Definition, Epidemiology, and Genetics
Arthrogryposis multiplex congenita (AMC), or arthrogryposis, and multiple congenital contractures (MCC) are equivalent terms used to describe a phenotypic condition associated with multiple congenital non-progressive contractures in three or more body sites. Arthrogryposis is not a single disease but rather a clinical feature associated with more than 300 conditions [1]. The contractures develop secondary to fetal akinesia, i.e., in utero movement limitation, of varying etiology: muscle and/or neuronal pathology, connective tissue abnormalities, external limitations of in utero space, maternal diseases or exposures, compromised blood supply of placenta and/or fetus, metabolic disorders, and epigenetic influence. Children are born immobilized with fixed and thickened joints and decreased muscle mass. Early medical care may improve the condition over time [1, 2].
Arthrogryposis occurs in 1 in 3,000 to 4,300 livebirths. Amyoplasia, the most common type of arthrogryposis, has an incidence of 1 in 10,000 births, with a higher prevalence in twin pregnancies. Arthrogryposis has equal sex distribution, though arthrogryposis associated with X-linked disorders is more common in males [1, 2, 3].
Genetic alterations, like single-gene mutations or chromosomal disorders, are responsible for 30% of arthrogryposis cases, and over 150 genes have been associated with the condition [1]. The inheritance patterns are diverse, including autosomal recessive (often associated with CNS dysfunction and severe fetal akinesia sequence); autosomal dominant (common in the distal arthrogryposis); X-linked; and mitochondrial disorders inherited in maternal pattern [4].
Clinical Features and Differential Diagnosis
A diagnosis of arthrogryposis is established when three or more body sites are affected with congenital joint contractures, mostly in the limbs; however, the jaw and spine may also be involved. Arthrogryposis may affect all four limbs, three limbs, or may be limited to only upper or lower extremities. The condition may be classified according to the etiology by several features: the involvement of the nervous system, localization of contractures, and their specific combinations [1, 2]. Therefore, arthrogryposis is divided into amyoplasia (congenital, non-hereditary, multiple contractures); distal arthrogryposis (10 types of multiple hereditary contractures); and syndromic arthrogryposis (contractures developing secondary to the limitation of movement caused by a specific primer syndrome) [1, 2].
Amyoplasia
Amyoplasia, sometimes also called classical or canonical arthrogryposis, is a condition characterized by decreased muscle mass in the limbs with a fatty-fibrous replacement of the muscular tissue. The affected limbs are 5–10% shorter compared to normal, usually affected in a symmetrical pattern, with intact sensation and decreased reflexes [3]. Characteristic signs are also lack of flexion creases and large deep dimples overlying the affected joints. Children with amyoplasia often suffer an intrauterine growth restriction and are born with a low weight (Figure 49.1).
Amyoplasia accounts for almost 1/3 of all cases of arthrogryposis, and is clinically recognizable by the following signs: internally rotated and adducted shoulders; fixed extended elbows; flexed and ulnarly deviated wrists; camptodactyly; dislocated hips; extended knees; and equinovarus deformity of the feet [3, 4]. A common feature in amyoplasia is a mid-facial hemangioma, or nevus flammeus, observed in 95% of cases. About 12% of children with amyoplasia have small and/or absent fingers and toes or absent hand or foot, while 10% present with gastrointestinal abnormalities (gastroschisis and bowel atresia); 9% with trunk defects (deficiency in abdominal and/or thorax musculature and fascia); and 8% with indentations in the limbs due to umbilical cord wrapping. Children with amyoplasia have gracile, osteoporotic long bones making them prone to iatrogenic fractures, particularly perinatally. The majority of affected children present with normal intelligence [3, 5, 6, 7].
Figure 49.1 Child with amyoplasia. In the upper extremity, the shoulders are internally rotated, the elbows are extended, the wrists are flexed and ulnarly deviated, the fingers are stiff, and the thumbs are positioned in the palm. In the lower limbs, the hips may be dislocated, the knees are extended, and the feet have severe equinovarus contractures.
