Introduction
Central nervous system (CNS) tumors are the second most common neoplasms in the pediatric population, being surpassed in incidence only by hematologic malignancies [1]. Congenital brain tumors are rare, representing 2% of all pediatric CNS tumors and having an incidence of approximately 1:1.1 to 1:3.4 million births, depending on the definition of “congenital” used by various reports: Jellinger and Sunder-Plassmann defined tumors as “definitely congenital” if they caused symptoms at birth or within the first two weeks of life, and “probably congenital” if they were diagnosed in the first year of life [2]; but, before them, Solitaire and Krigman classified congenital brain tumors in three categories: (1) “definitively congenital” if the tumor produced symptoms at birth, (2) “probably congenital “if the tumor produced symptoms within the first week of life, and (3) “possibly congenital” if the symptoms appeared within the first few months of life [3].
Due to their rarity, the vast literature on congenital brain tumors is represented by short series and case reports. It is apparent, though, that there is a male predominance (1.5:1 male to female ratio), independent of the type of tumor. The age at diagnosis tends to be in the second and third trimester of pregnancy, but it varies also by tumor type: while the mean age at diagnosis of congenital teratoma is 28 gestational weeks, astrocytomas are usually diagnosed around 36 gestational weeks [4].
Teratomas represent approximately 50% of all CNS tumors diagnosed before or shortly after birth. They are followed in incidence by astrocytomas, of which most are high-grade in this age group, but occasional cases of low-grade glial and glioneuronal tumors are seen [5]. Embryonal tumors and pineoblastoma, choroid plexus tumors, craniopharyngiomas, and lipomas can also be rarely encountered.
Risk Factors for Congenital Brain Tumors
The risk factors for congenital CNS tumors are not completely known. The potential associations of various factors like extreme birth weight (either large or small), maternal smoking, lack of folic acid and multivitamin supplementation, presence or absence of congenital malformations have been studied, but a clear association has not been discovered so far [6].
Some tumor predisposition syndromes are associated with CNS neoplasia, and the ones that have been reported to present with brain lesions in the prenatal period and in infancy are briefly discussed as follows.
Neurofibromatosis type I (NF1): NF1 is the most common form of neurofibromatosis, with an incidence of 1:3500 live births [7]. It can be inherited in an autosomal-dominant pattern, albeit approximately two-thirds of the cases are caused by spontaneous germ-line mutations in the NF1 gene at 17q11.2. In a review of 7 fetuses and 29 neonates with clinical features of NF1 reported by Isaacs [7], the majority of findings were detected during the third trimester of pregnancy or shortly after birth. The most common abnormalities observed in utero were intrauterine growth restriction, macrocephaly, congenital generalized neurofibromatosis [8], fetal hydrops, and thickened soft tissue in the neck. Subcutaneous neurofibromas, macrocephaly, café au lait spots, and buphthalmos were the relevant findings in neonates. Rarely, optic pathway gliomas are diagnosed in infancy, although the association with NF1 is not clearly defined in the literature [9].
Tuberous sclerosis: Another autosomal-dominant syndrome, tuberous sclerosis is caused by mutations in TSC1 at 9q34 and TSC2 at 16p13.3. It has an incidence of approximately 1:6000 individuals in the US, and a slight male predominance (1.2:1) [10]. Typically, the first signs of tuberous sclerosis appear on routine prenatal ultrasound, where heart and brain lesions can be present. In a review by Isaacs [10], more than half of the lesions found in fetuses and infants were represented by cerebral tubers, subependymal nodules, and subependymal giant cell astrocytoma (SEGA).
Li Fraumeni syndrome: An autosomal-dominant cancer predisposition syndrome affecting children and young adults, it is caused by germline alterations in the tumor suppressor gene TP53, and associated with a wide range of malignancies, including high-grade astrocytomas, ependymomas, and choroid plexus tumors [11]. Although the prenatal diagnosis of CNS tumors associated with Li Fraumeni syndrome are not reported in the literature, the presence of a TP53 mutation at a high allele frequency and/or diffuse nuclear expression of p53 antibody in a congenital tumor should raise suspicion for a germline mutation, and prompt genetic testing.
Rhabdoid tumor predisposition syndrome: This is caused by germ-line alterations in SMARCB1 (rhabdoid tumor predisposition syndrome type I) or SMARCA4 (rhabdoid tumor predisposition syndrome type II), and the mode of inheritance is not elucidated [12]. A family history of rhabdoid tumors or the presence of a rhabdoid tumor or skin neurovascular hamartoma [13] in an infant, are possible clues to the tumor predisposition syndrome, and germ-line testing should be pursued. The case of an infant who presented with a neurovascular hamartoma that led to imaging and the discovery of a possibly congenital atypical teratoid rhabdoid tumor (ATRT) and germline confirmation of the syndrome is illustrated in Figure 48.1.
