Objective
Recently the use of free fetal deoxyribonucleic acid (DNA) in maternal plasma and serum has been applicable for noninvasive prenatal genetic diagnosis. In this study, we applied a new algorithmic base conventional polymerase chain reaction (PCR) genotyping method and also real-time PCR for detecting fetal X and Y-chromosome sequences in maternal plasma to determine fetal sex in pregnant women in their early gestational ages (5-13 weeks). Finally, we compared the efficiency of each method in sex determination.
Study Design
DNA was extracted from 106 pregnant women and their husbands’ blood samples. Fetus mini–short tandem repeat (STR) genotyping was accomplished through amplification of 19 mini-STRs and 3 non-STR markers using conventional PCR followed by polyacrylamide gel electrophoresis analysis. Simultaneously, TaqMan real-time PCR was done with the use of DYS14-specific primers and probe.
Results
In conventional PCR method, 47 cases were diagnosed to be male and 49 to be female. In comparison, real-time PCR amplified DYS14 (Y-marker) sequences in 45 pregnant women plasma samples. Sensitivity and specificity were calculated to be 95.9% and 98% for conventional PCR and 91.8% and 100% for real-time PCR method, respectively.
Conclusion
According to our study, the conventional PCR method was more sensitive than real-time PCR and it could be employed in future clinical diagnostics singly or in combination with real-time PCR.
One of the long-sought goals in human genetics is noninvasive prenatal genetic diagnosis that during last decade detection of free fetal deoxyribonucleic acid (DNA) in maternal plasma and serum through molecular methods has made it appear possible. Fetal DNA is detectable from the fifth week of gestational ages and will be cleared from circulation of pregnant women very rapidly after delivery.
The concentration of free fetal DNA in maternal plasma is much higher than that present in the cellular fraction, and also it was shown that free fetal DNA is, on average, 3.4% of the total DNA in the maternal plasma and 0.13% in serum.
Knowing fetal gender at early gestational ages is useful for those pregnant women carrying a fetus with an X-linked disease or congenital adrenal hyperplasia (CAH). For example, in CAH cases, early sex determination gives us the ability to manage dexamethasone treatment.
Because invasive procedures like chorionic villus sampling and amniocentesis carry a risk of miscarriage of approximately 1% and they cannot be performed at early gestational ages, and also ultrasonography, which is a noninvasive method, cannot be done reliably during the first trimester (the fallus is not developed completely), the use of free fetal DNA in maternal plasma as a noninvasive method has been noticed for the above-mentioned applications more than other techniques.
Lo et al in 1997 determined fetal sex using free fetal DNA in maternal blood for the first time in which Y-sequences (DYS14) were targeted by conventional polymerase chain reaction (PCR). Moreover, many studies have shown the possibility of using DYS14, sex-determining region Y (SRY), and Y-specific repeat sequences by conventional PCR for sex determination. Since then, more sensitive techniques such as fluorescence-based PCR and real-time quantitative PCR procedures have been developed.
Recently real-time PCR has been run for detection of DYS14 and SRY sequences in maternal blood in different studies.
Based on different protocols and methods that have already been used, specificity and sensitivity of such studies ranges from 31% to 100%, so this prompted us to design a modified algorithm-based conventional PCR method with high accuracy and compare it with a high efficient and sensitive real-time PCR method (TaqMan).
Material and Methods
Subjects
Peripheral blood samples were obtained from 106 pregnant women at their 5-13 week gestational ages referring to obstetricians in Hafez Hospital (Shiraz, Iran) for their first visit. Women with their second (and more) pregnancies (nonsingleton pregnancies) and those who had abortion, transplantation, and blood transfusion were excluded. Their husbands’ blood samples also were taken to be used for the conventional PCR genotyping.
Informed written consent was obtained from each pregnant woman and her husband participating in this study before blood sampling. For each case, 6 mL of peripheral blood was collected into a vacutainer tube containing EDTA and rapidly was transferred to our laboratory. Plasma was isolated from the pregnant women’s whole blood samples as soon as possible by centrifugation in 2 consecutive steps (18,000 × g for 10 minutes [optional: storing at –80°C] and 2700 × g for 45 minutes). The buffy coat of pregnant women (after plasma removal) and also their husband’s whole blood samples were prepared for DNA extraction or stored at –20°C for subsequent use.