Distal Arthrogryposis
Distal arthrogryposis (DA) is a group of 10 autosomal dominant syndromes that affect distal joints of the limbs with variable clinical presentations [4, 6]:
-
DA type 1 – Camptodactyly and clubfoot; hypoplasia and/or absence of some interphalangeal creases; shoulders and hips are less frequently affected.
-
DA type 2 A (Freeman-Sheldon syndrome) – Hands and feet are affected as in DA1 with the addition of flexion and ulnar deviation of fingers and scoliosis. Syndrome is also characterized by oropharyngeal abnormalities, including a distinctive facial appearance (termed “whistling-face”): very small mouth (often only a few millimeters in diameter at birth), puckered lips, and an H-shaped dimple of the chin (Figure 49.2).
-
DA type 2B – Hands and feet affected as in DA1 including more prominent nasolabial folds, downslanting palpebral fissures, and a small mouth.
-
DA type 3 – Symptoms of DA1 with the addition of cleft palate and short stature.
-
DA type 4 – Symptoms of DA1 with the addition of scoliosis.
-
DA type 5 – Symptoms of DA1 with the addition of ophthalmoplegia, ptosis, and/or strabismus.
-
DA type 6 – Symptoms of DA1 with the addition of sensorineural hearing loss.
-
DA type 7 – Trismus (inability to fully open the mouth), pseudocamptodactyly, shortened hamstring muscles, and short stature.
-
DA type 8 – Autosomal dominant multiple pterygium syndrome.
-
DA type 9 – Congenital contractural arachnodactyly.
-
DA type 10 – Congenital plantar contractures.
A common feature in pregnancies resulting in arthrogryposis is oligohydramnios or deficiency of the amniotic fluid that exposes the fetus to additional uterine pressure. Pregnancies with boys are associated with oligohydramnios twice more often than pregnancies with girls. Oligohydramnios is often accompanied by renal agenesis, specific skin changes, dolichocephaly, and Potter facies, characterized by ocular hypertelorism, abnormal ear lobation, micrognathia, and flattened nasal tip [8].
Pena-Shokeir phenotype or fetal akinesia deformation sequence (PSP/FADS) is another subtype of multiple congenital contractures, often associated with polyhydramnios, that present with classic signs of intrauterine growth restriction, craniofacial anomalies, limb contractures, including other disorders such as pulmonary hypoplasia, short umbilical cord, and decreased intrauterine fetal movement. This phenotype includes 20 forms that may be inherited in an autosomal recessive manner, and are in some cases caused by mutations in the RAPSN or DOK7 genes [9].
Figure 49.2 Child with distal arthrogryposis type 2A (Freeman-Sheldon syndrome).
Differential Diagnosis
Arthrogryposis manifests in more than 300 disorders, and the underlying diagnosis may be the result of neurogenic or myopathic processes, connective tissue disorders, intrauterine compression, vascular insult, or teratogenic exposure. In newborns with arthrogryposis, the diagnostic challenge is thus to thoroughly assess the fetal condition, associated abnormalities, and family history. A multidisciplinary approach incorporating obstetrics, genetics, pediatric neurology, and fetopathology is often required to reach the most accurate diagnosis and provide appropriate counseling and therapy [5, 7].
Imaging
Ultrasound may be used to monitor fetal movements where spontaneous spikes of the motion signify normal fetal development in the first trimester, while disturbances in spontaneous movements may be a sign of maldevelopment of the neuronal and/or muscular component [5].
Although not routinely used, CT may be a useful diagnostic tool in cases of neurogenic and/or myopathic genesis of arthrogryposis. CT imaging can be used to visualize bone abnormalities, including gracile bones, synostosis, ankyloses, extra or missing bones, skeletal dysplasia, and scoliosis; CNS malformations (i.e., ventriculomegaly); and muscle tissue pathology [4, 10].
Dynamic MRI scans are used to evaluate limb movements, gross fetal motions, swallowing, and diaphragmatic motions. Cross-relaxation MRI may be used to visualize muscle tissue and fibrous fatty tissue replacement [11].
