Sperm nuclear and chromatin abnormalities are common among infertile men and are known to influence natural reproduction. These abnormalities are also considered detrimental to normal fertilization, embryo development, and successful implantation and pregnancies following assisted reproductive treatment (ART). Abnormalities in the sperm nucleus can be broadly classified into sperm chromosomal abnormalities (aneuploidies) and sperm DNA abnormalities such as abnormal packing, DNA integrity, or DNA fragmentation. For the past 30 years, numerous tests have been developed to quantify these abnormalities in sperm. In this chapter, we review the causes of sperm DNA and chromosomal abnormalities, describe the commonly used tests to evaluate these abnormalities, and finally review the impact of these abnormalities on male fertility and ART outcomes. We also performed a comprehensive meta-analysis and systematic review from the existing literature to summarize the effect of sperm DNA fragmentation on ART outcomes such as fertilization rate, embryo quality, and clinical pregnancies. A review of the literature presented in this chapter suggests that sperm nuclear and chromatin abnormalities are associated with male infertility, and they reduce the probability of a successful pregnancy following ART.
Highlights
- •
Sperm nuclear and chromatin abnormalities are common among infertile men and are known to influence natural reproduction.
- •
The haploid paternal genome provides the genetic material to essential to initiate normal embryonic development.
- •
Chromosomal abnormalities are seen in <1% of normospermia, high in 10–15% of azoospermia and 5% of oligozoospermia cases.
- •
The evidence presented here favors the use of sperm DNA assessment as clinically useful biomarkers.
Introduction
Infertility is a common problem worldwide and is known to affect both male and female partners. Approximately 15% of the couples are affected by infertility globally, among whom male infertility accounts for 50% of the reduced fertility . Semen analysis has been the gold standard test for male infertility evaluation, and in most patients diagnosed with known male factor infertility, one or more abnormal sperm parameters are observed. While sperm abnormalities are found in infertile men, recent research has shown that other male factors may play a role even when the semen analysis is normal . It is well established that sperm is a vehicle that aids the transport of the haploid paternal genome to the oocyte and that the delivery of intact and complete genetic material is required for normal embryonic development .
Chromosomal abnormalities in sperm are believed to be one of the major causes of male infertility and are associated with abnormal spermatogenesis. The occurrence of chromosomal abnormalities are high in 10–15% of men with azoospermia, in approximately 5% of men with oligozoospermia, and in less than 1% of men with normal semen analysis parameters . Other genetic abnormalities, such as Y-chromosome microdeletion, are also common in men with severely impaired spermatogenesis . Unlike in chromosomal abnormalities, sperm DNA fragmentation is believed to be a common property of all sperms. However, the level of DNA damage may vary from one sperm to another . Recent studies show that sperm DNA fragmentation is also observed in men with normal semen analysis parameters . This may be due to intrinsic factors, where poor organization of sperm chromatin leaves the sperm vulnerable to oxidative stress-mediated DNA damage. Extrinsic factors such as medication, heat, radiation, etc . are known to induce DNA damage . The damage occurring to sperm (chromosomal abnormality or DNA fragmentation) is believed to be an irreversible process as the sperm lacks the necessary DNA repair mechanism to fix it .
Over the past decades, numerous inverse relationships between sperm genetic abnormalities and male infertility and assisted reproductive treatment (ART) outcomes have been reported. These studies have employed a range of diagnostic assays including the chromomycin A3 test , terminal deoxynucleotidyl transferase , in situ nick translation , sperm chromatin structure assays , comet assay , sperm chromatin dispersion assay , protamine ratio analysis , and sperm chromosomal aneuploidy evaluation . In this chapter, we review the causes of sperm DNA abnormalities, tests available to detect them, and effect of these abnormalities on male infertility and ART outcomes.
Cause of sperm chromosome/DNA abnormalities
Sperm genetic abnormalities are common and recognized as indicators of male infertility . Such abnormalities can be broadly classified into DNA fragmentation, abnormal compaction, and chromosomal aberrations. Therefore, it is beneficial to determine the possible cause of these abnormalities as part of the management program for couples undergoing infertility diagnosis and treatment.
Meiotic recombination
Meiotic recombination during the midpachytene stage is essential for genetic diversity and proper chromosomal segregation. While proper segregation is tightly regulated through the number of recombinant foci and cross-over events, errors can occur and result in aneuploid sperm . Several studies have identified that infertile men do have a lower rate of meiotic recombination , indicating that these errors cause not only a reduction in total sperm count but also an increase in sperm aneuploidy risk .