Figure 48.1 A young infant born with a cutaneous red-pink lesion (Images from the archives of the Department of Pathology at Boston Children’s Hospital). A) Hematoxylin-eosin (H&E) section showing a vascular and spindle cell lesion in the superficial dermis (inset demonstrating higher magnification). INI1 expression is lost in the lesional nuclei B). The histologic features are in keeping with a neurovascular hamartoma. A third ventricular tumor was identified on MRI. Histology showed a densely cellular primitive neoplasm with occasional rhabdoid cells C), and INI1 expression was lost D).
Von Hippel-Lindau disease: This disorder has an autosomal-dominant pattern of inheritance and is caused by germline mutations of the VHL gene at 3p25-26. Approximately 30% of hemangioblastomas are associated with Von Hippel-Lindau. Hemangioblastomas are rare tumors of the CNS and retina, and only rare case reports have been described in infants, none of them associated with the syndrome [14; 15].
Gorlin syndrome (Nevoid basal cell carcinoma syndrome): This is caused by autosomal-dominant inheritance of genes encoding members of the Sonic Hedgehog Signaling (SHH) pathway. Gorlin syndrome is associated with developmental disorders, basal cell carcinomas, odontogenic keratocysts, intracranial calcifications, macrocephaly, as well as desmoplastic nodular medulloblastoma, SHH subtype. Medulloblastomas associated with Gorlin syndrome usually have intact TP53 and a good prognosis [16]. Congenital medulloblastomas are extremely rare; one desmoplastic nodular medulloblastoma was recently reported by Korostyshevskaya et al. in a 31 weeks gestation fetus. The histology was desmoplastic nodular, and RNA expression studies were in keeping with SHH pathway activation, albeit an investigation for germ-line mutations was not reported [17].
DICER1 syndrome: This is a rare, relatively newly identified tumor predisposition syndrome with an autosomal-dominant pattern of inheritance. The pathognomonic tumor for DICER1 syndrome is pleuropulmonary blastoma. Other manifestations described include thyroid benign and malignant proliferations, embryonal rhabdomyosarcoma, renal anaplastic sarcoma, Sertoli-Leydig tumor of the ovary, nasal chondromesenchymal hamartoma [18]. Recently, Uro-Coste and colleagues [19] described two cases of DICER1-related cerebellar embryonal tumors in 8 and 11 months old infants. The tumors resembled embryonal tumor with multilayered rosettes, with some areas reminiscent of medulloepithelioma. Both tumors demonstrated heterologous elements in the form of cartilage and skeletal muscle. LIN28A is an immunosurrogate for C19MC amplification, albeit not specific; it was positive in the two cases in this study, however, no amplification was present at chromosome 19q13.41 miRNA cluster, which argued against classic embryonal tumors with multilayered rosettes. Germline truncating DICER1 mutations were discovered in both children. The author of this chapter (SA) encountered in practice a similar case (illustrated in Figure 48.2) in a two month-old child. Given the young age, it is possible that all these three tumors developed in utero.
Figure 48.2 A young infant found to have a large posterior fossa and intraventricular mass (Images from the archives in the Department of Pathology at Boston Children’s Hospital) A). H&E sections showed a densely cellular embryonal neoplasm with abundant neuropil and some strands of skeletal muscle B). The tumor was diffusely positive for synaptophysin, in keeping with a primitive neuronal neoplasm C). Areas with skeletal muscle D) were highlighted by myogenin immunostain E). The tumor was positive for LIN28A F), however, there was no corresponding C19MC amplification on array CGH; next-generation sequencing was remarkable for a truncating and a missense mutation in DICER1 (not shown).
Pituitary blastoma is a rare DICER1-associated tumor that is usually diagnosed in early infancy, as a large pituitary mass that secretes ACTH and GH and causes Cushing-like manifestations [18]. Other recently described DICER1 CNS manifestations are pineoblastomas and primary CNS DICER1-associated sarcoma, albeit congenital cases have not been described.
Rare tumor predisposition syndromes with CNS manifestations: Other tumor predisposition syndromes with the risk of CNS tumors are Turcot (high-grade gliomas), Cowden (dysplastic cerebellar gangliocytoma), and various RAS-opathies (Noonan in particular, associated with dysembryoplastic neuroepithelial tumors) [20].