Laboratory Findings and Prenatal Diagnosis
The prenatal detection rate of multiple congenital contractures is estimated by some authors to be as low as 25% of all cases of arthrogryposis. This can be in part ascribed to the fact that the evaluation of fetal movements is not a part of a routine in utero ultrasonography (USG) [11]. Usually, fetal movements are evaluated only when a pregnant woman is concerned about the lack of fetal motility or a regular USG scan reveals abnormal features that raise suspicion of arthrogryposis. Nonetheless, women with a positive family history, and who are at an increased risk of development of arthrogryposis in their children, undergo USG screening with fetal movement assessment at 14, 16, 18, and 20 gestational weeks, as well as in the middle of the second trimester [5].
The first-trimester USG exam with the following findings may direct the clinical search towards the arthrogryposis [4, 5]:
-
Total fetal akinesia, talipes and bilateral fixed flexion deformities of the hands, wrists, elbows, and knees, abnormal flexion of the hips and extension of the knees;
-
Increased nuchal translucency (as a feature of associated syndromes);
-
Cystic hygroma.
The second-trimester abnormal USG findings pointing to arthrogryposis are:
-
Joint contracture of all the extremities with clenched hands, clubfeet, and, essentially absent fetal movements, often associated with polyhydramnios and pulmonary hypoplasia;
-
Cystic hygroma associated with pleural effusion;
-
Fetal hydrops;
-
Multiple congenital anomalies associated with decreased fetal mobility and multiple joint contractures.
The third-trimester USG findings are:
-
Decreased fetal movement;
-
Micrognathia;
-
Polyhydramnios;
-
Hypoechogenicity;
-
Hypomineralization of the long bones;
-
Pleural effusions;
-
Ventriculomegaly;
-
Hydronephrosis;
-
Collapsed stomach.
Laboratory findings in pregnant women are unspecific and therefore do not contribute towards the diagnosis of arthrogryposis. Laboratory tests in newborns may include serum measurement of creatine phosphokinase (CPK) levels in myopathic disorders; immunoglobulin M levels in viral infections; and maternal antibodies against neurotransmitters in myasthenia gravis [4].
Macroscopy, Histopathology, and Genetic Testing
A clinico-pathological study on lethal arthrogryposis in Finland has reported on 83 cases that were either stillborn fetuses; a termination of pregnancy following prenatal diagnosis; or died within 28 days after birth. The majority of cases (67, or 80.7%) were neurogenic in origin, including 41 (49.4%) with the autosomal recessive lethal congenital contracture syndrome (LCCS, McKusick 253310); 15 (18%) had milder anterior horn cell involvement, and 10 (12%) had CNS degeneration or dysgenesis. Congenital muscular dystrophy and nemaline myopathy were observed in 2 and 1 cases, respectively, while a non-neuromuscular basis was confirmed in 10 (12%) cases. In addition to the LCCS, in two families arthrogryposis recurred twice, therefore also pointing to the likelihood of autosomal recessive inheritance [12].
Another report analyzed a series of 20 fetuses (16 to 32 gestational weeks), 19 of which were post termination of pregnancy following prenatal diagnosis and 1 was a stillborn. Decreased fetal movement was the most frequently observed USG finding. The most common gross deformities of the upper extremities were 10 flexion contractures of the elbow joints (50%), 6 short clavicles (30%), and 2 clenched hand deformities (10%). The most common skeletal deformities of the lower extremities were 9 talipes equinovarus deformities (45%), 3 narrow pelvises (15%), and 6 flexion contractures (30%). Scoliosis was found in 2 cases (10%), and the other 2 had vertebral fusion abnormalities [13].
Genetic testing was successful in 13 cases in this series, including two AMC; two trisomy 13; two trisomy 18; and one each of triploidy 69, XXY; osteogenesis imperfecta; lethal multiple pterygium; Saldino-Noonan syndrome; perinatal lethal hypophosphatasia; Beckwith-Wiedemann syndrome; and one teratogenic drug effect. Consanguineous marriage, oligohydramnios, maternal illicit drug addiction, and teratogenic exposure, were among identified risk factors [13].