Meiotic recombination
Meiotic recombination during the midpachytene stage is essential for genetic diversity and proper chromosomal segregation. While proper segregation is tightly regulated through the number of recombinant foci and cross-over events, errors can occur and result in aneuploid sperm . Several studies have identified that infertile men do have a lower rate of meiotic recombination , indicating that these errors cause not only a reduction in total sperm count but also an increase in sperm aneuploidy risk .
Abortive apoptosis
Apoptosis in the male germ line is a complex cascade of events where abnormal cells are marked and ultimately degenerated prior to completing spermatogenesis. This process is leaky, and some spermatocytes do escape degeneration. These sperms yield a population of spermatozoa with increased DNA damage and retained apoptotic markers . It is believed that a majority of the abnormal sperm population is eliminated during spermatogenesis . Studies using a mouse model have shown that pro- and anti-apoptotic factors play critical roles in spermatogenesis and maintain an appropriate number of male germ cells . It is postulated that Sertoli cells start to regulate germ cell apoptosis through the apoptosis-stimulating fragment system (Fas), which is characterized by the interaction between Fas protein and Fas ligand . Apoptosis involves activation of endonucleases, which induce DNA strand breaks, leading to cell death . An early sign of apoptosis in sperm is translocation of the negatively charged phospholipid phosphatidylserine from the inner to the outer side of the plasma membrane . Binding of Fas ligand to Fas protein induces apoptosis . It is hypothesized that Sertoli cells express Fas ligand, which could be involved in the regulation of spermatogenesis, and that they could regulate the number of spermatocytes associated with them for support . It has also been proposed that programmed cell death may be responsible for the selective depletion of abnormal germ cells with abnormal morphology, altered function, or DNA damage . In support of this selective process, there are data that show a higher incidence of Fas positivity in the sperm of infertile men . These studies together suggest that apoptosis is possibly a pathway for the optimization of spermatogenesis and male fertility. This process seems leaky and results in mature sperm with increased DNA damage. It is interesting to note that while these sperms may be able to complete fertilization, they are less likely to contribute to a live birth.
Infection
Infections in the male genital tract, as indicated by leukospermia, are associated with sperm DNA damage . Leukocytes are known to produce reactive oxygen species (ROS), which could cause DNA base modification and DNA fragmentation. The adverse effect of leukospermia depends not only on the number of leukocytes generated but also on the activation state of leukocytes . Seminal leukocytes originate from the epididymis and secondary sexual glands . ROS produced by leukocytes are scavenged by antioxidants present in the seminal plasma . However, if the level of ROS generated is higher than the protection offered by the seminal plasma, then sperms are subjected to oxidative stress-mediated DNA damage . Studies have not delineated a difference in DNA damage originating from ROS and that from other sources that are detrimental to fertility.
Xenobiotic exposure
Exposure to xenobiotics can be classified into three major routes: occupational, pharmacological, and environmental. Regardless of the exposure route, male germ cells are particularly vulnerable to xenobiotic action, which results in DNA damage . In support of this fact, studies have shown that sperm DNA damage is higher among coke oven workers exposed to polycyclic aromatic hydrocarbon . In addition, work by Oh et al. has shown higher sperm DNA damage in waste incineration workers in comparison to controls . Additionally, factory workers exposed to organic molecules, such as styrene, also show significant increase in sperm DNA damage . With advancements in molecular medicine and cancer treatment regimens becoming more effective, men of reproductive age surviving treatment and pharmacologic exposure of the testis are becoming more common. A well-known example for such an intervention is cyclophosphamide, which is used as chemotherapeutic agent to treat cancer and is shown to cause an increase in sperm DNA damage . Environmental estrogens are abundant in food and water and known to induce sperm DNA damage . Organo chlorides and smog have also been established as aggressive inducers of sperm DNA damage. Currently, there are few studies assessing the overall population risk on the induction of male factor infertility from xenobiotic exposures. Large population studies are needed to better understand the epidemiology of these exposures.
Oxidative stress
In recent years, generation of ROS in the male reproductive tract has been a matter of concern . ROS is defined as any atom or molecule that contains one or more unpaired electron(s) , which confers a high reactive state and increases the oxidation potential. Higher levels of ROS have been reported in the seminal plasma of infertile men than in fertile men . Moreover, there are mechanisms by which sperm DNA is protected from this oxidative damage by compact packaging of the sperm DNA and presence of antioxidants in the seminal plasma . Sperms are vulnerable to oxidative stress-mediated damage because of the presence of a large amount of polyunsaturated fatty acids in the plasma membranes ; however, the exact mechanism by which ROS induces DNA damage is poorly understood. We do understand that an imbalance between ROS generation and the level of antioxidants present in the seminal plasma may result in sperm DNA fragmentation . Moreover, there is evidence that suggests that high levels of ROS are correlated with an increased percentage of single- and double-strand DNA damage in the sperm .