Clinical Presentation of Congenital Brain Tumors
Due to advances in prenatal imaging, many congenital brain tumors are diagnosed in utero on routine ultrasound, most frequently during the second and third trimesters of pregnancy. Signs that might indicate a brain tumor during fetal life are macrocephaly and hydrocephalus, often requiring cesarean delivery. Cases of polyhydramnios secondary to depressed swallowing from hypothalamic dysfunction have also been described. Congenital brain tumors tend to be large at presentation; hence the clinical presentation after birth usually includes macrocephaly, bulging fontanelle, and signs of increased intracranial pressure. Depending on anatomic location, other symptoms encountered are seizures, cranial nerve palsies, and increased fussiness, difficulty with feeding, and vomiting. Tumors in the hypothalamic/suprasellar region present with endocrinopathies and visual disturbances [21, 22]. According to Volpe [23], the presentation of congenital brain tumors can be grouped into one of the following four categories:
1. Gigantic tumors causing severe macrocrania that can lead to cephalopelvic disproportion, dystocia, stillbirth, premature labor
2. Infants presenting with a large head and bulging fontanelle due to hydrocephalus
3. Specific neurologic findings related to the type and site of the lesions
4. Sudden onset of intracranial hemorrhage
Imaging
Congenital brain tumors differ from those occurring in older children in terms of location and size. Because the sutures of fetal and neonatal calvarium are not fused, the brain tumors can reach large dimensions before they are diagnosed. Also, most congenital tumors are supratentorial, in comparison to an infratentorial location in older children. Imaging modalities that are useful in diagnosis are ultrasound, computed tomography (CT), and magnetic resonance imaging (MRI) [24]. The appearance of the most common congenital brain tumors is discussed below:
Teratoma: Teratoma is the most common congenital brain tumor, representing approximately 50% of all congenital brain tumors. The ultrasound or MRI may show a large cystic and solid mass that occupies almost the entire brain and extends to the orbits or bone structures; smaller tumors may cause hydrocephalus. They can arise from the pineal gland or suprasellar region, as well as from cerebral hemispheres, although the anatomic landmarks are difficult to establish when the tumors are massive. CT and MRI will demonstrate lesions with mixed density, adipose tissue, and calcifications. Contrast enhancement is usually heterogeneous, highlighting the solid and cystic components. Immature and malignant components have indistinct borders. Surrounding edema is usually present (Figure 48.3A).
Astrocytoma and glioneuronal tumors: Congenital astrocytomas and glioneuronal tumors are usually supratentorial, and high-grade histology is common. The tumor is often detected at prenatal ultrasound as an echogenic large mass that occupies large parts of a hemisphere and produces midline shift and obstructive hydrocephalus. On MRI, high-grade astrocytomas are isointense in T1- and T2-weighted images (Figure 48.3B). Restricted diffusion is present in tumors with increased cellularity, and is indicative of high-grade lesions. Intratumoral hemorrhage is not uncommon, and follow-up imaging might be necessary to distinguish a benign intracerebral hemorrhage from a neoplastic process. A particular entity that deserves special mentioning in this category is desmoplastic infantile astrocytoma/ganglioglioma (DIA/DIG), which is primarily seen in infants and young children and is exclusively supratentorial. There is characteristic involvement of large areas of cerebral hemispheres, mimicking a malignant process. On imaging, DIGs and DIAs are large, hypodense, cystic, and solid tumors, with contrast enhancement in the solid component (Figure 48.3C). The solid component is T2-hypointense, which is useful for differentiating this tumor from other astrocytomas.
Ependymoma: Although ependymoma is one of the most common tumors of childhood, it is rarely diagnosed in prenatal life. It usually arises from the fourth ventricle, with only a few cases arising in the supratentorial compartment. Imaging demonstrates a circumscribed tumor with various degrees of contrast enhancement (Figure 48.3D). Intratumoral hemorrhage, calcifications, and occasionally cysts are seen.
Embryonal tumors: The imaging characteristics of embryonal tumors are dependent on the specific diagnosis and anatomic location; cerebral embryonal tumors tend to be large bulky masses with areas of necrosis and hemorrhage. They occupy large areas of the cerebral hemispheres. MRI will show large, heterogeneous masses that are hypointense on T2-weighted images and demonstrate restricted diffusion. Medulloblastomas present as posterior fossa masses that cause hydrocephalus. The nodules of desmoplastic nodular medulloblastomas are sometimes apparent on MRI images. Pineoblastomas will have similar imaging characteristics and the location will predict the histologic diagnosis. A significant number of embryonal tumors will present with leptomeningeal dissemination and metastasis; therefore, contrast-enhanced MRI of the entire neuraxis should be included in the initial assessment (Figures 48.3E and 48.3F).
Choroid plexus tumors: Choroid plexus tumors (CPT) have an overall incidence of 3% in the pediatric group, but they represent up to 15% of the tumors that occur during the first year of life. In infancy, the most common location is lateral ventricles. Imaging of CPTs usually demonstrates a large intraventricular mass with a papillary pattern that is T1-hypointense and T2-hyperintense, and avid contrast enhancement due to robust intratumoral vascularity.
Figure 48.3 Various appearances of congenital and infantile brain tumors on MRI (Images from the archives at Boston Children’s Hospital). A) Newborn with a large left cerebellar hemisphere immature teratoma; axial T1 post-Gadolinium sequence showing heterogeneous signal intensity, areas of calcification, blood products, and cysts. B) Axial T2 Flair image showing a large bifrontal and intraventricular heterogeneous high-grade glioma causing hydrocephalus, peritumoral and periventricular edema and midline shift. C) Axial T1 post-Gadolinium image showing a solid and cystic large desmoplastic infantile astrocytoma occupying almost the entire right hemisphere of a young infant. D) Saggital T1 Flair image showing an intraventricular ependymoma. E) Axial T1 post-contrast: ATRT centered deep in the left insula. F) Atypical choroid plexus papilloma in a 6 month-old. Axial T1 post-contrast image showing a large, well-defined, vascular, intraventricular tumor.