Prognosis, Future Direction, and Therapy
The prognosis of arthrogryposis largely depends on the type and severity of the contractures. In early childhood, children with arthrogryposis require constant parental support and care. Two main goals of the arthrogryposis treatment are early stable ambulation and improving self-care. To make this possible, it is important to gently manipulate on affected joints soon after birth to improve passive and active range of motion [4]. All patients with multiple congenital contractures reach motor milestones later and show much lower activity levels compared to healthy children of the same age. Nonetheless, due to advanced physical therapy, orthopedic procedures, and surgical interventions, today it is possible to considerably improve the quality of life of affected children [14, 15]. With timely provided and appropriate medical care, individuals with amyoplasia can be independent of caregivers by the age of five in 85% of cases; 64% are age-appropriate in school; 75% are independent for feeding; 35% for toileting; 25% for bathing; 20% for grooming; and 10% for dressing [15].
Children with distal arthrogryposis and intact cognition are able to achieve functional ambulation in 100% of cases after the surgical correction of lower limb deformities. Surgical interventions include tendon release, lengthening of the extensor, repair of club feet, traction and casting, and distraction osteogenesis. Upper-limb function and independent living skills are generally excellent, with appropriate vigorous physical therapy, surgical muscle transfers, and mental support [16, 17].
References
Definition
Spinal muscular atrophy (SMA) refers to a group of progressive neurodegenerative conditions primarily involving spinal anterior horn cells, as well as select bulbar motor neurons. Onset may be in infancy, childhood, or teen years (historically classified into Types 1, 2, and 3; see Table 50.1). Adult-onset (type 4) SMA is not discussed further here.
Type | Age of onset | Lifespan | Overall motor function | Comment |
---|---|---|---|---|
0 (Prenatal) | Birth | Weeks | Little to none | May have arthrogryposis; Respiratory failure at delivery |
1 (Werdnig-Hoffman) (OMIM #253300) |
Birth (1A) |
6-24mos (80% die within 1yr) |
Sitting never achieved, or only with support | May have arthrogryposis |
<3mos (1B) | ||||
>3mos (1C) | ||||
2 (OMIM #253550) |
<18mos | 2yr-2nd decade or later | Sitting, but little or no ambulation (wheelchair use) | |
3 (Kugelberg-Welander) (OMIM #253400) |
<3 yrs (3A) | Approaching normal adult | Ambulation, but with proximal weakness, gait difficulty | |
>3 yrs (3B) | ||||
4 (OMIM #271150) |
Adulthood | Approaching normal adult | Attainment and later loss of motor abilities |
Epidemiology
SMA linked to abnormality in chromosome 5 (the most common type; discussed later) has a prevalence of about 1–2 per 100,000 individuals, and an incidence of approximately 1 in 10,000 live births [1]. Type 1 SMA (see Table 50.1) comprises about 60% of all cases. These values, along with carrier frequencies, vary greatly by geographic locale and ancestry.
Clinical Features
In all subtypes of spinal muscular atrophy, the main clinical manifestations result from denervation atrophy of skeletal musculature of the limbs and diaphragm, often with relative sparing of facial and extraocular muscles [1–3].
The clinical phenotype of SMA type 1 (SMN1), arising in the neonatal and early infantile period, is quite typical, consisting of hypotonia (“floppy infant”), with a “frog leg” posture when supine, and head lag when lifted. Weakness is symmetric, more pronounced in proximal muscles, and in legs greater than arms. In SMA 2 and 3, arrest (or sometimes loss) of motor milestones occurs at a later age and more variable pace. Neurologic examination in types 2 and 3 reveals tongue fasciculations, in addition to the proximal weakness as seen in type 1.
For the earliest onset (type 1; SMN1), lifespan is significantly shortened. Common to types 1 and 2 is weakness of pharyngeal, intercostal, axial, and proximal appendicular muscles, leading to progressive respiratory insufficiency and risk of aspiration or other intercurrent infection. The weakness may be only slowly progressive, or even static, in types 2 and 3, allowing normal lifespan.
Further classification has been increasingly based on genotype, including mode of inheritance (see the further discussion, and Table 50.2).