Studies show that there is a significant correlation between ROS and DNA fragmentation and increased DNA damage in the sperm by artificial introduction of ROS by hydrogen peroxide . The documented mode of ROS-induced DNA damage is in the form of strand breaks, modified bases, DNA cross-links, frame shifts, production of base-free sites, and chromosomal rearrangements . ROS are also known to cause gene mutations such as point mutations, polymorphisms , and DNA base-pair oxidation in sperm. Another interesting possible mechanism and exciting area to explore is the occurrence of ROS-mediated DNA damage through the formation of DNA adducts, which are modified bases and strand breaks. This DNA adduct is considered to be an important biomarker for oxidative DNA damage in other tissue types .
Tests for sperm chromosome/DNA abnormalities (sperm chromosome packaging, DNA integrity and fragmentation, and aneuploidies)
Regardless of the causes of DNA abnormalities in sperm, the measurement of these abnormalities is important. In particular, and as discussed here, poor organization of sperm chromatin, chromosomal ploidy, and increased DNA fragmentation are known to impair reproductive outcome. Presently, the major sperm DNA testing methods are sperm chromatin structure assay (SCSA), terminal deoxynucleotidyl transferase dUTP nick end labeling (TUNEL) assay, sperm chromatin dispersion (SCD) assay, in situ nick translation (ISNT), comet assay, and sperm aneuploidy screening.
Comet assay
The comet assay is one of the simplest and most affordable methods to measure sperm DNA damage by quantifying the single- and double-strand breaks . The principle of the assay is that sperm nuclear DNA is separated in an electric field based on charge and fragment size, which can then be stained with a fluorescent dye, viewed, and graded directly by visualization using a fluorescence microscope in a qualitative manner or using a quantitative analysis software package. The resulting images, which resemble comets with intact heads and tails depending on the amount of DNA damage and intensity of staining, determines the extent of damage . Additional parameters have been used to increase the efficiency of the test such as diameter of the nucleus, olive tail moment, and the comet length . One of the principles of the comet assay is that double-stranded DNA remains in the comet head and shorter fragments of double- and single-stranded DNA migrate into the tail area . Therefore, the sperm with high levels of DNA strand breaks would show increased comet tail, measured by the intensity of fluorescence in the head and tail regions and the length of comet tail . The comet assay can be performed in a neutral or alkaline environment; in the neutral buffer, only double-strand DNA damage is measured, while in the alkaline buffer, both single- and double-strand DNA damage and alkali-labile sites are detectable due to the unwinding of the strands . Comet assay is the only technique that can qualitatively measure the level of DNA damage in individual cells.
The comet assay is inexpensive and one of the most sensitive techniques available to measure DNA damage, and according to a previous study , the results of the comet assay are correlated to the results obtained from the TUNEL assay. The alkaline comet assay can be used in all cell types, including sperm , and requires only a few cells for the analysis. The clinical importance of the comet assay in assessing male infertility has been demonstrated by a number of authors . The disadvantage of the assay is that it lacks standardized protocols, which makes it difficult to fully understand and relate the results obtained from studies of different authors . As discussed above, the alkaline comet assay also marks alkali-labile sites and therefore makes it difficult to discriminate between endogenous and induced DNA breaks. The assay is also criticized for underestimation of DNA damage due to the entanglement of DNA strands. Incomplete chromatin decondensation, in the case of sperm DNA, will not allow the breaks to be revealed. Overlapping comet tails decrease the accuracy of the assay, and small tail fragments may be lost or difficult to visualize. The assay is laborious, has high level of inter-laboratory variation, and hence has been deemed by some as not-recommended for clinical use .
Sperm chromatin structure assay
The sperm chromatin structure assay (SCSA) is the largest available commercial test to characterize male infertility related to sperm DNA damage and chromatin abnormalities. It is a flow cytometric method to determine abnormal sperm chromatin, which is highly susceptible to physically induced in situ partial DNA denaturation. The extent of DNA denaturation is determined by measuring the metachromatic shift from green fluorescence to red fluorescence of acridine orange as it intercalates into DNA after heat or acid treatment . The most important parameter of this test is the DNA fragmentation index (DFI) (%), which represents the population of cells with DNA damage . It also measures the high DNA stainability (HDS) (%). The SCSA measures the susceptibility of sperm DNA to heat- or acid-induced DNA denaturation in situ after staining with acridine orange stain where the dye-bound double-strand DNA fluoresce green and the dye-bound single-strand DNA fluoresce red . The use of flow cytometry makes it possible to measure a large number of spermatozoa per sample, making the technique highly reproducible . DFI represents the sperm population with detectable denaturable single-stranded DNA and the HDS cells describe the sperm population with increased accessibility of double-stranded DNA to the dye, mainly due to the impaired replacement of histones with protamines .
