Situation
Examples
Predominant pathophysiological disturbance(s)
Mode
PEEP*
V T range (mL/kg)
Considerations regarding ventilator mode and settings
Apnoea
Preterm infant with apnoea of prematurity
Ex-preterm infant with respiratory syncytial virus
Poor respiratory drive, physiological consequences of episodic apnoea (bradycardia and desaturation)
SIMV
Low–medium
4–5
Should only need low mandatory ventilator rate. Lungs often relatively compliant, but potential for overdistension and volutrauma if pressure settings too high
Surgery/postsurgery
General aspects
Any infant with a painful surgical incision
Suppression of respiratory drive due to sedation. Limitation of respiratory excursion due to pain
(S)IMV
Medium
5–6
Low tidal volumes (< 5 mL/kg) may predispose to atelectasis
May not trigger effectively if heavily sedated
Abdominal surgery
Term infant – gastroschisis repair
Preterm infant – laparotomy for necrotising enterocolitis
Raised intra-abdominal pressure and diaphragmatic splinting
SIMV or AC
Medium–high
5–6
High PEEP needed to maintain EELV and recruitment
Where intra-abdominal pressure very high, may be poor response to conventional ventilation; HFOV or HFJV preferred
Thoracic surgery
Term infant – lobectomy for congenital lobar emphysema
Preterm infant – ligation of persistent ductus arteriosus
Lung compliance alterations intra-operatively; post-operative risk of air leak
SIMV or AC
Medium
4–5
Changes in both PEEP and V T may be needed intra-operatively
Lung disease
Diffuse alveolar disease
Preterm infant with RDS
Poor lung compliance, compliant chest wall
AC
High
4–5
Recruitment using PEEP increments may be helpful. Short T I and fast rate well tolerated
Term or preterm infant with haemorrhagic pulmonary oedema
Poor lung compliance
SIMV or AC
High
4–6
Recruitment using PEEP increments and fixed tidal volume may be helpful. Longer T I needed to help recruitment
Localised or heterogeneous disease
Preterm infant with nosocomial pneumonia
Term infant with meconium aspiration syndrome
Areas of low compliance with adjacent areas of relatively normal lung
SIMV or SIMV + PSV
Medium
5–6
Potential for overdistension of relatively normal lung regions. Fast ventilator rates may result in a T E that is too short for normal lung regions. Higher V T needed in meconium aspiration due to increased alveolar dead space
Inflammatory and/or fibrotic disease
Preterm infant with chronic lung disease
Regions of low compliance and increased resistance, especially expiratory resistance
SIMV + PSV or AC
Medium–high
5–7
Fast ventilator rates may result in a T E that is too short for the diseased lung regions with long expiratory time constants. Sufficient PEEP is needed to prevent expiratory flow limitation at low lung volumes
Pulmonary hypoplasia
Preterm infant born after early and prolonged rupture of membranes
Term infant with congenital diaphragmatic hernia
Low lung compliance related to small total lung volume. Overdistension of lungs at standard ventilator pressures
SIMV or AC
Low–medium
3–5
Hypoplastic lung is vulnerable to overdistension, stretch injury and high pulmonary vascular resistance. Avoid high V T/PIP. Consider high-frequency ventilation if PIP > 25 cm H2O, or if there is refractory hypoxic respiratory failure
Gas trapping/air leak
Preterm infant with pulmonary interstitial emphysema
Compression of normal airspaces by interstitial gas
SIMV
Medium
4–5
Low PEEP approach may lead to persistent hypoxaemia and respiratory acidosis. High-frequency ventilation (especially HFJV) is preferable
Pneumothorax (after drainage)
Continued leak of gas into the pleural space
SIMV or AC
Medium
4–5
Consider high-frequency ventilation (if available) where there is a torrential gas leak
Pulmonary insufficiency of prematurity
Extremely preterm infant in room air shortly after birth, failing extubation due to inadequate respiratory effort
Global immaturity of respiratory system, with low respiratory muscle capacity
AC or SIMV + PSV for weaning
Medium
4–5
Lungs become relatively compliant once atelectasis resolved, and there is potential for overdistension and volutrauma if pressure settings too high.