Type (OMIM #) | Gene | Locus | Inheritance | Phenotype | Clinical features | Allelic disorders |
---|---|---|---|---|---|---|
SPSMA (181405) | TRPV4 | 12q24.11 | AD | Scapuloperoneal spinal muscular atrophy | Progressive scapuloperoneal muscle weakness, laryngeal palsy | Congenital dSMA, CMT2 C, AD |
SMALED1 (158600) | DYNC1H1 | 14q32.31 | AD | Lower extremity-predominant spinal muscular atrophy-1 | Muscle weakness affecting proximal lower extremities and sparing upper limbs | CMT2O, malformations of cortical development, mental retardation |
SMALED2 (615290) | BICD2 | 9q22.31 | AD | Lower extremity-predominant spinal muscular atrophy-2 | Muscle weakness affecting proximal lower extremities and sparing upper or early-onset contractures, upper motor neuron involvement | Late-onset HSP |
LAAHD (611890) | GLE1 | 9q34.11 | AR | Arthrogryposis with anterior horn cell disease | Fetal immobility, hydrops, micrognathia, pulmonary hypoplasia, pterygia and multiple joint contractures, prenatal akinesia | Lethal congenital contracture syndrome 1 |
SMAX2 (301830) | UBA1 | Xp11.23 | XR | Lethal infantile spinal muscular atrophy, with arthrogryposis | Hypotonia, areflexia, chest deformities, facial dysmorphic features, congenital joint contractures, bone fractures, genital abnormalities | |
SMAPME (159950) | ASAH1 | 8p22 | AR | Spinal muscular atrophy with progressive myoclonic epilepsy | Refractory to treatment myoclonic epilepsy, dysphagia, respiratory muscle weakness | Farber lipogranulomatosis |
PCH1A (607596) | VRK1 | 14q32.2 | AR | Pontocerebellar hypoplasia with infantile spinal muscular atrophy | Pontocerebellar hypoplasia, microcephalia, mental retardation, early death | |
PCH1B (614678) | EXOSC3 | 9p13.2 | AR | Pontocerebellar hypoplasia with infantile spinal muscular atrophy | Pontocerebellar hypoplasia, microcephalia, mental retardation, early death | |
BVVLS1 (211530) | SLC52A3 | 20p13 | AR | Brown-Vialetto-Van Laere syndrome 1 | Ponto-bulbar palsy, bilateral sensorineural hearing loss | Fazio-Londe disease |
BVVLS2 (614707) | SCL52A2 | 8q24.3 | AR | Brown-Vialetto-Van Laere syndrome 2 | Ponto-bulbar palsy, bilateral sensorineural hearing loss | Fazio-Londe disease |
AD = autosomal dominant; AR = autosomal recessive; CMT = Charcot-Marie-Tooth; XR = X-linked recessive; HSP = hereditary spastic paraplegia; dSMA = distal SMA. (Modified from [2].)
Genetics
Chromosome 5q11.2–13.3 is the locus of the survival motor neuron gene (SMN), which exists as two alleles (SMN1 and SMN2), differing by a single nucleotide transition in exon 7, leading during transcription to reduced levels of functional SMN protein. The vast majority of individuals with SMA have mutations in SMN1, and may even have asymptomatic family members sharing the same haplotype. Among those caused by homozygous deletions or mutations of chromosome 5q11.2–13.3, the tempo of progression and severity of disability vary widely (see Table 50.1) [1–4]. Modifier genes such as neuronal apoptosis inhibitory protein gene (NAIP) may therefore play an important role in the phenotype in a given patient.
Non-5q forms of SMA account for about 5% of all SMA cases and include an autosomal dominant form which is lower-extremity predominant (SMALED1), due to mutations of the DYNC1H1 gene on 14q32.31 [2]. Onset has been described to occur as early as the first two years of life, resulting in some motor delay, but lifespan is not necessarily shortened. SMALED2A and -2B are similar but have mutation in BICD2 on chromosome 9q22 [2]. Other non-5q forms of spinal muscular atrophy are also recognized: as of this writing, at least 16 different genes have been associated with proximal non-5q spinal muscular atrophy phenotypes (Table 50.2) [2]. The complexity of the genetic underpinnings of SMA, as well as its many associations with other phenotypes such as pontocerebellar hypoplasia (sometimes called “SMA plus syndrome”), is beyond the scope of this text. Of note, the recent availability of clinical testing for mutations in SMN has resulted in a marked decrease in skeletal muscle biopsy for the diagnosis of SMA.