Sperm DNA damage measured by SCSA is known to be more constant over a longer period of time when compared with the traditional sperm evaluation parameters . The consistency of the test makes it useful in epidemiological studies . Freezing of the semen samples does not affect the test, allowing samples to be batched for convenience or used in multi-center trials and analyzed in a central facility. The assay can determine the percentage of sperm with damaged DNA. Several clinical studies have shown its usefulness in evaluating male infertility . It is simple and rapid for the analysis of thousands of human sperm . Authors in earlier studies have found a threshold value above 30% DFI and 15% HSD for couples who are likely to be infertile. Several clinical studies have also shown its usefulness in evaluating male fertility in relation with fertilization, blastocyst development, ongoing pregnancy in in vitro fertilization (IVF), and Intracytoplasmic sperm injection (ICSI) . The SCSA does not give information about the extent of DNA damage in individual sperm , and the assay requires very expensive equipment for analysis. Laboratory factors can affect the test, which increase the variation between batches and even replicates . There are conflicting data regarding its usefulness in predicting fertilization rates, embryo quality, or pregnancy outcomes .
Terminal deoxynucleotidyl transferase-mediated dUTP nick end labeling assay
The terminal deoxynucleotidyl transferase-mediated dUTP nick end labeling (TUNEL) assay leverages the incorporation of deoxyuridine triphosphate (dUTP) at single- and double-strand DNA breaks in a reaction catalyzed by the template-independent enzyme, terminal label deoxynucleotidyl transferase . The incorporated dUTP, which labels the breaks, can be quantified by flow cytometry or qualitatively observed under fluorescence or light microscopy, depending on the conjugated indicator . The TUNEL assay is widely known to measure direct sperm DNA fragmentation. The TUNEL assay resembles the nick translation in situ in a number of technical aspects and can reveal both single- and double-strand damage . The sperm DNA fragmentation, measured by TUNEL assay, is stable with time, and it is thus possible to measure baseline damage in both fertile and subfertile men . The assay is broadly used to assess sperm DNA fragmentation and its value as an indicator of male infertility and has been demonstrated to predict the outcome of assisted reproduction .
TUNEL assay simultaneously detects single- and double-strand breaks, unlike other assays, which either measure a sperm’s susceptibility to DNA damage or require elaborate protocols to study both types of strand breakages . Freezing raw or prepared samples does not affect the results of the TUNEL assay, which increases the ease of its use in research and clinical settings . The TUNEL assay is more expensive than other methods; however, its popularity is justified by good quality control parameters, such as low intra- and inter-observer variability . The anti-digoxigenin-peroxidase labeling technique eliminates the problems associated with dye fading in the fluorescence microscopic method, thereby giving technicians more time to analyze a greater number of cells . The use of the TUNEL assay in flow cytometry also makes it possible to evaluate a very high number of cells, thus enhancing the reproducibility of the technique. The TUNEL assay does not quantify the magnitude of DNA damage within a cell; it only counts the number of cells within a population with a threshold level of DNA damage, reported as TUNEL-positive cells . It should be noted that a nuclear decondensation technique is not typically used with this assay, and hence, the labeling of DNA fragments is likely restricted to the periphery of the sperm nucleus; this is due to the unique DNA packaging in sperm. Relaxation of sperm DNA prior to labeling should be further evaluated .
In situ nick translation
The in situ nick translation (ISNT) assay is a modified form of TUNEL assay that quantifies the incorporation of biotinylated dUTP at single-stranded DNA breaks in a reaction that is catalyzed by the template-dependent enzyme DNA polymerase I . Unlike the TUNEL assay, which uses template-independent end labeling, nick translation can only be used for single-strand breaks and not for both single- and double-strand breaks as used in the TUNEL assay . This assay identifies sperm that contains low and variable levels of endogenous DNA damage and positively associated with protamine deficiency . The clinical value of the nick translation assay is severely limited because no correlation has been proven for fertilization during in vivo studies with it , and unlike other assays, it lacks sensitivity . Furthermore, data suggests that the single-strand breaks are more likely to be repaired by the embryo than the double-strand breaks . This in turn suggests that measurement of only single-strand breaks may be less clinically relevant.
