In the last few years, a new generation of antiepileptic drugs (AEDs) has been developed (1,2), with much interest in drugs that work on the gamma-aminobutyric acid (GABA) system. GABA is the primary inhibitory neurotransmitter in the mammalian nervous system (3,4). Increasing the effects of the GABA system is theorized to prevent seizures. This type of prevention has already been noted with the barbiturates and benzodiazepines, which act on the GABA receptor. Valproate, a GABA analogue, is an effective AED against a variety of seizure disorders, although its precise mechanism of action remains unclear (5,6). Recent attempts to utilize the GABA system have focused on GABA metabolism and have resulted in the development of two new drugs. One drug, vigabatrin (Chapter 67), is an irreversible inhibitor of GABA transaminase (1,5,7). By blocking degradation, vigabatrin raises the levels of GABA. The second drug, tiagabine (Gabitril), was approved by the U.S. Food and Drug Administration (FDA) for the adjunctive treatment of partial seizures in adults and adolescents in 1997.
Tiagabine was developed as a designer drug to block GABA uptake by presynaptic neurons and glial cells (1,3,8–13). This blocking should increase GABA concentration in the synapse, resulting in longer duration of action in the synaptic cleft without substantially altering total brain GABA levels. Tiagabine has undergone extensive clinical trials in adults with intractable partial seizure disorders (14–19). Less information is available on the use of tiagabine in children, although some clinical trials were done (20–23). In this text, we review the available data on tiagabine with an emphasis on its use in the pediatric age group. Because tiagabine use in epilepsy has not been substantial, the pediatric data are relatively sparse. This chapter reviews the available data, including the recent safety data, with an emphasis on the pediatric aspects.
PHYSICOCHEMICAL CHARACTERISTICS
Tiagabine hydrochloride is a whitish, odorless crystalline powder with the chemical name (-)-(R)-1-[4,4-Bis (3-methyl-2-thienyl)-3-butenyl] nipecotic acid hydrochloride. The chemical formula is C20H25NO2S2• HCl, and the structure is shown in Figure 64.1. It is 3% soluble in water and is insoluble in hexane. The nipecotic acid moiety of the molecule has an asymmetric carbon, and the R (−) enantiomer is four times more potent that the S (+) enantiomer. The name tiagabine refers to the R (−) enantiomer, which consists of nipecotic acid joined to a lipophilic anchor.
Animal experiments demonstrated that nipecotic acid was anticonvulsant and could preferentially block glial and neural GABA uptake in mice (24). However, nipecotic acid had to be injected intracerebrally in animals because it did not cross the blood–brain barrier. The addition of a lipophilic anchor to nipecotic acid allowed passage across the brain barrier and maintained the desired property of blocking GABA uptake. The resulting compound is tiagabine hydrochloride and is referred to as tiagabine.
MECHANISM OF ACTION
Tiagabine increases the amount of GABA available in the extracellular space by preventing GABA uptake into presynaptic neurons, as schematically shown in Figure 64.2. The increased GABA enhances inhibitory effects on receptors of postsynaptic cells. This effect was confirmed in hippocampal rat slices, in which tiagabine prolonged GABA-mediated inhibitory postsynaptic potentials (25).
In vitro work in adult rats has shown that tiagabine blocks GABA uptake by binding in a stereospecific and saturable fashion to the GABA recognition sites in neurons and glial cells (26). Tiagabine binds to a class of high-affinity binding sites that most likely represent the GABA transporter GAT-1 (27).
The action of tiagabine is confined to blocking GABA uptake. Tiagabine is not itself taken into neurons or glia. It is highly selective for the GABA system and does not affect other neurotransmitters. Tiagabine binds weakly to the benzodiazepine and H1 receptors, but only at concentrations 20 to 400 times those required to inhibit GABA uptake (26). This binding is unlikely to be clinically relevant.
FIGURE 64.1 Chemical structure of tiagabine HCl.