Laboratory Findings and Prenatal Diagnosis
Laboratory testing in affected individuals shows slight to moderate increases in serum creatine kinase in types 2 and 3. Electromyography highlights neurogenic features of spontaneous fibrillations and polyphasic potentials. Nerve conduction velocities, including in sensory nerves, may be diminished [3].
Prenatal testing using chorionic villus sampling, or collection of fetal DNA by amniocentesis, as well as preimplantation analysis during in vitro procedures, are available [3].
Macroscopy
Autopsy examination of the spinal cord of an affected patient may disclose thinning and grayish discoloration of the anterior roots, compared to dorsal roots (Figure 50.1). The brain is generally normal macroscopically.
Figure 50.1 Spinal muscular atrophy (SMA). Ventral (motor) spinal rootlets are grossly atrophic in comparison to dorsal (sensory) spinal rootlets of the cauda equina (A). Paraffin sections stained with H&E (C, D) and plastic sections stained with toluidine blue (E, F) show that the ventral rootlet atrophy is due to a loss of axons (C and E), while dorsal rootlets remain normal (D and F). There is a paucity of anterior horn neurons in H&E-stained paraffin sections from the lumbar spinal cord (B). In the inset from a normal control anterior horn, several lower motor neuron cell bodies are present. Frozen sections of quadriceps muscle stained with H&E demonstrate grouped abnormally small (hypotrophic) and grouped hypertrophic skeletal muscle fibers (G). Grouped hypertrophic fibers are type I (H; slow myosin immunohistochemistry).
Histopathology, Genetic Testing
Microscopically, the anterior horn cells and bulbar motor neurons are reduced in number, with residual neurons showing chromatolytic changes. Rarely, neuronophagia is observed. Gliosis is present but may be slight relative to the degree of neuronal loss. Other affected nerve cells include those in the thalamus, Clarke’s column, dorsal root ganglia, and rarely the motor cortex. Anterior spinal nerve roots have reduced caliber and overall number of myelinated axons, with scattered myelin digestion chambers and variable endoneurial collagen deposition. In cases with dorsal root ganglia involvement, the posterior columns may show degenerative changes as well.
The skeletal muscle, whether sampled during life or at post-mortem examination, shows a striking pattern of denervation in affected neonates and infants, the atrophic fibers having rounded rather than angulated contours (the latter pattern one sees in older children and adults), interspersed with hypertrophic fibers (Figure 50.1). The atrophic fibers may be of type 1 on enzyme histochemical stain, reminiscent of congenital fiber-type disproportion (see Chapter 54). In longer-standing disease, classical group atrophy and fiber-type grouping are notable. As a rule, atrophic type 2 fibers will predominate, and type 1 fibers may be hypertrophic. Immunohistochemistry for slow myosin highlights the larger (spared) fibers. In much later time-points, there may be appreciable endomysial connective tissue deposition with adipocyte proliferation (i.e., myopathic appearance in end-stage neurogenic atrophy; Figure 50.1).
Differential Diagnosis
In SMA types 2 and 3, the presence of small type 1 fibers may suggest a congenital or early-onset myopathy, such as nemaline myopathy, congenital fiber-type disproportion, or even muscular dystrophy (see Chapter 53). “Atypical” forms of SMA include those with cerebellar hypoplasia (VRK1 mutation) and nerve deafness and palsies of the lower cranial nerves (SLC52A3). Genetic testing, if available, may distinguish among these [5, 6].
Prognosis and Therapy
In general, the earlier the onset of symptoms, the greater the disability and the shorter the lifespan, which is negatively impacted mainly as a result of respiratory weakness. An antisense oligonucleotide drug, nusinersen, that modifies pre-messenger RNA splicing of the SMN2 gene and thus promotes increased production of full-length SMN protein has been approved for use in several countries [7]. Single-dose gene therapy for SMA, AAV9-SMN1 (AVXS-101), has even more recently received FDA approval [8].