Craniopharyngiomas: Craniopharyngiomas diagnosed in utero and in infancy are very rare. They present on imaging studies as well-defined midline suprasellar lesions with mixed hypo-and hyperintense signal and calcifications. They may be locally destructive, disrupting the pituitary gland, optic pathway, and hypothalamus.
Other tumors and mimickers of tumors: Lipomas represent approximately 10% of prenatal tumors [25, 26]. Lipomas are usually in midline locations and associated with agenesis of the corpus callosum or Chiari malformation. MRI is particularly useful in establishing the presence of adipose tissue and possible associated brain malformations.
A perpetual differential diagnosis of brain tumors is intracranial hemorrhage and infarction, which can present on imaging studies as a disorganized, heterogeneous mass with ill-defined borders and hydrocephalus. Because prenatal tumors have a propensity to bleed, an underlying tumor should always be considered when evaluating an intracranial hemorrhage.
Congenital vascular anomalies can present in the neonatal period with neurological symptoms due to an acute intralesional bleed. As with intracranial hemorrhages, the distinction from a brain neoplasm can be challenging.
Hamartia and hamartomas are collections of disorganized neurons in abnormal locations and may cause seizures. MRI studies demonstrating signal features similar to normal gray matter and lack of mass effect are usually reassuring in these situations.
Pathologic Diagnosis
A specific pathologic diagnosis of prenatal brain tumors and all CNS tumors in general will integrate the radiologic findings, gross and microscopic appearance, and molecular test results, as suggested in recent practice guidelines published by the International Society of Neuropathology [27].
On gross examination, the tumors are usually large, involve multiple structures, and produce distortion of anatomic landmarks and hydrocephalus (Figure 48.4). Teratomas contain cysts, calcification, parts of teeth, and fat, whereas germ cell tumors are usually solid and soft. Choroid plexus tumors will present as intraventricular, cauliflower-like masses with a rich vascular network. Astrocytomas are ill-defined and may contain solid and cystic areas, as well as areas of hemorrhage and necrosis. Embryonal tumors are soft and solid, infiltrative, with areas of necrosis and possibly hemorrhage. Leptomeningeal involvement and spread to the cerebrospinal fluid can be seen in all malignant prenatal brain tumors.
Microscopic histologic and immunohistochemical features, as well as genetic findings, are discussed as follows for each tumor type.
Teratoma: Congenital germ cell tumors are typically teratomas, which are composed of somatic tissues derived from two or three of the germ layers. Teratomas are further stratified as:
– Mature, composed of adult-type tissues (most commonly skin and skin appendages, eccrine glands, smooth and skeletal muscle, bone, cartilage, respiratory and gastrointestinal epithelium, neural tissue).
– Immature, which contain embryonic or fetal tissue, either by itself, or in combination with mature elements.
– Malignant, in which a component of the teratoma undergoes malignant transformation; the most common situation is the carcinomatous transformation of an epithelial component, however, sarcomas and embryonal tumors are also possible [28].
Congenital teratomas are usually immature. They are composed of mitotically active stroma resembling embryonic mesenchyme and immature neuroectodermal elements forming multilayered true rosettes with a central lumen. Congenital teratomas may contain melanotic neuroepithelium derived from the abortive retinal pigment epithelium. The presence of an even minor immature component in a mature teratoma warrants a diagnosis of immature teratoma (Figure 48.5A-D).
Figure 48.5 Mixed germ cell tumor with teratomatous elements (Images from the archives of the Department of Pathology, Boston Children’s Hospital): A) glandular structures lined by columnar epithelium next to immature cartilage, in a mesenchymal background stroma; B) rhabdomyoblasts and more mature skeletal muscle fibers; C) immature neuronal component in the form of multilayered rosettes, next to intestinal-type yolk sac tumor. Some areas of immature neuronal components resembled primitive neuroectodermal tumor, with more sheet-like growth and increased mitoses D).
Teratomas with malignant transformation of a tissue type have been described [29, 30], with rhabdomyosarcoma, undifferentiated sarcoma, and carcinomatous transformation being the most common presentations.
Teratomas containing abundant glioneuronal tissue can sometimes be difficult to diagnose on biopsies, and may be confused with a glioneuronal tumor if only the brain tissue component is sampled. When resection is not possible, sampling of many regions of the tumor and correlation with imaging studies is necessary in order to avoid misdiagnosis. Figure 48.6 demonstrates such a case encountered in practice by the author of this chapter (SA): a 3-month-old child was found to have a third ventricular solid tumor with some possible cystic spaces identified on MRI. Initial biopsy demonstrated only glioneuronal tissue with mild atypia and disorganization, and was interpreted as “glioneuronal tumor.” A follow-up partial resection contained gland-like structures, smooth and skeletal muscle, focal immature neuronal tissue, and pigmented epithelium, in keeping with an immature teratoma.