Tiagabine is an anticonvulsant in a variety of animal models of epilepsy, including pentylenetetrazol (PTZ)-induced seizures (11), DMCM-induced seizures (8), and, at high doses, seizures induced by maximal electroshock (11) (DMCM, methyl 6,7-dimethoxy-4-ethyl-beta-carboline-3-carboxylate, is a proconvulsant that is an inverse agonist of the benzodiazepine receptor).
Tiagabine has shown other anticonvulsant activity in animal models. Tiagabine suppresses amygdala-kindled seizures in rats (8) and is effective in treating convulsive status epilepticus in cobalt-lesioned rats (28). Tiagabine also has moderate efficacy in the genetically determined generalized epilepsy in photosensitive baboons. Tiagabine may have a proconvulsant effect that has been noted with other AEDs. Spike-and-wave discharges were noted after control of motor status at doses of more than 5 mg/kg in both lesioned and normal rats (28). In a strain of rats (WAG/Rij) that are genetically prone to epilepsy—a strain that is considered an animal model of generalized nonconvulsive absence epilepsy—type II spike–wave discharges were increased at doses of 3 mg/kg and 10 mg/kg, but not at doses of 1 mg/kg, which is closer to the therapeutic range (29). In these epilepsy-prone rats, the increase in spike–wave discharges was not correlated with behavioral abnormalities in the animals. These data suggest that tiagabine may exacerbate absence seizures. However, tiagabine is effective in blocking PTZ-induced seizures, which often predicts efficacy against generalized seizures.
FIGURE 64.2 Tiagabine inhibits the uptake of GABA in the synaptic cleft.
The adult animal data suggest that tiagabine may have efficacy in treating some types of both partial and generalized seizure disorders. Data are lacking on the effects of tiagabine on experimental seizure models in the developing brain of animals. This lack of data is unfortunate, because some AEDs have age-dependent effects on seizures (30–32), but it is a common problem with all AEDs in early clinical usage.
METABOLISM AND PHARMACOKINETICS
Animal studies have shown that tiagabine is rapidly absorbed and has a short half-life: 1 to 3 hours in rats and 1 to 2 hours in dogs. Bioavailability is 25% in dogs and 50% to 75% in rats (9). Tiagabine, like many other AEDs, is metabolized by the hepatic cytochrome P450 system (33). Metabolites include 5-oxotiagabine, which is excreted in the urine. Tiagabine is also conjugated with glucuronic acid, and metabolites are excreted via urine and via feces through the biliary system (9).
Studies in adult volunteers have shown that tiagabine is rapidly absorbed from the gastrointestinal tract, and peak plasma concentrations (Cmax) are achieved within 2 hours. Absorption is linear and independent of the dose (34). The rate—but not the extent of absorption—is reduced by food. The time to peak plasma concentration (Tmax) after a meal is more than twice that in the fasting state. However, although the Cmax is lower, the area under the curve (AUC) is similar (35). Clinical trials were therefore performed with patients taking tiagabine after a meal in order to reduce the possible peak-related toxicities without altering the total amount of drug absorbed.
In normal volunteers, the mean half-life of tiagabine is 4 to 9 hours and is independent of the dose. Because tiagabine is metabolized by the hepatic cytochrome P450 system, it has a shorter half-life in patients taking drugs that induce that system, such as barbiturates, carbamazepine, and phenytoin (36). When given in single or multiple doses, tiagabine does not appear to induce or inhibit hepatic microsomal enzyme systems (34,37).