Persistent pulmonary hypertension
PPHN with parenchymal lung disease
Reduced pulmonary blood flow secondary to increased pulmonary arteriolar tone, exacerbated by low alveolar pO2 and pH
SIMV or AC
Medium
4–6
V T and PEEP requirements depend on associated parenchymal disease
PPHN with normal lung parenchyma
Reduced pulmonary blood flow secondary to increased pulmonary arteriolar tone
SIMV or AC
Low
4–5
Avoid hyperventilation
Cardiac disease
Left to right shunts
Preterm infant – patent ductus arteriosus
Term infant – large ventricular septal defect
Decreased lung compliance related to pulmonary engorgement and oedema
AC
High
5–6
Increasing CO2 can help limit blood flow to some degree
Vulnerable pulmonary circulation
Pulmonary atresia with duct-dependent pulmonary circulation
Hypoplastic left heart syndrome, post-Norwood operation
Pulmonary blood flow highly variable and under the influence of intra-alveolar pressure
SIMV
Low–medium
5–7
Lung overdistension with high-pressure settings will restrict pulmonary blood flow. Manipulation of pCO2 can help to control pulmonary blood flow – lower CO2 to promote blood flow, increase CO2 to restrict blood flow
Neuromuscular disease
Term infant with myotonic dystrophy
Low FRC and V T secondary to compromised respiratory muscle function
AC or SIMV + PSV
Medium
4–6
Appropriate to give mechanical support for each breath
Airway obstruction
Supra-glottic airway obstruction
Severe Pierre Robin sequence
Alveolar hypoventilation, normal lung compliance
SIMV
Low–medium
4–6
Glottic/sub-glottic airway obstruction
Term infant with oesophageal atresia and tracheomalacia
Alveolar hypoventilation, expiratory prolongation and gas trapping
SIMV
Low–medium if obstruction relieved, high otherwise
4–6
Settings influenced by whether the obstruction can be relieved by positioning of the endotracheal tube tip beyond the point of maximal narrowing
42.2 Choosing the Ventilator Mode
To a significant degree, the clinician’s choice of ventilator modes is limited by the equipment available in his or her NICU. Although most modern ventilators are capable of providing the basic modes of synchronised (patient-triggered) ventilation, there are a variety of hybrid modes or combinations that may be unique to each manufacturer and device. For example, some ventilators make possible the combination of synchronised intermittent mandatory ventilation (SIMV) and pressure support ventilation (PSV), and other ventilators employ PSV as a stand-alone mode with a backup rate, similar to assist/control (AC) but flow rather than time cycled. PSV eliminates the inspiratory hold, making for better synchronisation and automatically adjusting the effective inspiratory time (T I) as time constants change. However, this mode may not be appropriate in extremely small premature infants in the first few days of life, in whom respiratory time constants are so short that the actual T I may be less than 0.25 s. This duration of inspiration may be inadequate for gas mixing in the terminal air spaces and additionally may lead to excessively rapid respiratory rates.
Ventilators designed primarily for adult/paediatric patients but capable of also supporting neonates tend to have a greater variety of modes (some untested in newborn infants) and to offer volume-controlled ventilation. Unlike on the specialty neonatal ventilators, PSV on these devices does not have a backup rate and thus requires a reliable respiratory effort, not often present in preterm infants. However, when ventilating newborn infants, the clinician must be aware of the pitfalls of applying various modes to this unique population. The major limitation of volume-controlled ventilation is that the device only controls the volume delivered into the proximal (ventilator) end of the circuit, not the volume entering the patient’s lungs. Because of compression of gas and stretching of the circuit, as well as leak around uncuffed endotracheal tubes (ETT), there is only a very indirect relationship between set and delivered V T. For this reason, time-cycled, pressure-limited ventilation has been the standard ventilation mode in the NICU. There are several volume-targeted modes of pressure-limited ventilation with different operating principles and capabilities that more accurately control delivered V T in newborn infants. Some of these modalities have been studied in considerable detail and shown to reduce the incidence of hypocapnia, excessively large V T, duration of mechanical ventilation and to decrease levels of pro-inflammatory cytokines (Keszler 2009). Volume-targeted ventilation is discussed in detail elsewhere in this book. The more conventional modes are discussed below, and suggestions regarding the most appropriate modes for neonatal ventilation are incorporated in Table 42.1.