Figure 48.6 Three-month-old child with a third ventricular solid tumor (Images from the archives of the Department of Pathology, Boston Children’s Hospital). The tumor is mostly homogeneous, with a small cystic area and focal contrast enhancement (A, axial T2 post contrast). A first small biopsy demonstrated only glioneuronal tissue with mild atypia, favored initially to represent a glioneuronal neoplasm (B, H&E). More tissue was obtained at reoperation, and histologic examination showed glandular structures admixed with smooth muscle and glioneuronal tissue (C), as well as rare foci with primitive neuronal elements (D), in keeping with an immature teratoma.
Immunohistochemical stains meant to highlight different teratomatous tissues and to exclude other germinomatous components are frequently used in practice (e.g., keratins for epithelium, as well as embryonal carcinoma, myogenin for skeletal muscle, GFAP and synaptophysin for glial and neuronal tissue; CD117, Oct3/4, LIN28A and D2-40 will be positive in germinoma, but negative in yolk sac tumors, which express glypican 3 and AFP; beta-HCG will highlight choriocarcinomatous elements and CD30 will be immunoreactive in embryonal carcinoma). Serum levels of alpha feto-protein and beta-hCG are also helpful in supporting or ruling out a mixed germ cell tumor and in guiding clinical management.
The genetic profile of congenital and perinatal pure teratomas lacks meaningful mutations; they are chromosomally balanced diploid tumors, resembling infantile testicular teratomas.
Astrocytoma: High-grade gliomas, and glioblastoma WHO grade 4 in particular, are the leading form of congenital astrocytomas. Histologically, congenital high-grade gliomas are indistinguishable from the ones occurring later in childhood and in adults. They are infiltrative and demonstrate increased cellularity and atypia, frequent mitoses, microvascular proliferation, and necrosis. Largely, the tumors are diffusely positive for glial markers (GFAP and OLIG2) and negative for synaptophysin expression. Genetically, they are quite distinct from high-grade glioma in childhood and adulthood: while they can harbor TP53 alterations and loss of PTEN, EGFR amplification, and IDH mutations are not described [31]. Histone mutations are also infrequent in congenital glioblastoma. Recent publications, conference papers, and clinical observations suggest that activation of MET-ALK pathway through ALK and ROS1 fusions (and potentially through other gene alterations) are responsible for a subset of congenital and infantile high-grade gliomas [32]. ALK immunohistochemical stain can be used in practice to screen for ALK genetic alterations. This discovery is particularly important for therapeutic management, as ALK inhibitors are already used in some adult carcinomas and hematologic malignancies, and experimental studies are in incipient phases in glioblastoma cell lines [33].
Low-grade astrocytomas are very rare in prenatal life and infancy. Upon a literature review, subependymal giant cell astrocytomas (SEGA), pilocytic astrocytomas, and desmoplastic infantile astrocytomas/gangliogliomas seem to rarely arise prenatally [4, 34].
– SEGAs occur in the subventricular areas, over the basal ganglia, as nodular, well-delineated proliferations of large cells with eosinophilic cytoplasm that resemble gemistocytes. The cells are arranged in sweeping fascicles and sheets with occasional perivascular palisading. Smaller, spindle cells or pyramidal morphology resembling ganglion cells can also be present. The stroma is vascular, and perivascular aggregates of lymphocytes and mast cells are frequently encountered. Although sometimes SEGAs can demonstrate increased mitotic activity, microvascular proliferation, and necrosis, these features are not indicative of a more aggressive behavior. Perivascular and parenchymal calcifications are frequent. SEGAs are extensively immunopositive for both glial and neuronal markers. As mentioned in the discussion of risk factors for congenital tumors, SEGAs are closely associated with tuberous sclerosis syndrome, and TSC1 or TSC2 mutations that result in the activation of the mTOR pathway. A recent study by Kuki and colleagues demonstrated the efficacy of mTOR inhibitor everolimus in children with congenital and infantile SEGAs [34]. In that study, all five patients included experienced shrinkage of the tumors, with the most pronounced change in size occurring in the first three months of treatment. A small subset of SEGAs harbor BRAF V600E mutation independent of the status of TSC1 and TSC2, offering another therapeutic possibility [35].
– Pilocytic astrocytomas can arise anywhere in the neuraxis. The spinal cord, cerebral hemispheres, and optic tracts are common locations in the prenatal period, with the latter being associated with NF1 syndrome and portending a worse prognosis. Histologically, pilocytic astrocytomas are biphasic tumors with areas that are more hypercellular and have a dense fibrillary background alternating with areas with myxoid stroma and cystic changes. The cells are mildly atypical and have round-to-oval nuclei and bipolar processes. Occasional mitoses, linear microvascular proliferation, and infarct-type necrosis are not uncommon, and are not associated with aggressive behavior. The tumor is mostly compact with infiltration in the adjacent tissue at the edge. Rosenthal fibers and eosinophilic granular bodies are present. Pilocytic astrocytomas are positive for glial markers and usually negative for neuronal markers. A neurofilament protein immunostain can be helpful in highlighting areas of minimal infiltration, where it is positive in entrapped axons. Optic pathway pilocytic astrocytomas are usually associated with NF1 germline mutations and lack BRAF alterations, which are the most common alteration of pilocytic astrocytomas in other CNS locations. The vast majority of pilocytic astrocytomas carry a KIAA1549-BRAF fusion, albeit BRAF mutations, fusions with other genes, intragenic deletions, and alterations in other genes in the MAP kinase pathway has been described in a recent genomic analysis of 203 pediatric brain tumors [36].