Tiagabine is extensively metabolized by the hepatic microsomal system because less than 2% is excreted unchanged in the urine (34,38,39). Radiolabeled [14C]tiagabine studies in human volunteers showed that 25% was excreted in the urine and 63% in the feces (40). Tiagabine has no identified active metabolites (40). Tiagabine does not appear to accumulate appreciably in plasma with multiple dosing. In Phase I studies of healthy volunteers given daily doses over a 5-day period, both the peak levels and the AUC increased proportionally with the dose, as would be expected in a drug with linear pharmacokinetics (34). The therapeutic half-life, as distinct from the serum half-life, is unclear. Microdialysis studies in patients given oral tiagabine demonstrated extracellular GABA levels in the brain that rise within about 1 hour after oral dosing (41) but are not sustained more than a few hours and do not completely correlate with the serum concentrations (40). The duration of the antiepileptic activity, which is presumably mediated by these changes in GABA, remains unclear and has not been precisely studied.
Tiagabine is heavily protein bound, although it does not appear either to displace or to be displaced by most of the commonly used drugs (42,43). In vitro studies showed that tiagabine is more than 95% protein bound, primarily to albumin and a glycoprotein.
Tiagabine pharmacokinetics were studied in patients with epilepsy who were taking tiagabine as add-on therapy. Steady-state tiagabine doses ranged from 24 to 80 mg/day in four divided doses (44). All patients were taking enzyme-inducing concomitant AEDs. Results showed that tiagabine had linear kinetics in this population also, with a linear dose–response curve for peak concentrations (Cmax) as well as for the AUC. Although the kinetics remained linear, the half-life of tiagabine was shorter in patients taking AEDs that induce the cytochrome P450 system than for those on monotherapy or taking noninducing AEDs (36). This difference is expected and explains why the dosages used in this study were considerably higher than those tolerated by healthy volunteers who were not taking enzyme-inducing AEDs. The dosage ranges in this study exceeded the upper doses of 56 mg/day used in the randomized clinical trials in the descriptions that follow.
The most important drug interaction of tiagabine to date is the effect of enzyme-inducing AEDs. The half-life of tiagabine was shorter and the AUC smaller for the same dose in patients receiving enzyme-inducing AEDs (carbamazepine, phenytoin, phenobarbital, primidone) than in those on tiagabine monotherapy (9,36,45). The pharmacokinetics of tiagabine in patients taking an AED that was not enzyme inducing, such as valproate, were similar to those in healthy volunteers. Dosages of tiagabine must to be substantially higher in patients taking concurrent enzyme-inducing AEDs than with monotherapy or noninducing AEDs. Similar guidelines have been suggested for the recently approved AED lamotrigine (46,47). Although the half-life of tiagabine is shortened by the concurrent use of enzyme-inducing AEDs, it is not prolonged by the concurrent use of valproate, as is the case with lamotrigine and phenobarbital (46–49).
Tiagabine has little effect on other AEDs. Tiagabine does not appear to affect the kinetics of carbamazepine or phenytoin (42). However, tiagabine did reduce the peak concentration and the AUC of valproate by approximately 10% (49). The reason for this reduction is expected to be of little or no clinical importance. Because tiagabine is metabolized by the 3A isoform subfamily of the hepatic cytochrome P450 system (33), there is potential for interaction with other drugs with similar metabolism, including other AEDs, cimetidine, theophylline, warfarin, and digoxin. However, no clinically significant interactions have been found between tiagabine and these drugs (43). Surprisingly, a recent study showed no interaction with erythromycin (50).
Oral contraceptives are also metabolized by the cytochrome P450 system. In one study, tiagabine, given at a dose of 8 mg/day as monotherapy to healthy young women, did not affect the metabolism of oral contraceptives (37,43). Higher doses of tiagabine would not be expected to have an interaction because tiagabine is neither an inducer nor an inhibitor of the cytochrome P450 system, but data are lacking to confirm this impression.
Tiagabine pharmacokinetics behave predictably in hepatic and renal disease (51,52). There is no effect from renal impairment, and there is reduced clearance and a longer half-life with hepatic impairment. Patients with hepatic disease require lower doses to attain concentrations similar to those in healthy subjects.