DNA preparation
DNA was extracted from 1 mL plasma samples using QIAamp DNA blood minikit (QIAGEN, Hilden, Germany) according to the blood and body fluid protocol with minor modifications. The final volume of the eluted DNA was about 50 μL. The pregnant women’s buffy coat and their husband’s whole blood samples were applied for DNA extraction using a modified salting-out method. The DNA quantity and quality were measured by NanoDrop (ND1000; NanoDrop Technologies, Wilmington, DE) and proved to be implemented efficiently as template for PCR analysis. By strict precautions and aerosol-resistant pipette tips, exogenous DNA contamination was prevented throughout the process. In addition, all procedures including blood specimen collection and preparation, DNA extraction, and PCR amplification were performed almost exclusively by a female staff member in separated partition.
Modified conventional PCR method
For conventional PCR, we made use of 10 X-chromosome mini-STRs and 12 Y-chromosome mini-STRs and non-STR markers to trace fetal DNA in maternal plasma. X and Y Mini-STRs and non-STR markers (maximal expected size range of each PCR product is less than 313 bp because this is the size of more than 99% of fetal-derived DNA molecules) were selected among different forensic and related studies in which these mini-STRs were highly cited by various articles in the field and applicable for detecting fragmented DNA samples. Thus, they were used in our experiment after assessing their heterozygosity in our population, if possible, and size and distributiveness on related chromosomes.
Incidentally, using specific primers for each mini-STR ( Appendix ; Supplementary Figure and Tables 2 and 3 ), PCR was performed with 2 different thermal conditions. The PCR for amplifying X mini-STRs was carried out by the following: initial heating at 95°C for 5 minutes, 30 cycles of 95°C for 1 minute, 60°C for 30 seconds, and 72°C for 20 seconds and a final extension at 60°C for 40 minutes. For amplifying the Y mini-STRs and non-STR markers, the conditions were 95°C for 5 minutes for an initial denaturation, 30 cycles of 95°C for 1 minute, 59°C for 30 seconds, and 72°C for 20 seconds and a final extension at 60°C for 40 minutes. Each reaction (25 μL) for amplifying either X mini-STRs or Y markers consisted of the following: 2.5 μL of 10× PCR buffer, 1.5 mM of MgCl 2 , 0.3 mM of deoxynucleotide triphosphates, 2 U of SmartTaq polymerase, 0.3 mM of each primer, and 4μL of plasma DNA (about 24 ng) or 2.5 μL of each parent’s DNA samples. Afterward, we used polyacrylamide gel electrophoresis (PAGE) for the size separating of PCR products.
PAGE analysis
After preparation of 10% polyacrylamide gel, 7 μL PCR product of each mini-STR was loaded in a vertical electrophoresis apparatus (PROTEAN II Xi cell; Bio-Rad Laboratories, Hercules, CA), and the gel was run at a constant current of 130 mA. Then the gel was stained with the silver staining method. Finally, the genotypes of each fetus were determined by the comparison with his or her parents’ pattern of mini-STR alleles.
Real-time PCR method
Real-time PCR analysis was performed with the ABI PRISM 7500 sequence detection system (Applied Biosystems, Foster City, CA) using TaqMan chemistry. This technique allows for the amplification of a specific sequence of DNA. If the target sequence is present, exponential DNA amplification causes a proportional increase in reporter dye fluorescence. The number of amplification cycles required to reach a fixed threshold signal intensity is termed the cycle threshold. In this method the whole reaction consisted of the following: 10 μL Premix EX Taq (2 times) (TaKaRa Co, Shiga, Japan), 5 μL plasma DNA (about 30 ng), 0.8μL forward primer (10 nM), 0.8 μL reverse primer (10 nM), 0.4 μL probe (10 nM), and 0.4 μL ROX reference dye (50 times) (TaKaRa Co). Real-time PCR thermal conditions were as follows: 50°C for 2 minutes for the AmpErase activation, 95°C for 10 minutes as initial denaturation, and 45 cycles of 95°C for 10 seconds and 60°C for 1 minute.
The DYS14 sequence was targeted using a pair of specific primers and a very specific probe obtained from previous highly cited articles ( Appendix ; Supplementary Figure and Table 4 ). It should be noted that, we utilized triplicates and also excess volume of plasma DNA in each reaction tube to make the method work reliably and efficiently for detecting fetal DNA.