– Desmoplastic infantile astrocytoma/ganglioglioma (DIA/DIG) is usually diagnosed in the first year of life as a large cystic and solid hemispheric tumor, occasionally with intratumoral hemorrhage. They are composed of astrocytes with oval and spindled nuclei with a desmoplastic and collagenous background stroma. Despite the large size, marked atypia, and frequent mitoses, their behavior is indolent. Reticulin stains thick fibers surrounding nests of tumor cells that are positive for GFAP. Ganglion cells are seen in DIG. A recent multi-institutional study by Wang and colleagues demonstrated frequent BRAF V600E and BRAF V600D somatic mutations in a cohort of twelve DIA/DIG. In this study, three of the tumors recurred and they were found to have genetic features indicative of an aggressive behavior: one had a TP53 mutation, another one had an ATRX deletion, and the third had an EML4-ALK fusion. Methylation studies and clustering analysis revealed that DIA/DIG cluster separately from all other pediatric gliomas, independent of their BRAF status, reinforcing that they are a distinct histologic and molecular entity [37].
Ependymoma: Congenital ependymomas are rare, with less than ten cases reported in the literature [38]. They are well-defined usually solid tumors composed of mildly atypical cells with oval nuclei, speckled chromatin, and glial processes. The tumor cells are arranged in sheets and perivascular pseudorosettes. Criteria for anaplasia is not well defined, however, in general, it is accepted that a high mitotic index, palisading necrosis, and microvascular proliferation are indicative of anaplasia. The tumor cells are extensively positive for GFAP and S100, and EMA is usually positive in a dot-like pattern. In an international multi-institutional collaboration, Pajtler and colleagues subclassified ependymomas in nine subgroups, three in each of the CNS compartments: spinal cord, posterior fossa, and supratentorial. In the spinal cord, subependymoma, myxopapillary ependymoma, and ependymoma each have their own molecular features, and they differ from the posterior fossa ependymomas, which can be subdivided into subependymoma, group A (usually infants, chromosomally balanced and poor prognosis) and group B (older children, chromosomally imbalanced and better prognosis than group A); in the supratentorium, the discovery of RELA fusions and their correlation with poor prognosis, led to a specific ependymoma diagnosis in WHO Classification of CNS tumors: “RELA-fusion ependymoma.” In practice, L1CAM immunostain can be used as a surrogate for molecular alteration. Other supratentorial ependymomas have YAP1 fusions and are thought to have a better prognosis [39]. Figure 48.7 illustrates representative microscopic features of these neoplasms.
Figure 48.7 A) Congenital glioblastoma with an ALK rearrangement (Images from the archives in the Department of Pathology, Boston Children’s Hospital); ALK immunostain was positive (B). C) Pilocytic astrocytoma is composed of astrocytes with oval, mildly atypical nuclei and prominent glial processes. Rare Rosenthal fibers are present. D) Subependymal giant cell astrocytoma (SEGA) composed of cells with abundant eosinophilic cytoplasm and occasional prominent nucleoli; calcification is present. E) Desmoplastic infantile astrocytoma is composed of spindle glial cells arranged in disorganized fascicles. Mitoses and moderate nuclear atypia are common. F) Ependymoma composed of cells with oval-to-round nuclei and short processes, arranged in perivascular pseudorosettes. True ependymal rosettes are also seen in the image.
Congenital embryonal tumors: In 2016, an international collaborative study by Sturm and colleagues [40] demonstrated that institutionally diagnosed CNS primitive neuroectodermal tumors (CNS-PNET) are a molecular heterogeneous group of tumors, and that the majority of them can be reclassified in other well-characterized CNS entities after transcriptomic and methylation analysis. Some of the CNS-PNETs could be classified as medulloblastomas, embryonal tumors with multilayered rosettes (ETMR), atypical teratoid rhabdoid tumors (ATRT), and neuroblastoma, whereas others clustered with high-grade gliomas and ependymomas, meningiomas, or sarcomas. Four new entities emerged from this study: high-grade neuroepithelial tumor (HGNET) with BCOR internal tandem duplication (HGNET-BCOR ITD), HGNET with MN1 alterations, CNS EFT-CIC, and neuroblastoma with FOXR2 alterations.
The literature refers to many of the congenital embryonal tumors as “congenital PNET.” This section describes embryonal tumors as listed in the 2016 WHO Classification of Central Nervous System Tumours, in prenatal life and infancy [41].