Single-dose tiagabine pharmacokinetics have been studied in children (20). In this study, 25 children ages 3 to 10 with complex partial seizures who were taking one AED were given a single dose of 0.1 mg/kg of tiagabine, and plasma concentrations were checked over the next 24 hours. The mean half-life of tiagabine was 3.2 hours in the 17 children on enzyme-inducing concomitant AEDs (carbamazepine or phenytoin) and 5.7 hours for the 8 children on valproate (noninducing AED). This result is comparable to the adult data. Clearance rates, peak plasma concentrations, and AUC of tiagabine were affected by the concurrent AEDs in a manner similar to adults. The clearance of tiagabine was two times higher in children than in noninduced adults with epilepsy when the rate was adjusted for body weight. However, when adjusted according to body surface area, clearance rates of tiagabine in children were 1.5 times higher than in noninduced adults with epilepsy. The results indicate that tiagabine pharmacokinetics in children ages 2 and older are similar to those in adults, including the effects of concurrent AEDs. Infants have not been studied.
The initial studies of tiagabine were done in patients who were also on other AEDs, primarily enzyme-inducing ones such as carbamazepine. Subsequently, tiagabine has been used in conjunction with AEDs that are not enzyme inducing, as well as in monotherapy. The effect of enzyme-inducing AEDs is clearly much stronger than had been appreciated. It appears that the dose of tiagabine for someone who is not on enzyme-inducing AEDs is less than half that for someone who is. Failure to appreciate this difference when tiagabine is used in conjunction with the newer AEDs that are not enzyme inducers can result in significant toxicity.
DATA ON HUMAN ADULT EFFICACY
Add-On Therapy in Adults With Partial Seizures
The clinical data regarding the efficacy of tiagabine as add-on therapy come from five placebo-controlled clinical trials in patients with intractable partial seizures. In all five trials, tiagabine was statistically significantly more effective than placebo in the treatment of one or more partial seizure types (53). Two of the studies were crossover and three were parallel group. Most of the patients were adults ages 18 to 75; however, adolescents starting at age 12 were also included. Thus, efficacy data are available in this age group, although in a relatively small number of patients. Pediatric trials in younger children are discussed separately.
The first Phase II multicenter study was a crossover design performed in Europe with 94 patients ages 18 to 65 with refractory complex partial seizures (14). The study had an “enrichment” design. Patients started with a dose of 8 mg/day of tiagabine; then the dose was titrated to either reduce seizures sufficiently or produce unacceptable adverse events, with a maximum dose of 52 mg/day. Patients who improved in this phase were then enrolled in a double-blind trial. Of the 94 patients enrolled, 20 discontinued participation during the open-label phase, and another 28 failed to qualify for the double-blind phase for lack of sufficient reduction in seizures. The average total daily dose of tiagabine in the 42 patients in the double-blind phase was 33 mg/day, and most were taking a concomitant enzyme-inducing AED. Even with this relatively small sample size, the frequency of complex partial seizures only and partial seizures with secondary generalization was significantly reduced when the tiagabine and placebo phases were compared. The second study was similar in design and allowed dosing up to 64 mg/day. The intent-to-treat group was 36 patients taking a mean dose of 46 mg/day. Tiagabine was significantly superior to placebo in reduction of all partial, complex partial, and partial with secondary generalization seizures (54).
The three randomized Phase III multicenter, placebo-controlled, double-blind, parallel-group studies in adults included two studies in the United States and one in Europe. All of these studies showed that tiagabine was significantly superior to placebo as adjunctive treatment of partial seizures.
The first U.S. study (15) was a dose–response study, with patients receiving placebo, or 16, 32, or 56 mg/day of tiagabine in addition to their regular stable AED regimen. Tiagabine was significantly better than placebo at both 32 and 56 mg for median change from baseline and proportion of patients achieving at least 50% reduction in complex partial seizures (in both, P < .03). Tiagabine was effective for both median change from baseline and proportion, with more than 50% reduction in the groups receiving 16, 32, and 56 mg for simple partial seizures.
