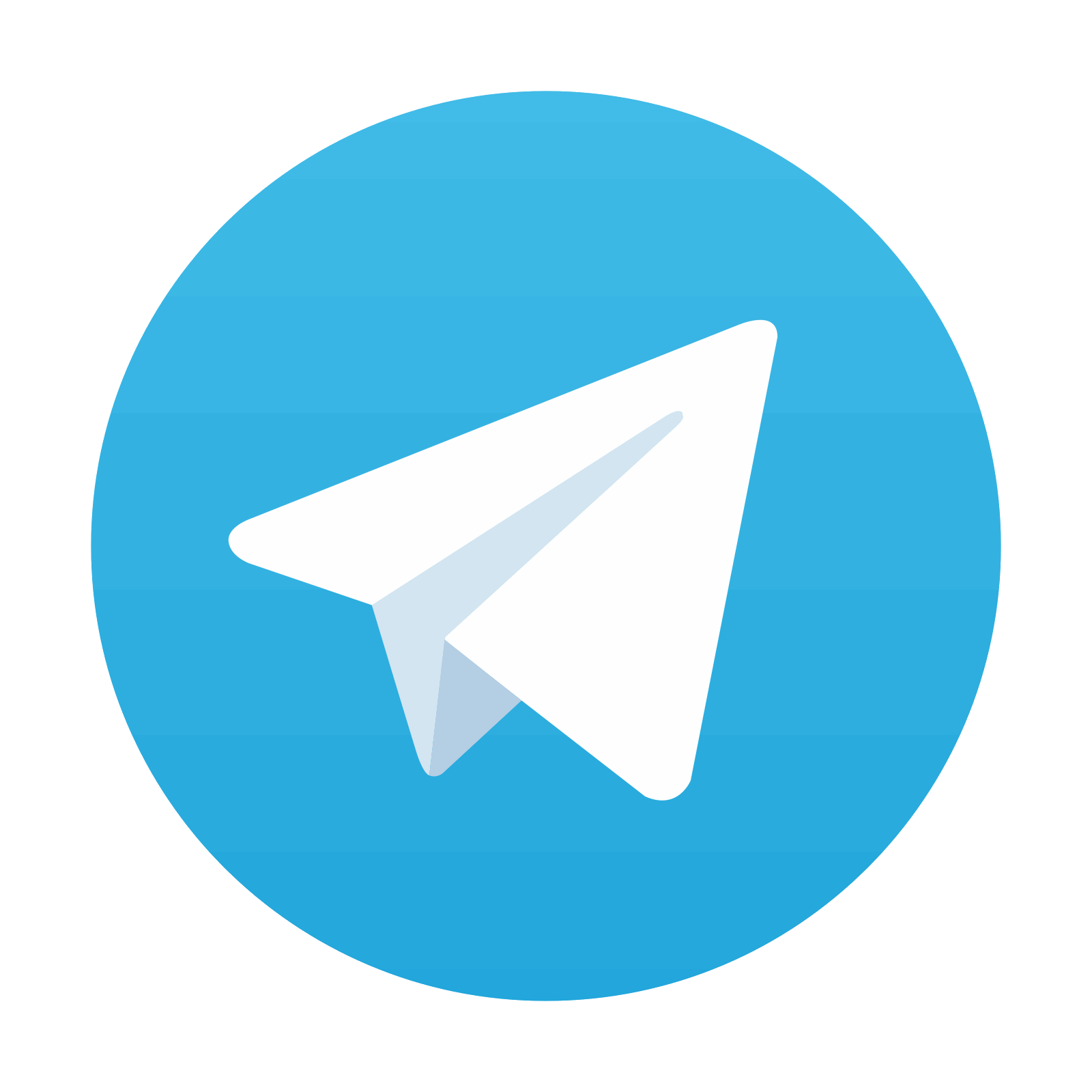
Stay updated, free articles. Join our Telegram channel

Full access? Get Clinical Tree
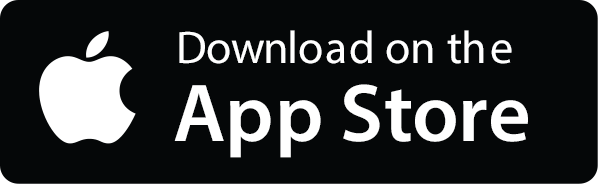
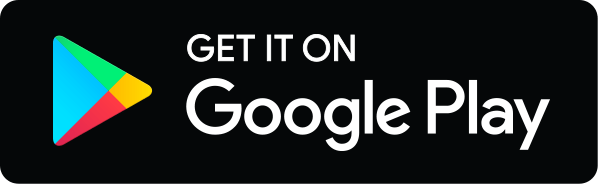