Despite many years of daily use, no consensus has emerged regarding the relative merits of the commonly used modes of synchronised ventilation. Guidance from large prospective trials with important clinical outcomes, such as incidence of air leak, chronic lung disease or length of ventilation, is lacking. Short-term clinical trials have demonstrated less tachypnoea and less variable V T (Mrozek et al. 2000), more rapid weaning from mechanical ventilation (Chan and Greenough 1994; Dimitriou et al. 1995) and smaller V T with smaller fluctuations in blood pressure (Hummler et al. 1996) with AC, compared to SIMV. There are important physiological considerations suggesting that SIMV may not provide optimal support in very premature infants. SIMV supports a user-defined number of breaths synchronised with the infant’s spontaneous respiratory effort. Spontaneous breaths in excess of the set rate are not supported, resulting in uneven tidal volume and high work of breathing during weaning. This is particularly important in extremely small and immature infants intubated with a narrow bore ETT (Fiastro et al. 1988). The high airway resistance of the ETT, limited muscle strength and mechanical disadvantage of the infant’s excessively compliant chest wall result in small, ineffective V T. Small breaths that largely rebreathe dead space gas will contribute little to effective alveolar ventilation. To maintain adequate alveolar minute ventilation, relatively large V T is thus required with the limited number of mechanical inflations provided by the ventilator. In a group of ELBW infants in the recovery phase of respiratory distress syndrome (RDS) ventilated with SIMV, the V T of mechanical inflations was about 6 mL/kg with spontaneous V T of 2.5 mL/kg, very close to anatomical dead space (Osorio et al. 2005). However, most clinicians continue to use SIMV, particularly during the weaning phase (Sharma and Greenough 2007). This is based on the intuitive assumption that fewer mechanical inflations are less damaging and on the traditional thinking that the ventilator rate must be lowered before extubation. However, because lung injury is primarily caused by excessive V T, irrespective of the pressure required to generate that V T (Dreyfuss and Saumon 1993; Hernandez et al. 1989; Dreyfuss and Saumon 1998), the smaller V T used with AC (Hummler et al. 1996) likely outweighs the faster rate. This concept is supported by the Oxford Region Controlled Trial of Artificial Ventilation study where a ventilator rate of 60 inflations per min compared to 20–40 was shown to result in less air leak with unsynchronised IMV (OCTAVE Study Group 1991). Many clinicians also mistakenly believe that assisting every breath prevents respiratory muscle training. This concern reflects a poor understanding of the patient–ventilator interaction during synchronised ventilation. With synchronised ventilation, the V T is generated by the combined inspiratory effort of the patient (the baby pulling air in) and the positive pressure generated by the ventilator pushing air in. The resulting transpulmonary pressure, together with the compliance of the respiratory system, determines the V T. Thus, as ventilator inflation pressure is decreased during weaning, the infant gradually assumes a greater proportion of the work of breathing and achieves respiratory muscle training. Ultimately, the ventilator pressure is decreased to the point when it only overcomes the added resistance of the ETT and circuit, at which point the infant should be extubated.
There is little difference between the modes during the acute phase of the disease when SIMV rate is relatively high. The differences become more meaningful during weaning, especially at rates <20. The less intuitive weaning process with AC appears to be one of the reasons clinicians continue to employ SIMV during weaning from mechanical ventilation. Another reason is the anecdotal observation that sometimes infants become tachypnoeic with AC and may have mild hypocapnia. This tachypnoea may reflect the infant’s response to low mean airway pressure (P AW) during weaning – shortening the expiratory time (T E) serves to maintain lung volume at end expiration. Tachypnoea appears to be infrequent when decreasing peak inspiratory pressure (PIP) is balanced by higher PEEP to avoid loss of lung volume. Low rate SIMV, especially in small infants with 2.5-mm-diameter ETT, is likely to impair the weaning process. Some ventilators offer the option of supporting spontaneous breaths with pressure support ventilation. This will compensate for the problems associated with simple SIMV but becomes a more complex process requiring the clinician to select settings for both SIMV and the level of pressure support. Nonetheless, more rapid weaning when SIMV was combined with PSV compared to SIMV alone has been demonstrated (Reyes et al. 2006). Based on these findings, it is recommended that when SIMV is used during the weaning process, PSV should be utilised to support spontaneous breathing. The alternate and less complicated approach is to use AC or PSV as a stand-alone mode to assist all spontaneous efforts and simply wean the inflations pressure, taking care to maintain adequate P AW. This is most easily accomplished when using volume-targeted modes of ventilation discussed earlier in this book. Finally, the attributes of the particular ventilator may influence the choice of triggered modes. Many flow-triggered devices are susceptible to auto-triggering due to leak around the ETT. This is more problematic with AC than with SIMV, where the mechanical breath rate is limited. Devices that have a very effective leak-adapted trigger and breath termination algorithms, such as the Draeger Babylog, are virtually immune from this problem and thus facilitate the use of AC.
42.3 First Settings
This section focuses on the initial management of the ventilated infant following intubation. Overall rationale for, and evidence supporting, choice of ventilator settings in newborns is set out in Sect. 42.4.
After intubation, a period of ventilation using a portable ventilation device will usually be required until the endotracheal tube is secured, nasogastric tube inserted, and the baby positioned in readiness for ventilation. In preterm infants, bolus surfactant may also be given at this time. If the ventilation device does not allow delivery of PEEP, the infant should be connected to the ventilator as quickly as possible.