Partial seizures with secondary generalization were reduced compared to placebo, but this reduction did not reach significance, possibly because of fewer patients and numbers of seizures. A clear dose response was present in this study. The minimum effective dose of tiagabine necessary to achieve a reduction of 50% in patients with complex partial seizure frequency was 32 mg/day, with the group at 56 mg/day showing a better response. Doses greater than 56 mg were not studied in this trial. Note that the patients in this study and in the other two randomized studies were all taking one to three concurrent AEDs, at least one of which was required to be an inducer of the cytochrome P450 enzyme system. Thus, as discussed in the metabolism section, the doses required in monotherapy, or with a single concomitant noninducer such as valproate, are substantially smaller than when used together with an enzyme-inducing AED such as carbamazepine. The patients in this study were, by and large, a very refractory group with a median duration of epilepsy of 20 years, who had failed to respond to a median of seven AEDs prior to study entry.
The second U.S. multicenter, randomized, double-blind, placebo-controlled, parallel-group trial compared placebo to 32 mg/day of tiagabine as add-on therapy in 318 patients (16). In this dose-frequency trial, the tiagabine patients received a total of 32 mg/day as 16 mg twice a day or as 8 mg four times a day. Both groups were superior to placebo (P < .001) in the number of patients achieving a reduction greater than 50% in complex partial seizures. Both groups were also superior to placebo for simple partial seizures, for either reduction from baseline or proportion, with a reduction in seizures of at least 50%. Taken together with the prior study, the data suggested that the add-on dose of 32 mg/day was likely to be in the low therapeutic range for enzyme-induced patients.
The multicenter European double-blind, parallel-group study was the only adjunctive trial to evaluate tiagabine given three times daily (tid) (55). Tiagabine was given as 10 mg tid or 8 mg tid if a dose of 30 mg/day was not tolerated. There were 154 patients randomized to tiagabine (n = 77) or placebo (n = 77). The tiagabine-treated group had a significant reduction in the number of both complex and simple partial seizures.
Tiagabine consistently demonstrated efficacy against partial seizures in these trials, but the dose ranges chosen were probably too low in enzyme-induced patients to establish its upper therapeutic range and maximum efficacy. These trials included 67 adolescents ages 12 to 18 and therefore can be regarded as demonstrating efficacy and safety for this age group as well.
These placebo-controlled trials showed that tiagabine is superior to placebo. The challenge for the practicing clinician is to determine the role of a new AED in the treatment of patients for whom there are a number of other AEDs to consider. Tiagabine has been compared to add-on carbamazepine or phenytoin in a double-blind multicenter study. Placebo and active drug were given in a double-dummy design of patients on baseline carbamazepine receiving add-on tiagabine or phenytoin, or patients on baseline phenytoin receiving add-on tiagabine or carbamazepine. Patients were allowed to titrate to their best-tolerated dose within a wide range to mimic clinical practice.
Preliminary results of the patients taking baseline carbamazepine were reported (56). There was no difference in efficacy between the tiagabine group (n = 106) and the phenytoin group (n = 100) for any partial seizure type in the intent-to-treat analysis. However, phenytoin patients had greater discontinuations for adverse events (17%) than those on tiagabine (10%). Overall discontinuation rates also favored tiagabine (31% vs. 22%). However, further studies are needed before the precise role of tiagabine in treating refractory partial seizures can be determined.
Monotherapy Trials
There have been three studies of tiagabine monotherapy in adults with partial seizures (57,58). The first was a double-blind randomized pilot study of 11 patients who had their AEDs discontinued while they were in the hospital undergoing monitoring for presurgical evaluation (58). Patients receiving tiagabine had fewer seizures than those receiving placebo during the treatment period. In the second study (57), which was an open-label, dose-ranging study, 19 of 31 patients (61%) successfully converted to tiagabine monotherapy. In the third study, which included 198 randomized patients with partial seizures, patients were randomized to daily doses of tiagabine as either 6 or 36 mg/day. Baseline AEDs were discontinued after tiagabine was added on. Seizure frequency was reduced in both the low- and high-dose groups, with the high-dose group having a significantly higher proportion of patients who experienced a reduction in seizure frequency that was greater than 50% (57). The median change in seizure reductions, however, did not reach significance between the groups. The following open-label pediatric data have also been promising (22).