Statistical analysis
SPSS 16.0 (SPSS Inc, Chicago, IL) software was utilized for data analysis. Sensitivity and specificity of each test were calculated with the confidence interval of 95%.
Results
Pregnancy outcome and fetal sex, as determined by ultrasonography in late gestational ages or after birth, revealed that among 106 pregnant women, 49 of all (46.2%) were carrying male fetuses, 6 of them (5.6%) were reported with a miscarriage (excluded from statistical analysis), and the rest of them (48.1%) were carrying female fetuses.
Modified conventional PCR method
Conventional PCR followed by PAGE detected Y-chromosome mini-STR and non-STR sequences (and/or simultaneous absence of paternal X-miniSTR alleles) in 47 pregnant women in which their fetuses were assumed to be male. Paternal X-miniSTR alleles were found in 49 of the Y-chromosome sequence-negative samples in which their fetuses were addressed to be female.
Among all samples, 95 cases were correctly predicted to have the child with the same sex as what it was determined to be. Among 5 cases that were not correctly predicted, the sex of 1 case was incorrectly predicted and the fetal DNA in 4 cases failed to be detected by a conventional PCR method ( Figure 1 ). So the sensitivity and specificity of modified conventional PCR regarding the pregnancy outcome were calculated to be 95.9% and 98%, respectively ( Table 1 and Figure 2 ).

Pregnancy | Pregnant women with male fetus | Pregnant women with female fetus | Sensitivity | Specificity | Failed samples | Gestational age for failed samples, wks |