While medulloblastoma is the most common embryonal tumor later in childhood, it is very rare for it to be diagnosed prenatally. ETMR and ATRTs are more frequent in prenatal life [42, 43]. Pineoblastomas have been infrequently described, too. Although some histologic features are helpful in the diagnosis of particular embryonal tumor-types (e.g., presence of multilayered rosettes in ETMR and rhabdoid cells with eccentric nuclei with pronounced nucleoli in ATRT), there is substantial overlap in the findings seen on hematoxylin-eosin-stained sections; for example, ATRTs can demonstrate extensive primitive neuronal components, which makes it difficult to distinguish them from a medulloblastoma, pineoblastoma, or ETMR. Correlation with imaging studies, anatomic location, immunohistochemical and molecular studies is essential for a specific diagnosis. Below is a discussion on the pathologic diagnosis of the most common congenital embryonal tumors:
– ETMR can arise anywhere in the neuraxis; they are infiltrative, large, aggressive tumors composed of primitive small blue cells in a background of neuropil; there are areas of increased cellular density with multilayered rosettes, admixed with areas of abundant neuropil (Figure 48.8A). Necrosis, apoptotic figures, and mitoses are frequent. A large proportion of ETMRs have fusions or amplifications of C19MC locus at 19q13.42, and they comprise most embryonal tumors previously classified as an embryonal tumor with abundant neuropil and true rosettes (ETANTR), ependymoblastoma, and medulloepithelioma. Each of these patterns is based on its histologic appearance: ETANTR show a biphasic architecture with dense clusters of small cells with high nuclear-to-cytoplasmic ratio arranged in multilayered rosettes with a central lumen, admixed with large, paucicellular areas of neuropil with occasional neurons and ganglion cells; ependymoblastoma is composed of clusters of poorly differentiated cells forming numerous multilayered rosettes and pseudorosettes, but lacking the large areas of neuropil; medulloepithelioma is composed of trabeculi and papillae of multilayered primitive cells with an external limiting membrane that is periodic acid-Schiff-positive. Away from the papillary structures, there are sheets of primitive cells that may form multilayered rosettes. Mesenchymal differentiation or melanin pigment can be present.
ETMRs express synaptophysin, nestin, and vimentin and are negative for glial markers. LIN28A, which is a protein that binds small RNAs, is a useful immunosurrogate for C19MC amplification and fusion, albeit non-specific (Figure 48.8B).
The genetic profile of ETMRs includes gains of chromosomes 2, 7q, 11q, and loss of 6q, as well as the characteristic amplicon at 19q13.42, found in the majority of ETMRs. This genetic alteration has not been identified in other brain tumors. Complex rearrangements at the same chromosomal locus, as well as C19MC-TTYH1, have also been seldom described.
– ATRT is rarely diagnosed in the prenatal period. It can arise anywhere in the neuraxis, and usually has leptomeningeal dissemination at presentation. The macroscopic aspect is similar to that of any other CNS embryonal tumor: ATRTs tend to be soft, pink-tan, and large, with areas of necrosis and hemorrhage. Microscopically, an ATRT is composed of cells with eccentrically placed nuclei with vesicular chromatin and prominent nucleoli. The cytoplasm is abundant and contains paranuclear intermediate filaments. Rhabdoid cells are arranged in sheets and nests, and are discohesive (Figure 48.1). Most tumors will contain primitive neuroectodermal, mesenchymal, or epithelial features – hence the focal immunopositivity with SMA, GFAP, synaptophysin, and EMA. The diagnosis of ATRT requires inactivation of SMARCB1at 22q11.2 or, rarely, SMARCA4, either through biallelic deletion, mutation, or a combination of deletion and mutation. This leads to loss of INI1 or BRG1 expression in the tissue, which is helpful in distinguishing ATRT from other embryonal tumors. The association of ATRT with rhabdoid tumor predisposition syndrome was discussed previously in the “Risk Factors” section of this chapter. Because the risk of a germline alteration is approximately 33%, genetic testing is advised for all newly diagnosed cases.
– Medulloblastoma is very rarely congenital, with only sparse case reports found in the literature. The cerebellar location, retained INI1, and molecular testing results consistent with one of the four molecular subgroups (Wnt, SHH, Group 3 and 4) are helpful in establishing a diagnosis [44]. Congenital and infantile medulloblastomas tend to be of desmoplastic-nodular or extensive nodularity subtype (Figure 48.8C) with SHH-pathway activation, and sometimes they are associated with Gorlin syndrome.
Pineoblastoma is a primitive tumor of the pineal gland that can be differentiated from other embryonal tumors based on anatomic location, immunoexpression of the retinal marker CRX [45] (Figure 48.8D and 48.8E), and molecular test results. Pineoblastomas can be encountered in Rb and DICER1 tumor predisposition syndromes, as well as sporadic. While in most DICER1-associated tumors DICER1 biallelic inactivation occurs through mutations – one truncating and one missense – in pineoblastoma there is a complete loss of DICER1 function through a loss of heterozygosity [46]. A similar effect on the microRNA’s pathway in pineoblastomas occurs through DROSHA homozygous deletions, which interferes with the microRNA activity upstream of DICER1 [47]. Other alterations in pineoblastomas come from a recent study that uncovered somatic mutations in ARID1A and KDM5 C, but their biological implications are not clearly elucidated [48].