Because of the efficiency of the DNA polymerase enzyme, which is used in the assay, the single-strand nicks are incorporated with labeled dUTP, and hence, very low levels of fluorescence are identified in the sperm. Unlike other assays, this assay has a very low dynamic range and lacks sensitivity . Because no correlation has been proven with fertilization in in vivo studies, the clinical value of the nick translation assay is severely limited . Both the TUNEL and comet assays show better correlations with ART outcomes than ISNT because they measure both single- and double-strand breaks present in the sperm DNA .
Sperm chromatin dispersion assay
The sperm chromatin dispersion (SCD) assay has been described as a simple and inexpensive method for the analysis of sperm DNA fragmentation. It is based on the principle that sperm with fragmented DNA fail to produce the characteristic halo when mixed with agarose, following acid denaturation and removal of nuclear proteins . The methodology of the test includes the following steps: sperms are immersed in an agarose matrix on a slide, treated with an acid solution to denature DNA that contains breaks, and treated with lysis buffer to remove membranes and proteins. The agarose matrix allows working with unfixed sperm on a slide in a suspension-like environment. Removal of the sperm nuclear proteins results in a nucleoid with a central core and a peripheral halo of dispersed DNA loops. Using a fluorescent stain, the sperm nuclei with elevated DNA fragmentation produce very small or no halos of DNA dispersion, while sperms with low levels of DNA fragmentation release their DNA loops, forming large halos. This biochemical phenomenon has been confirmed by DNA breakage detection-fluorescence in situ hybridization (FISH), a procedure in which the restricted single-stranded DNA motifs generated from DNA breaks can be detected and quantified .
The SCD assay is a simple, fast, highly reproducible, and inexpensive technique. The test does not rely on fluorescence intensity; hence, it is simple to analyze. The test does not require the use of complex instrumentation, so it can be carried out in a routine andrology laboratory, and the qualitative test endpoints (non-dispersed and dispersed nuclei) provide simple quality control mechanisms. There are also some drawbacks of the SCD assay. SCD has been reported to have low-density nucleoids, which are fainter with less contrasting images. Thus, the peripheral limit of the halo, where the chromatin is less dense, may not be accurately discriminated from the background. All the halos are not in the same plane; hence, the use of analysis software may result in misreading due to unfocused halos. Moreover, sperm tails are not preserved; therefore, discriminating sperm from other contaminant cells is problematic.
Sperm aneuploidy screening with fluorescent in situ hybridization
The use of inter-phase chromosomal testing for aneuploidies in sperm using has enabled the routine screening of men in at-risk populations with high polyploidies and nullisomies. These at-risk populations are men of advanced reproductive age (over 40 years), severe oligozoospermia, non-obstructive azoospermia, Klinefelter syndrome, chromosomal translocations, severe teratozoospermia, increased macrocephalic sperm, increased multiflagellar, globozoospermia, unexplained recurrent pregnancy loss, and repeated IVF failure . Application of FISH to interphase sperm nuclei has been described previously . In brief, sperms are concentrated onto glass slides, fixed, treated with a decondensation agent to allow the probe to access the tightly packaged sperm DNA, hybridized with a cocktail of centromeric and/or site-specific chromosomal probes, washed, and then 2000–5000 sperm are evaluated for ploidy. Automated platforms are available for classifying the sperm, which may lessen the technician’s time needed for evaluation . Routine evaluation typically employs a set of five chromosomes (X, Y, 13, 18, 21), while others may be needed for differential diagnosis of infertility. For instance, it has been shown that chromosomes 1, 15, 17, 21, and 22 may be more predictive of early miscarriage .
Impact on infertility and assisted reproduction
Several indicators of male reproductive health including physiological levels of male reproductive hormones and semen quality have been extensively studied , and data suggest a decline in male reproductive health in the past few decades. Carlsen et al. and Andersson et al. showed a clear pattern of declining semen quality among young men and an increase in sperm genetic abnormalities, leading to hypospadias and cryptorchidism. While semen parameters are not prognostic for ART success, they are essential for initial diagnosis of male infertility; decline in semen quality is associated with decline in male reproductive health.
Measurements from recent studies, as cited herein, have shown that sperm DNA and chromosomal abnormalities are closely associated with male reproductive health and ART success.