References
Definition and Epidemiology
Charcot-Marie-Tooth disease (CMT), one of the most common inherited neurological disorders, is named after the three physicians who first identified it in 1886, i.e., Jean-Martin Charcot and Pierre Marie in Paris, France; and Howard Henry Tooth in Cambridge, United Kingdom. CMT, also known as hereditary motor and sensory neuropathy (HMSN) or peroneal muscular atrophy, comprises a group of genetically heterogeneous disorders characterized by abnormal myelination and/or axonal degeneration of peripheral nerves [1, 2].
CMT can be classified by the mode of inheritance (i.e., dominant or recessive), and by the primary localization of pathology (i.e., affecting neuronal soma, axons, or Schwann cells). The main categories comprise CMT 1–7 and an X-linked category. The disease usually clinically presents in the first or second decade of life with slowly progressing distal motoric weakness, sensory deficit, and foot deformities that may eventually result in a mild or moderate disability [1, 2].
This chapter mainly addresses forms of CMT that present in infancy and early childhood including Dejerine-Sottas disease (DSD) and congenital hypomyelinating neuropathy (CHN). Both of these early-onset disorders are classified as CMT 3 and are characterized by a more severe clinical course compared to the late-onset forms [1, 2].
CMT affects approximately 1 in 2,500 people in the United States, without any known ethnic or racial predispositions. CMT 1 and 2 account for the largest segment of CMT patients, with an incidence of 15 in 100,000 and 7 in 100,000, respectively. Individuals with X-linked forms account for approximately 10–20% of the entire CMT population. Other categories, including the early-onset CMT 3 forms, are far less common [3, 4].
Genetics
CMT is caused by mutations in one of several genes responsible for myelination and/or development of certain axonal structures within peripheral nerves. Inheritance is usually autosomal dominant, though autosomal recessive and X-linked variants are not uncommon [3, 4].
Over 80 genes have been linked to CMT; however, mutations in just four of them are responsible for the vast majority of cases: PMP22 (40.9–61.9%), GJB1 (10.7–10.8%), MPZ (3.1–6.7%), and MFN2 (2.8–7.0%). Mutations in GJB1, PMP22, MPZ, EGR2, LITAF, NEFL, or PMP2 are causing the absolute majority of autosomal dominant demyelinating CMT (Figure 51.1) [1, 4].
Clinical Features and Diagnosis
The clinical course and age of onset vary by the CMT subtype, and the disease may have strikingly different presentations, even among the affected family members. Apart from CMT 3 forms, in most cases first symptoms appear later in childhood, occasionally in adolescence, or even in adulthood. Typically, CMT begins with distal muscle weakness and atrophy manifesting with foot drop and pes cavus. As the disease advances, foot deformities (e.g., hammertoes) and weakness of hands may develop. Despite the fact that proprioception and vibration sensation are usually severely affected, many patients do not report sensory deficits. This is possibly due to the gradual deterioration of these sensations, where patients fail to notice the impairment. Nociception and thermoception are commonly intact, as pain and temperature signals are conveyed via unmyelinated nerve fibers. Sensory gait ataxia and positive Romberg test are common [2, 3, 4].
Dejerine-Sottas Disease (DSD)
Characteristic features of DSD include hypotonia, delayed motor development, distal weakness, sensory deficits, ataxia, and reduced or absent deep tendon reflexes that start manifesting in early infancy. Another typical deformity is early scoliosis, which may be accompanied by contractures. Nonetheless, the disease progression is typically slow and patients may preserve the ability to walk, sometimes even through adulthood [2, 3, 4].
Histopathology in patients with DSD typically shows prominent demyelination with thinning of the myelin sheath, as well as characteristic “onion bulbs” (Figure 51.1A, B); i.e., formations of circumferentially directed Schwann cells and their processes [6].