The initial ventilator settings should take into account the context in which ventilation is being applied to the neonate (Table 42.1) and the apparent response to the pressure settings being used during ventilation with a portable device. There should be a stepwise approach to setting the ventilator at the time of initiation of mechanical ventilation (Table 42.2), guided in part by a thorough clinical appraisal (Table 42.3). PEEP should be considered first, with suggested PEEP levels for a range of different situations set out in Table 42.1. The T I should be set at around 0.5 s for a term infant and 0.35 s for a preterm infant, and then further adjusted depending on the analysis of the flow–time curve, which can be displayed on most modern neonatal ventilators. For most applications of time-cycled pressure-limited ventilation, T I should be set long enough to allow complete or near-complete inflow of gas into the lung during inspiration. If the T I is too short, there may not be sufficient time for an adequate tidal volume to be delivered nor for alveolar recruitment to occur with each tidal inflation. Setting PIP should be guided by a visual appreciation of chest rise and preferably the measured V T. Suggested target values for V T are set out in Table 42.1. Finally, T E (and thus, respiratory rate) is adjusted, with the aim of producing sufficient minute ventilation to clear CO2 without causing dynamic hyperinflation related to inadvertent PEEP. An estimate of minute ventilation can be made using the product of measured V T and respiratory rate, with a value of 200–300 mL/kg/min usually being an adequate starting point before the first pCO2 reading is obtained. Note that this estimate of minute ventilation does not incorporate dead space volume, which is substantial in some infants, particularly those with hyperinflated lungs (“alveolar dead space”). Additionally, rapid shallow breathing leads to high dead space to V T ratio and reduced alveolar ventilation. Avoidance of inadvertent PEEP can be difficult when an infant has a rapid spontaneous breathing rate and each breath is being supported by the ventilator. Examination of the flow–time curve during expiration will reveal whether gas outflow is complete before the next inflation begins and thus whether dynamic hyperinflation is likely to occur. If so, further adjustment to the mode of ventilation, respiratory rate and even inspiratory time may be necessary, as discussed below.
Table 42.2
Stepwise approach to initial ventilator settings
These parameters to be set in the order suggested |
PEEP – set according to context (see Table 42.1) and oxygen requirement |
T I – sufficient to allow complete gas inflow to occur (and longer, if tidal recruitment needed) |
PIP – sufficient to produce adequate chest movement (and V T, when measured) |
T E (or ventilator rate)a – set so as to allow complete gas outflow to occur and, in combination with V T, to produce adequate minute ventilation |
Table 42.3
Clinical evaluation after initiation of mechanical ventilation
Observation |
Colour and circulation |
Infant’s spontaneous respiratory rate |
Patient–ventilator interaction – evidence of triggering/autocycling |
Chest rise and diaphragmatic excursion |
Work of breathing |
Gastric distension |
Auscultation |
Breath sounds to all lung areas |
Adventitial sounds |
Large airway sounds |
Heart sounds |
Ventilation parameters |
Delivered tidal volume |
Flow–time curve – evidence of sufficient inspiratory and expiratory time |
42.3.1 Assessment After Going onto the Ventilator
A thorough clinical re-evaluation after initiation of ventilation is essential (Table 42.3), with the expectation that further adjustments to ventilator settings may be necessary. Careful note of the rate of spontaneous ventilation, and the effectiveness of triggering, should be made as the infant recovers from the intubation and the effects of any sedative and muscle relaxant drugs used prior to it. The need for further sedation/analgesia should be assessed. Muscle relaxation is rarely indicated in the era of effective synchronised ventilation. Narcotic analgesia should be used judiciously; clinical trial evidence suggests morphine administration relieves pain in ventilated neonates but may increase the risk of adverse neurological outcomes (Anand et al. 2004). If spontaneous breathing is to continue, the trigger sensitivity may need to be altered to optimise patient–ventilator interaction. In general, the trigger threshold should be as low as possible without causing autocycling, because higher trigger threshold is associated with higher work of breathing and longer trigger delay. An attempt should always be made to confirm whether “triggered” inflations are actually preceded by spontaneous respiratory effort or, alternatively, whether the ventilator is autocycling. The latter situation is more likely when there is a significant tube leak (despite “tube compensation”) (Bernstein et al. 1995), or with condensed water collecting in the ventilator tubing, and can lead to very significant hyperventilation particularly in AC mode.
Observation of the chest rise and abdominal motion will give some guide to the delivered V T, although this is a clinical skill that requires some time to learn (Aufricht et al. 1993), and may underestimate the actual V T (Schmolzer et al. 2010). High residual work of breathing may reflect inadequate tidal volume or inadequate minute ventilation, and ventilator settings should be adjusted appropriately. In the immediate post-intubation period, respiratory system compliance may be transiently decreased due to gaseous abdominal distension, especially after prolonged face mask ventilation. Venting of the stomach with a tube should occur soon after intubation to overcome any impairment of respiratory system compliance related to gastric distension with gas (Heldt 1988). Airway obstruction or ETT malposition should also be ruled out if the infant appears to be struggling.
Auscultation of the chest gives vital information in the intubated patient (Table 42.3) and should be conducted thoroughly and carefully after mechanical ventilation has been initiated. The intensity and distribution of breath sounds within the thorax gives information about the nature of the respiratory pathology and the effectiveness of ventilation. Uneven breath sounds (lower intensity over left hemithorax) suggest the possibility of tube malposition with the tip in the right main bronchus. The presence of a large airway noise which abates when the tube is pulled out a small distance adds further support. Large airway noise (low-pitched inspiratory noise) can relate to a build-up of secretions in the intrathoracic conducting airways and may also be transmitted from the glottic level in the presence of an endotracheal tube leak. If caused by tube leak, the noise will be of similar intensity (or louder) on auscultation with the stethoscope applied over the forehead (due to its extrathoracic origin). Applying cricoid pressure to ablate the leak provides additional confirmation.