Figure 48.8 A) Embryonal tumor with multilayered rosettes (ETMR) – a densely cellular primitive neoplasm with areas in which the cells tend to aggregate and occasional multilayered rosettes, alternating with neuropil-reach areas. B) LIN28A immunostain is extensively positive. C) Desmoplastic nodular medulloblastoma with nodules composed of more mature cells and generous neuropil, surrounded by areas of dense primitive cells. D) Pineoblastoma – densely cellular primitive neoplasm with a background of neuropil. E) The cells of pineoblastoma are immunopositive for CRX.
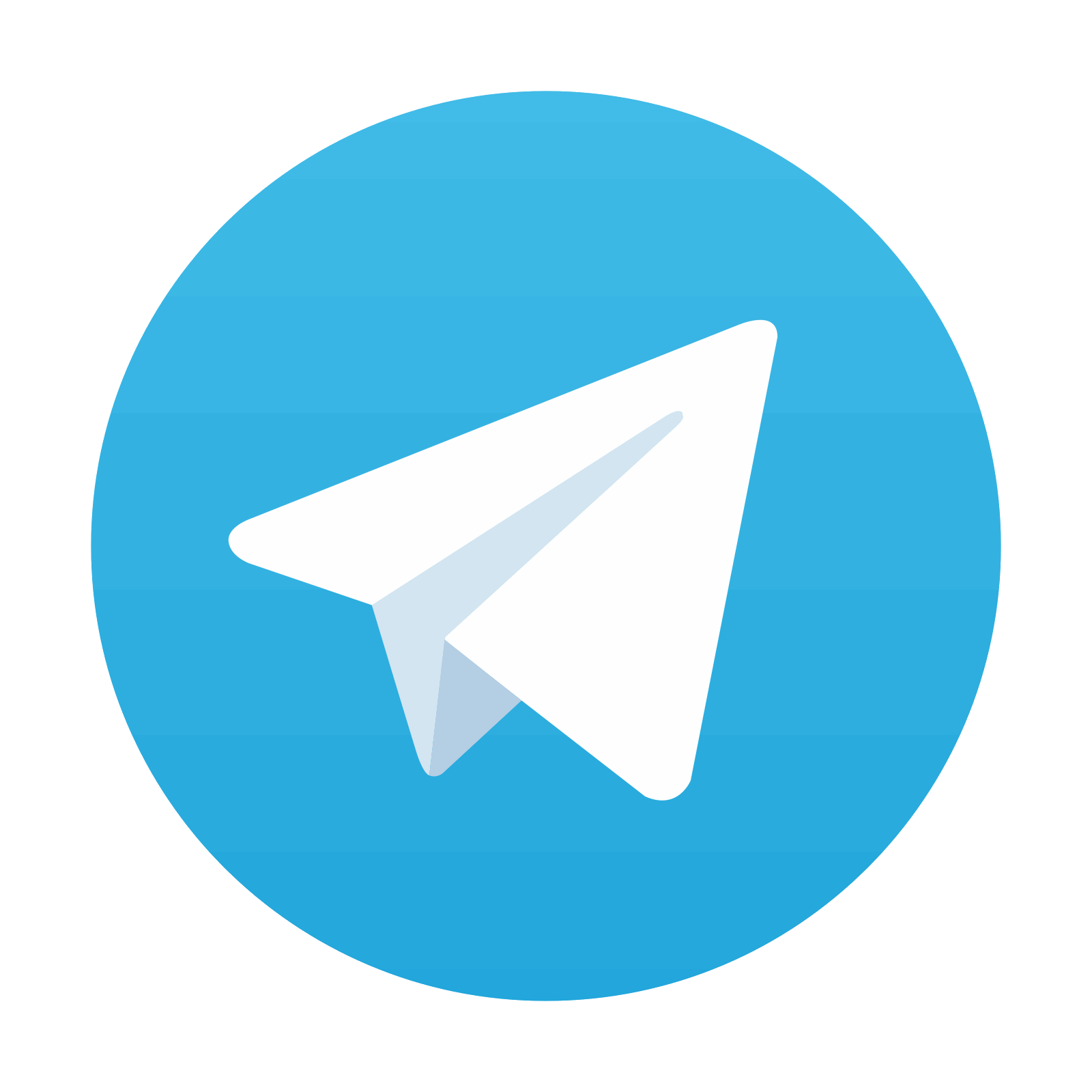
Stay updated, free articles. Join our Telegram channel

Full access? Get Clinical Tree
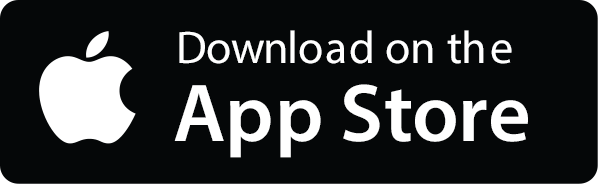
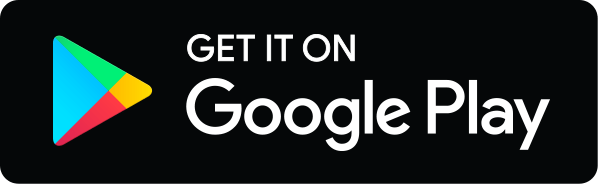