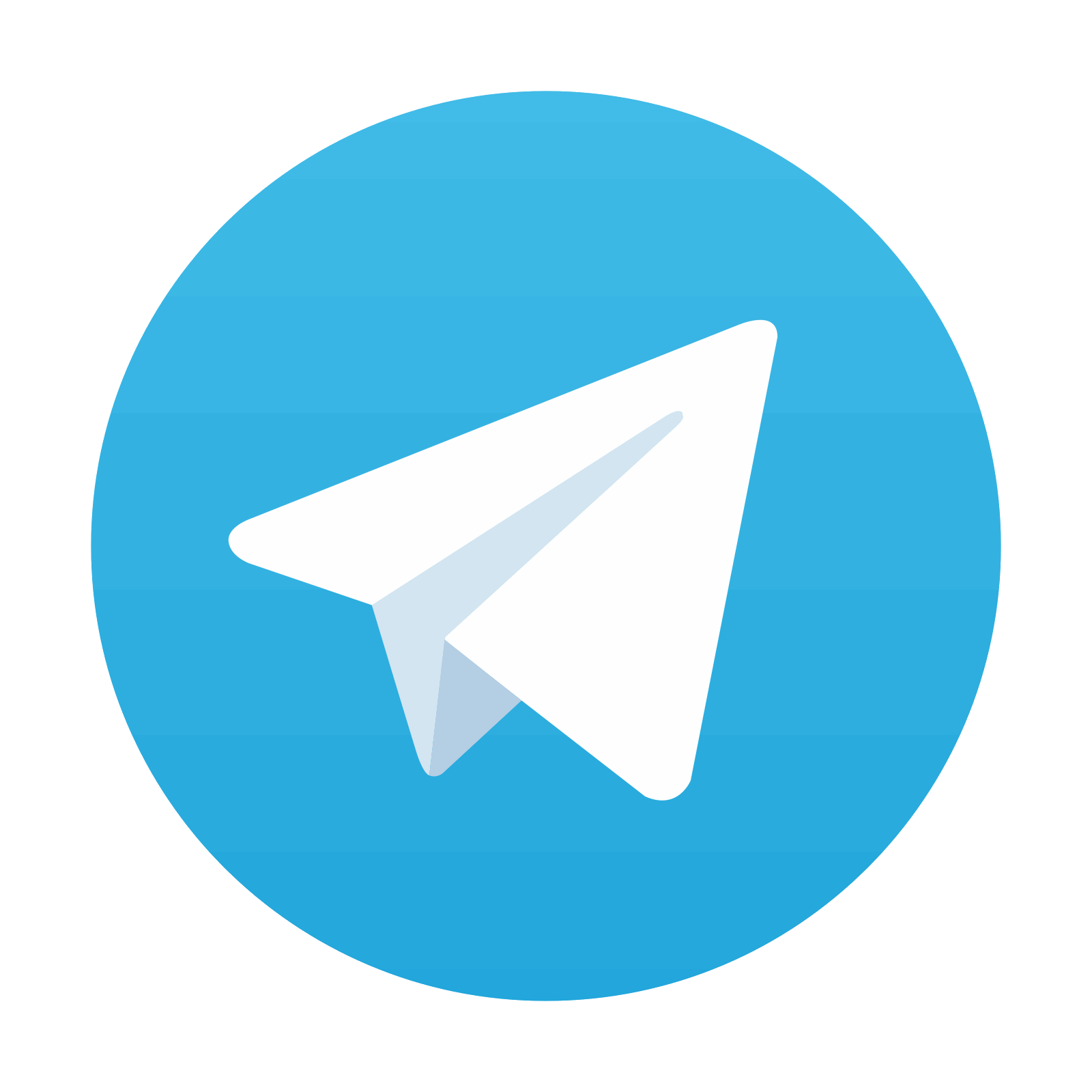
Stay updated, free articles. Join our Telegram channel

Full access? Get Clinical Tree
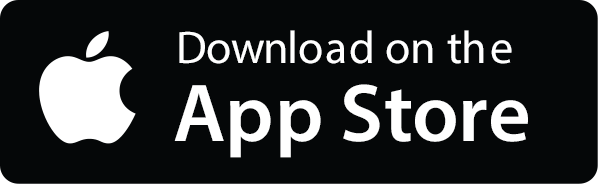
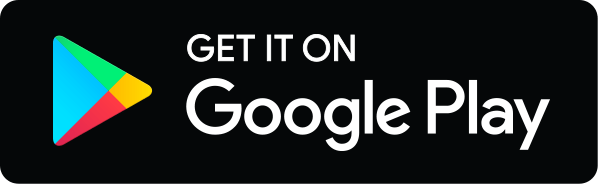