The final component of the initial clinical assessment after commencing ventilation is an analysis of available ventilator data. Observation should be made over a number of inflations of the delivered V T for a set PIP or, conversely, the PIP required to deliver a set V T. If in a volume-targeted mode, the PIP limit may need to be increased if the desired V T is not being delivered. Analysis of the flow waveform will allow refinements of T I and expiratory time (T E).
After intubation and commencement of mechanical ventilation, a chest X-ray should always be performed to confirm the position of the endotracheal tube, to assess the state of the lung parenchyma and to gain an appreciation of the degree of lung inflation. Given that chest X-rays are taken at peak inflation, the degree of lung inflation will be a reflection of end-inspiratory lung volume (EELV plus V T), and thus does not help significantly in titrating PEEP. For this reason, some investigators have chosen to perform chest X-rays at end expiration (Pohlandt et al. 1992), but this is not currently a widespread practice.
42.3.2 Monitoring and Documentation During Mechanical Ventilation
With the need for mechanical ventilation comes the need for intensive monitoring and careful charting of physiological and ventilatory parameters. All mechanically ventilated infants should have, at the very least, cardiorespiratory monitoring, continuous measurement of oxygen saturation (SpO2) via a transcutaneous pulse oximetry probe and temperature monitoring (Table 42.4). An intra-arterial catheter to continuously monitor blood pressure and allow periodic arterial blood gas sampling is essential in the unstable and critically ill infant and highly desirable in any infant requiring mechanical ventilation. A continuous estimate of pCO2 derived from transcutaneous monitoring or end-tidal gas sampling is also valuable, whether or not an arterial line is in place. Because ET CO2 monitoring adds significantly to the instrumental dead space and is often inaccurate in small infants whose rapid respiratory rates do not allow an end-tidal plateau to be reached, transcutaneous monitoring is to be preferred in most ventilated neonates.
Table 42.4
Monitoring of the ventilated infant
Physiological monitoring |
Essential |
Cardiorespiratory monitoring (numeric display of heart rate and respiratory rate, with preferably a real-time display of 3-lead ECG wave and respiratory impedance tracing) |
Transcutaneous SpO2 (numeric display with preferably a plethysmographic SpO2 tracing) |
Temperature |
Desirable |
Intra-arterial catheter placement for continuous blood pressure monitoring and intermittent arterial blood gas sampling |
Transcutaneous pCO2 (+/− pO2) |
End-tidal CO2 (infants beyond term) |
Ventilation parameters to be monitored and recorded |
Essential |
Delivered ventilator pressures (PEEP, PIP and P AW) |
T I |
Respiratory rate (mechanically assisted and total) |
V T (breath by breath, preferably the expired V T measured at the airway opening) |
%leak (difference between inspired and expired V T, expressed as a percentage of the inspired V T) |
Humidifier temperature |
Desirable |
Ventilator waveforms (time scalars of pressure and flow) |
Compliance and resistance measurements |
Tidal pressure–volume curves |
Ventilation parameters that should be monitored and recorded include ventilator pressures and timing and the total breath number and number of mechanically assisted breaths per minute (Table 42.4). The delivered V T (and the target V T, if set) should be noted, along with the proportion of leak. To be accurate, V T must be measured at the airway opening, not at the ventilator end of the circuit. Whilst some devices offer a compliance compensation feature that corrects for the loss of V T in the circuit, this feature does not function well in the presence of ETT leak. The humidifier temperature should be regularly checked and recorded. Most modern ventilators also provide continuous display of waveforms and/or tidal ventilation loops, and these, along with breath-by-breath compliance and resistance measurements, can be very helpful in fine-tuning ventilator settings, as described in this and other chapters in this text.
42.4 Ventilator Settings and Strategies: Pathophysiological Rationale, Meta-analytic Evidence and Therapeutic End Points
42.4.1 Manipulating End-Expiratory Lung Volume
Physiological first principles, and a large body of experimental and clinical evidence, point to the importance of manipulating EELV during mechanical ventilation of the diseased lung. It has become clear that to apply ventilation optimally requires a well-recruited lung with adequate end-expiratory volume (Rimensberger 2002; Clark et al. 2000). Such an approach is dependent on the application of sufficient PEEP, and the physiological considerations that guide PEEP setting are discussed in this section.
Clinical and experimental data point to a clear relationship between PEEP and EELV and, thus, oxygenation. Changes in other ventilator settings may, however, potentially lead to alterations in EELV. A long-held belief was that an increase in P AW by alteration of any combination of ventilator settings would have a similar impact on oxygenation and thus that there should be an optimal P AW at which to apply ventilation (Boros et al. 1977). It is now becoming accepted that the components that make up the P AW (PIP, PEEP, T I, rate and gas flow) should be considered separately. As stated by Monkman and Kirpalani:
In retrospect, the simplifying but reductionist elegance that focused upon a single unitary number [PAW] may have misled the field. (Monkman and Kirpalani 2003)
Thus, an improvement in oxygenation after a PEEP increase should not be viewed as having occurred simply because the P AW is higher but rather because EELV has increased and
matching has improved. Despite also producing P AW changes, alteration in other ventilator parameters does not have the same effect on EELV (Fig. 42.1) (Thome et al. 1998) nor on oxygenation (Stewart et al. 1981). The inextricable linkage between PEEP, EELV and oxygenation dictates that it is PEEP that is the first, and arguably the most important, ventilatory parameter to be considered in the continuing management of the ventilated neonate.