Impact on male infertility
In most infertility clinics, the diagnosis of male infertility is limited to semen analysis, which is performed during diagnosis or prior to ART. Semen analysis is considered the cornerstone of male infertility diagnosis and includes the measurement of semen volume, liquefaction time, pH, sperm count, motility, morphology, and contamination by other cells . The semen analysis has not been regarded as an accurate test for male fertility potential or male infertility, except in the case of azoospermia . Proteomic analysis of human sperm shows possible protein differences between normal and asthenozoospermic individuals . Detection of mutations in functional genes provides important evidence to determine the basis of infertility. Individuals with Kartagener syndrome have mutations in dynein genes resulting in severe asthenozoospermia . Other mutations in genes coding for dyneins of the flagella are also found in men with asthenozoospermia .
Karyotyping is the standard test to evaluate the chromosomal content; studies show that sperm of men suffering from infertility may contain numerical or structural chromosomal defects . Klinefelter syndrome is seen in 14% of patients with nonobstructive azoospermia . Recently, molecular karyotyping using genomic hybridization microarray has been used for the diagnosis of structural chromosome rearrangements . Molecular karyotyping has also been used in detecting Y chromosome microdeletions to determine the etiology of severe oligozoospermic and nonobstructive azoospermic patients . Assessment of aneuploidy in sperm by FISH may elucidate errors in spindle formation, chromosome cohesion, and chromosome segregation during meiosis. Increased aneuploidy rates are seen in men with unexplained infertility and couples diagnosed with early pregnancy loss . Accordingly, chromosomal abnormalities are shown to be higher in infertile men than in fertile men . While many of these diagnostic tests are not routinely used, the etiology of these defects could provide useful information and aid important decision-making during fertility treatment.
In recent years, a number of studies have compared the level of sperm DNA fragmentation between fertile and infertile men . Most studies suggest that sperm DNA fragmentation is more common in infertile patients and may be a contributing factor for their reproductive health. An increase in sperm DNA fragmentation can be attributed to a number of factors, such as abnormal histone to protamine exchange , abnormal protamine content and ratio , and oxidative stress in the seminal plasma . A study examining the effect of sperm DNA fragmentation among first pregnancy planners with no previous knowledge of their fertility suggested that fecundity declined with an increase in sperm DNA fragmentation and concluded the necessity of normal sperm chromatin for the expression of full male fertility potential . From the available literature, it can be postulated that abnormalities in sperm genetic material can be considered as indicators of the status of male reproductive health, and these tests, in addition to semen analysis, may help diagnose male infertility.
Effect on assisted reproduction
The existing literature associating sperm DNA damage with ART outcomes is controversial. Some studies show a strong influence of sperm DNA fragmentation on male reproductive health and that sperm DNA testing should be incorporated into routine clinical tests , whereas other clinical reviews do not support the clinical use of sperm DNA fragmentation testing .
In conjunction with this review, we performed a literature search and meta-analysis, which identified 90 articles (120 studies) associating sperm DNA fragmentation with ART outcomes ( Table 1 ) using the four commonly used assays (SCSA, TUNEL, SCD, and comet assays). When clinical pregnancy was evaluated, studies that did not control for female age and/or female factor infertility were excluded from analysis.
Study | ART type | Assay | (n) | Correlation with ART outcomes | ||
---|---|---|---|---|---|---|
Fertilization rate | Embryo quality | Clinical pregnancy | ||||
Anifandis et al., 2015 | IVF + ICSI | SCD | 139 | Non-significant | Significant | Non-significant |