---|---|---|---|---|---|---|
Conventional PCR | 47 | 49 | 95.90% | 98% | 1, 2, 24, 41, 57 | 5, 6, 5, 9, 7 |
Birth outcome | 49 | 51 | ||||
Real-time PCR | 45 | 51 | 91.80% | 100% | 1, 2, 19, 43 | 5, 6, 6, 5 |
Birth outcome | 49 | 51 |
Classification | Mini-STR | Primer sequences (5′-3′) | Motif repeat | Product size (bp) |
---|---|---|---|---|
Y mini-STRs | DYS389b |
| (TCTG)5 (TCTA)12 | 122 |
DYS459 |
| (ATTT)n | 136-156 | |
DYS446 |
| (TCTCT)n | 85-160 | |
DYS426 |
| (GTT)n | 85-110 | |
DYS438 |
| (TTTTC)n | 95-140 | |
DYS481 |
| (CTT)n | 115-158 | |
DYS505 |
| N-7-(T)-[TCCT]n−2-N24 | 97-125 | |
DYS441 |
| N7-[TTCC]n-2-(T)-N-7 | 91-119 | |
DYS392 |
| (TAT)n | 94-130 | |
Non-STR markers | SRY |
| Nonrepeat sequences | 76 |
DYS14 |
| Nonrepeat sequences | 84 | |
X and Y markers | Amelogenin |
| Nonrepeat sequences | 106,112 |
X mini-STRs | DXS7133 |
| TAGA | 76–100 |
DXS8378 |
| CTAT | 95–11 | |
DXS7132 |
| (TCTA)x-(TCA)(0-l)-(TCTA)2 | 135–163 | |
DXS7423 |
| CCAT | 95–115 | |
GATA31E08 |
| (AGGG)x-(AGAT)y | 95–131 | |
DXS6789 |
| (TATC)(0-l)-(TATG)x-(TATC)y | 124–168 | |
GATAD05 |
| TAGA | 122–150 | |
DXS6803 |
| (TCTA)x-(TCA)(0-l)-TCTA | 102–130 | |
DXS101 |
| (CTT)x-(ATT)y | 126–177 | |
GATA165B12 |
| AGAT | 90–110 |
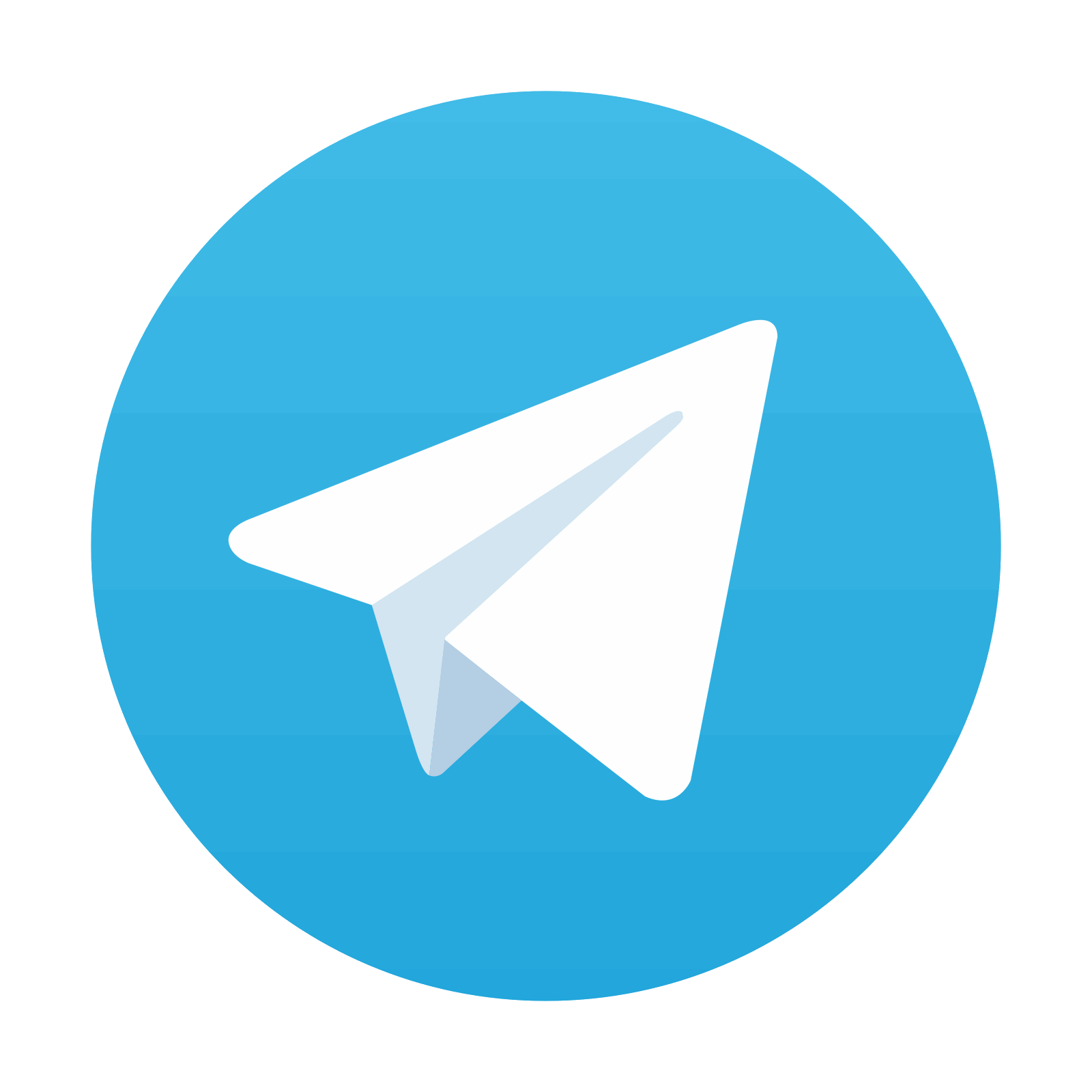
Stay updated, free articles. Join our Telegram channel

Full access? Get Clinical Tree
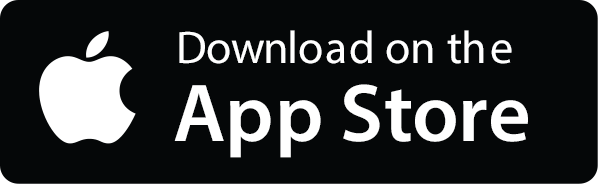
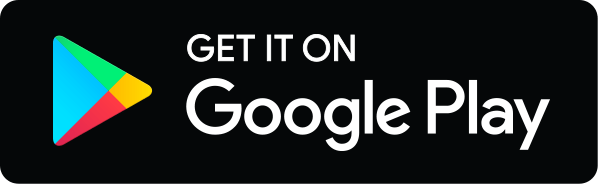