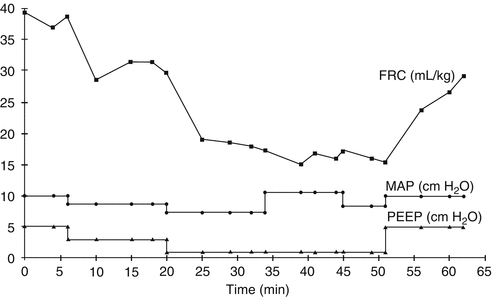

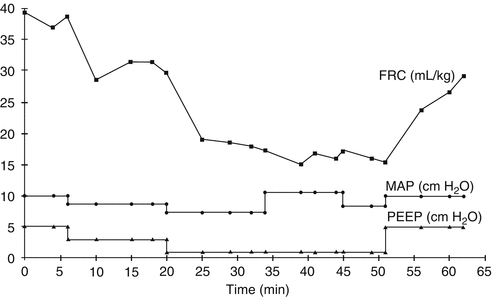
Fig. 42.1
Effect of ventilation changes on end-expiratory lung volume. Changes in end-expiratory lung volume (EELV, denoted as functional residual capacity, FRC) during programmed manipulation of ventilator settings in a ventilated infant. EELV is seen to reduce with stepwise reductions in positive end-expiratory pressure (PEEP) and thus mean airway pressure (P AW, denoted as MAP) during the first 30 min. At minute 34, inspiratory time is lengthened from 0.3 to 0.6 s, leading to an increase in P AW, but no significant change in EELV. Conversely, when PEEP is increased (minute 52), there is a substantial improvement in EELV (Reproduced from Thome et al. (1998) with permission)
An important difficulty in manipulating EELV in the ventilated subject is that the available methods to measure EELV at the bedside are imprecise and in some cases impractical (Dargaville and Rimensberger 2010). Whole-body plethysmography (Stocks et al. 2001) and gas dilution (Sivan et al. 1990; Vilstrup et al. 1992), both of which give absolute values for EELV, are not appropriate for repeated measurements in a clinical setting. Other methods, including respiratory inductance plethysmography (Adams 1996; Emeriaud et al. 2010), fibre-optic plethysmography (Davis et al. 1999) and electrical impedance tomography (Frerichs et al. 2001), allow measurement not of absolute volume but of change in EELV relative to a baseline condition, and only with prior calibration can a gas volume change in mL be derived. Despite these limitations, a great deal of information has been obtained from experimental studies in which EELV has been measured, either at the bedside or in the laboratory, as alterations in PEEP have been made. The key findings of this work are set out below. This knowledge can be used by clinicians to set and titrate PEEP in ventilated subjects, aided by indirect measures of EELV, in particular oxygenation and tidal breath compliance.
42.4.1.1 Relationships Between PEEP, EELV and Recruitment
An understanding of what can potentially be achieved through manipulation of lung volume using PEEP comes from firstly examining the changes in EELV and recruitment during stepwise PEEP manoeuvres. Such information is derived from lung modelling, studies in experimental animals and in humans with lung disease. An example is shown in Fig. 42.2. With stepwise PEEP increments and a constant inflating pressure (PIP–PEEP), a surfactant-depleted lung starting at residual volume follows the path of the inflation limb of the pressure–volume (PV) relationship (Fig. 42.2), with an improvement in tidal breath compliance as more non-aerated lung units are recruited, followed by a fall in compliance as more lung units become overdistended near total lung capacity. During PEEP decrements (Fig. 42.2), the property of hysteresis is demonstrated with a different PV trajectory than during PEEP increments, following a path closer to the deflation limb of the PV relationship. There is preservation of recruitment through a large proportion of this trajectory, and the open tidal ventilation loops reflect the marked improvement in compliance during PEEP decrements, as has been predicted by lung modelling (Hickling 2001). At the point of maximal curvature of the deflation limb (“closing pressure”), the lung is still well inflated, recruitment is largely preserved and tidal ventilation at this point is associated with minimal overdistension and a peak in compliance. This is the point of “optimal PEEP”, at which the lung is ventilated with the best possible oxygenation and compliance and with the least possible pressure (Rimensberger et al. 1999a). Additional advantages of optimising PEEP in this way are shown in Table 42.5. In the experimental animal with recruitable lungs, PEEP set in relation to the point of maximal curvature of the deflation limb is often at or below the PEEP value predicted from the lower inflection point of the inflation limb (Rimensberger et al. 1999a).