Avendano et al., 2010 | ICSI | TUNEL | 36 | NA | Significant | Significant |
Baker et al., 2013 | IVF + ICSI | TUNEL | 48 | NA | NA | Non-significant |
Bakos et al., 2007 | IVF | TUNEL | 45 | Significant | Non-significant | Non-significant |
IVF + ICSI | TUNEL | 113 | Non-significant | Non-significant | Significant | |
ICSI | TUNEL | 68 | Non-significant | Non-significant | Significant | |
Benchaib et al., 2003 | IVF | TUNEL | 50 | Significant | Non-significant | Non-significant |
ICSI | TUNEL | 54 | Significant | Non-significant | Non-significant | |
Benchaib et al., 2007 | IVF | TUNEL | 84 | Non-significant | Non-significant | Non-significant |
ICSI | TUNEL | 218 | Significant | Significant | Non-significant | |
Boe-Hansen et al., 2006 | IVF | SCSA | 139 | NA | NA | Non-significant |
ICSI | SCSA | 47 | NA | NA | Non-significant | |
Borini et al., 2006 | IVF | TUNEL | 82 | Significant | NA | Non-significant |
ICSI | TUNEL | 50 | Non-significant | NA | significant | |
Bounartzi et al., 2016 | IVF + ICSI | SCD | 55 | Non-significant | Non-significant | Significant |
Bradley et al., 2016 | ICSI | SCSA | 2175 | Non-significant | Non-significant | Non-significant |
Bungum et al., 2007 | IVF | SCSA | 388 | Non-significant | Non-significant | NA |
ICSI | SCSA | 223 | Non-significant | Non-significant | NA | |
Caglar et al., 2007 | ICSI | TUNEL | 56 | Non-significant | Non-significant | Non-significant |
Check et al., 2005 | ICSI | SCSA | 106 | NA | Significant | Non-significant |
Chi et al., 2011 | IVF | Neutral Comet | 36 | Significant | Non-significant | Non-significant |
ICSI | Neutral Comet | 55 | Significant | Non-significant | Non-significant | |
Dar et al., 2013 | ICSI | SCSA | 153 | Non-significant | NA | Non-significant |
Daris et al., 2010 | ICSI | TUNEL | 20 | Non-significant | NA | NA |
Esbert et al., 2011 | IVF + ICSI | TUNEL | 178 | Non-significant | Non-significant | Non-significant |
Fang et al., 2011 | IVF | SCSA | 111 | Significant | Non-significant | Non-significant |
Fortunato et al., 2016 | ICSI | SCD | 89 | NA | NA | Significant |
ICSI | TUNEL | 89 | NA | NA | Non-significant | |
Frydman et al., 2008 | IVF | TUNEL | 117 | Non-significant | Non-significant | significant |
Gandini et al., 2004 | IVF | SCSA | 12 | Non-significant | NA | Non-significant |
ICSI | SCSA | 22 | Non-significant | NA | Non-significant | |
Garolla et al., 2016 | IVF + ICSI | TUNEL | 166 | NA | NA | Non-significant |
Gosalvez et al., 2013 | ICSI | SCD | 81 | NA | NA | Significant |
Gu et al., 2009 | IVF | SCD | 136 | Significant | NA | Significant |
Gu et al., 2011 | IVF | SCD | 67 | Significant | Non-significant | Significant |
Guerin et al., 2005 | IVF + ICSI | SCSA | 100 | Non-significant | Non-significant | Non-significant |
Haghpanah et al., 2016 | ICSI | SCD | 20 | Non-significant | Non-significant | NA |
Hammadeh et al., 2006 | IVF | TUNEL | 26 | Non-significant | NA | NA |
ICSI | TUNEL | 22 | Non-significant | NA | NA | |
Hammadeh et al., 2008 | IVF | TUNEL | 26 | Non-significant | NA | Non-significant |
ICSI | TUNEL | 22 | Non-significant | NA | Non-significant | |
He et al., 2016 | ICSI | SCD | 97 | Non-significant | Non-significant | Non-significant |
Henkel et al., 2003 | IVF | TUNEL | 208 | Non-significant | Non-significant | NA |
ICSI | TUNEL | 54 | Significant | Non-significant | NA | |
Host et al., 2000 | IVF | TUNEL | 175 | Significant | Significant | Non-significant |
ICSI | TUNEL | 60 | Non-significant | Non-significant | Non-significant | |
Huang et al., 2005 | IVF | TUNEL | 204 | Significant | Non-significant | Non-significant |
ICSI | TUNEL | 86 | Significant | Non-significant | Non-significant | |
Jiang et al., 2011 | IVF | SCSA | 137 | Non-significant | Non-significant | Non-significant |
ICSI | SCSA | 50 | Non-significant | Non-significant | Non-significant | |
Jin et al., 2015 | IVF | SCD | 2085 | NA | NA | Non-significant |
ICSI | SCD | 777 | NA | NA | Non-significant | |
Kennedy et al., 2011 | ICSI | SCSA | 233 | NA | NA | Non-significant |
Larson-Cook et al., 2003 | IVF + ICSI | SCSA | 82 | Non-significant | Non-significant | Significant |
Lazaros et al., 2013 | ICSI | SCSA | 36 | Significant | Significant | Significant |
Lewis et al., 2004 | ICSI | Comet | 77 | Non-Significant | NA | Significant |