Human Pediatric Efficacy Data
In an open-label pilot study (22), 25 children with refractory complex partial seizures from a single-dose pharmacokinetics study (20) were enrolled in an open-label, long-term study. Children were initially taking a dose of 0.1 mg/kg/day of tiagabine. They were also continuing a baseline concomitant AED. The dosage was increased every 2 weeks by 0.1 mg/kg/day until clinical efficacy or toxicity was reached. The results of this study have been encouraging for the use of tiagabine in children. As of January 1996, 23 of 25 children were still taking tiagabine. Of 21 patients treated for longer than 6 months, 19 (90%) had a reduction in the frequency of complex partial seizures that was greater than 50% compared with historical seizure rates. These children had previously uncontrolled partial seizures. For these children, the median 4-week historical baseline rate for complex partial seizures was 5 (range 1–90) and mean 21.1. Conversion to tiagabine monotherapy was possible for 16 of the children, and they remained seizure-free at least 2 months on a mean dose of 0.31 mg/kg/day.
The other pediatric study was a dose-ranging, multicenter, add-on study, conducted at three European sites, of 52 children ages 2 to 15 with epilepsy with a variety of seizure types and epilepsy syndromes refractory to other AEDs (21). The results are unfortunately difficult to interpret because of the heterogeneous nature of the epilepsy disorders in the study. Twenty-two of the children had generalized epilepsies, including Lennox–Gastaut syndrome; others had myoclonic seizure disorders, infantile spasms, or childhood absence. Before entry into the trial, the children had been unsuccessfully tried on 3 to 16 other AEDs, including carbamazepine, valproate, and vigabatrin for more than 80% of them.
This study differed from the U.S. open-label study. After a placebo lead-in period of three weeks, tiagabine was given at more rapid escalating doses than in the U.S. study of 0.25 mg/kg/day up to 1.5 mg/kg/day. Dose escalation in the U.S. study was by 0.1 mg/kg/day at no faster than weekly intervals. Patients who completed this phase either entered a long-term extension study or were withdrawn from tiagabine. Of the 53 patients enrolled, 47 entered the tiagabine-dosing phase. Of these, 20 completed the trial and 20 withdrew for lack of efficacy (n = 16), adverse events (n = 3), or other reasons (n = 1). Seven patients were still in the dosing phase when the preliminary report was published (21). Seventeen of 20 patients completing the study entered the long-term extension.
The drug was generally well tolerated (see the following discussion of safety). The study showed no improvement for children with generalized epilepsy, but those with partial seizures improved at doses ranging from 0.37 to 1.25 mg/kg/day. However, the results of this small-scale trial in a very refractory population did not demonstrate a statistically significant effect. These results suggest even more strongly that a controlled trial is needed to determine the place of tiagabine in treating children with partial seizures. The results from the open-label U.S. experience are encouraging, but they should have further confirmation.