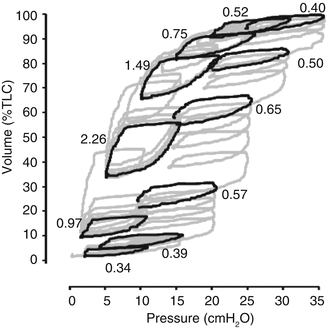
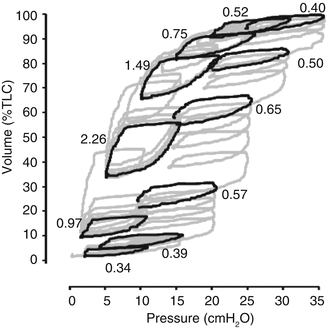
Fig. 42.2
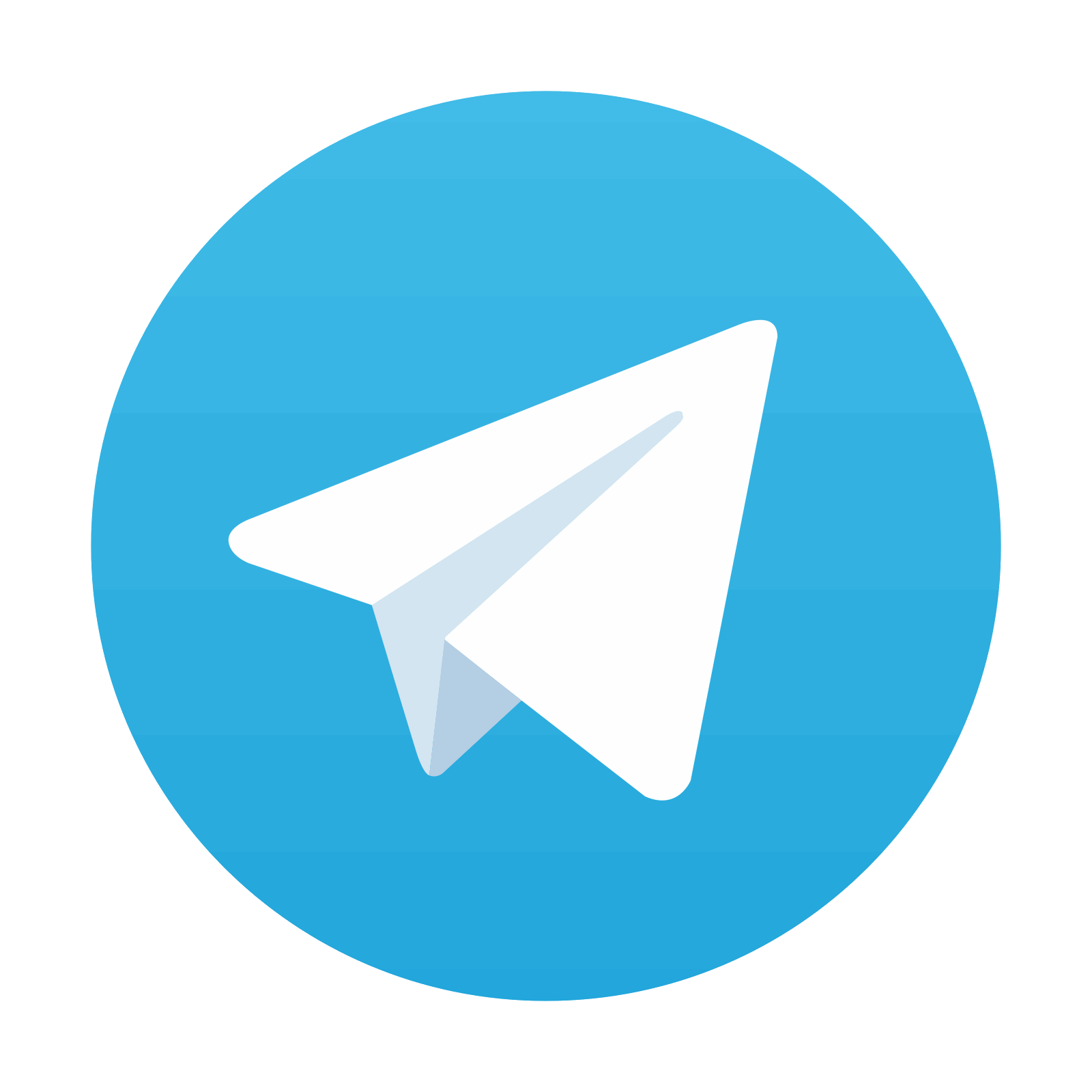
Stepwise vital capacity manoeuvre in the injured lung. Pressure–volume curve during a stepwise vital capacity manoeuvre using positive end-expiratory pressure (PEEP) increments and decrements in a mechanically ventilated 2.1 kg piglet after repeated saline lavage. Each PEEP level was held for 15 s. Inflating pressure (peak inspiratory pressure – PEEP) remained at 10 cm H2O throughout. Y-axis shows lung volume measured by