SAFETY
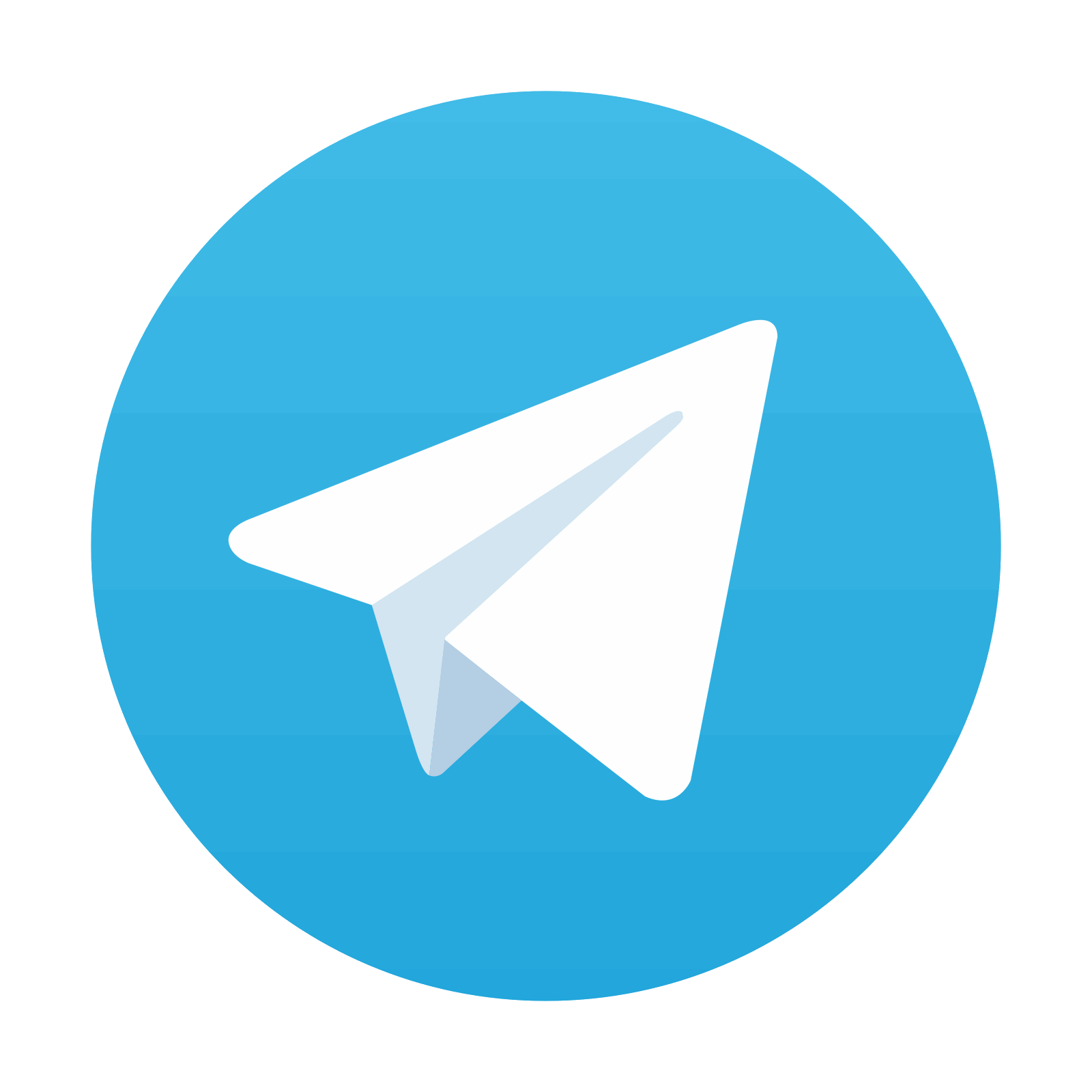
Stay updated, free articles. Join our Telegram channel

Full access? Get Clinical Tree
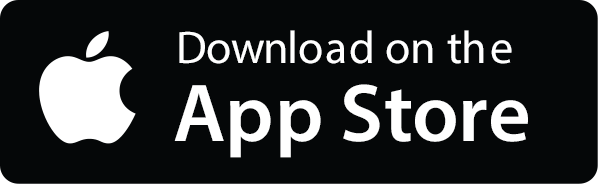
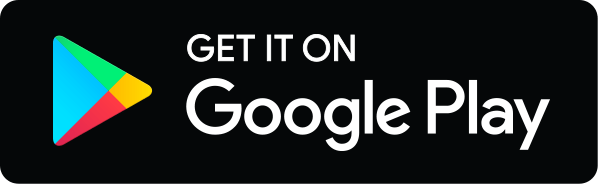