respiratory inductance plethysmography and scaled to total lung capacity (TLC). Complete tracing is shown in grey and the final breath at each PEEP level in black, and the tidal breath compliance (mL/cm H2O/kg) for each of the final breaths is indicated in italics (Data derived from same experimental series as reference (Dargaville et al. 2010))
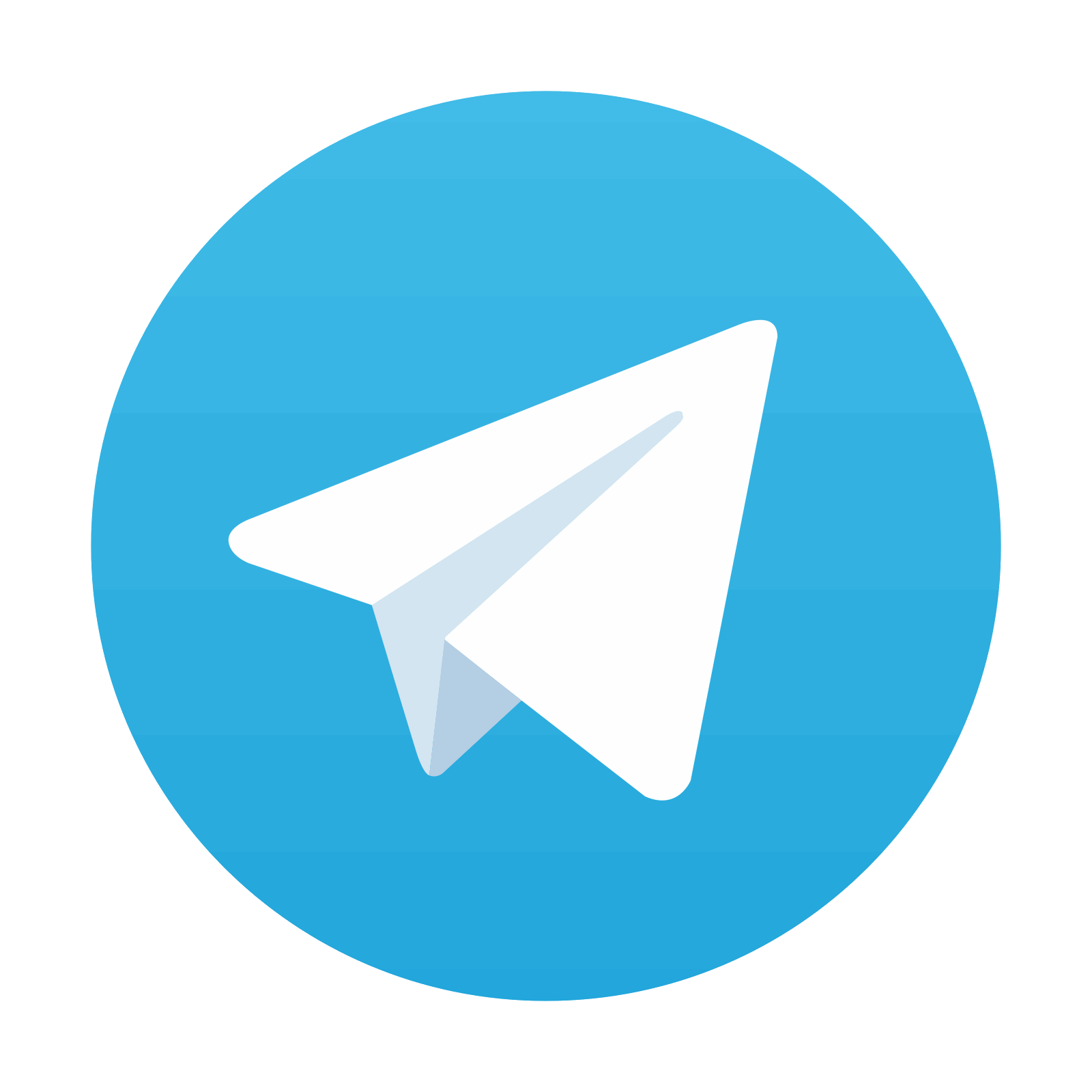
Stay updated, free articles. Join our Telegram channel

Full access? Get Clinical Tree
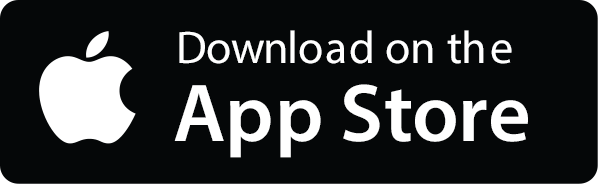
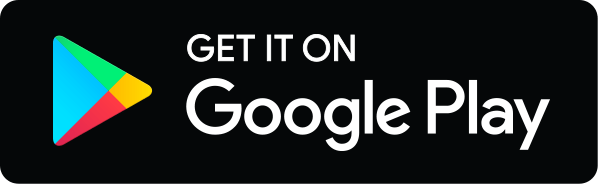