Lin et al., 2008 | IVF | SCSA | 137 | Non-Significant | Non-significant | Non-significant |
ICSI | SCSA | 86 | Non-significant | Non-significant | Non-significant | |
Lopes et al., 1998 | ICSI | TUNEL | 131 | Significant | Non-significant | NA |
Lopez et al., 2013 | IVF + ICSI | SCD | 152 | NA | NA | Non-significant |
Marchetti et al., 2002 | IVF | TUNEL | 111 | Significant | NA | NA |
Meseguer et al., 2011 | IVF + ICSI | SCD | 210 | NA | NA | Significant |
Micinski et al., 2009 | ICSI | SCSA | 60 | Significant | Non-significant | NA |
Morris et al., 2002 | IVF + ICSI | Comet | 60 | Non-significant | Significant | Non-significant |
Muratori et al., 2016 | IVF + ICSI | TUNEL | 90 | NA | NA | Non-significant |
Muriel et al., 2006 | IVF + ICSI | Halo | 85 | Significant | Significant | Non-significant |
Na & Li et al., 2011 | IVF | SCD | 51 | Significant | Non-significant | NA |
Nasr-Esfahan et al., 2005 | ICSI | Comet | 28 | Non-significant | Significant | NA |
Ni et al., 2014 | IVF | SCD | 1380 | NA | Non-significant | Non-significant |
ICSI | SCD | 355 | NA | Non-significant | Non-significant | |
Nicopoullos et al., 2008 | ICSI | SCSA | 56 | Non-significant | NA | Non-significant |
Nijs et al., 2009 | IVF + ICSI | SCSA | 205 | Significant | NA | Non-significant |
Nijs et al., 2011 | IVF + ICSI | SCSA | 278 | Non-significant | NA | Non-significant |
Niu et al., 2011 | IVF | SCSA | 218 | Non-significant | Significant | Non-significant |
Nunez-Calonge et al., 2012 | ICSI | SCD | 70 | Non-significant | NA | Significant |
Oleszczuk et al., 2016 | IVF | SCSA | 1117 | Significant | Non-significant | Non-significant |
ICSI | SCSA | 516 | Non-significant | Non-significant | Non-significant | |
Ozmen et al., 2007 | ICSI | TUNEL | 42 | Non-significant | Non-significant | NA |
Payne et al., 2005 | IVF + ICSI | SCSA | 95 | Significant | Non-significant | Non-significant |
Pregl Breznik et al., 2013 a | IVF | SCD | 133 | Significant | Significant | NA |
ICSI | SCD | 133 | Non-significant | Non-significant | NA | |
Rama Raju et al., 2012 | IVF + ICSI | SCD | 247 | Non-significant | Significant | Significant |
Seli et al., 2004 | IVF + ICSI | TUNEL | 49 | NA | Significant | NA |
Sharbatoghli et al., 2012 | ICSI | SCD | 120 | Non-significant | Non-significant | Non-significant |
ICSI | TUNEL | 120 | Non-significant | Non-significant | Significant | |
Simon et al., 2010 | IVF | Comet | 219 | Significant | Significant | Significant |
ICSI | Comet | 116 | Non-significant | Non-significant | Non-significant | |
Simon et al., 2011 | IVF | Comet | 70 | Significant | Significant | Significant |
Simon et al., 2011b | IVF | Comet | 70 | Significant | Significant | Significant |
ICSI | Comet | 22 | Non-significant | Non-significant | Non-significant | |
Simon et al., 2014 | IVF + ICSI | Comet | 238 | Significant | Significant | Significant |
IVF + ICSI | TUNEL | 238 | Significant | Non-significant | Significant | |
IVF + ICSI | SCSA | 102 | Non-significant | Non-significant | Non-significant | |
Smit et al., 2010 | IVF + ICSI | SCSA | 27 | NA | NA | Non-significant |
Smit et al., 2013 | IVF + ICSI | SCSA | 19 | NA | NA | Significant |
Speyer et al., 2010 | IVF | SCSA | 192 | Non-significant | Non-significant | Non-significant |
ICSI | SCSA | 155 | Non-significant | Non-significant | Significant | |
Speyer et al., 2015 | IVF | SCSA | 200 | Non-significant | Non-significant | Non-significant |
ICSI | SCSA | 199 | Non-significant | Non-significant | Non-significant | |
Sun et al., 1997 | IVF | TUNEL | 143 | Significant | Significant | NA |
Tandara et al., 2013 | IVF + ICSI | SCD | 72 | NA | Significant | NA |
Tandara et al., 2014 | IVF | SCD | 88 | Significant | Significant | Significant |
IVF | SCSA | 88 | Significant | Significant | Significant | |
Tarozzi et al., 2009 | IVF | TUNEL | 82 | NA | NA | Non-significant |
ICSI | TUNEL | 50 | NA | NA | Significant | |
Tavalaee et al., 2009 | IVF + ICSI | SCD | 92 | Non-significant | Non-Significant | Non-significant |
Tavalaee et al., 2017 | ICSI | TUNEL | 35 | Non-significant | Significant | Non-significant |
Tomsu et al., 2002 | IVF | Comet | 40 | NA | Significant | Non-significant |
Velez de la Calle et al., 2008 | IVF + ICSI | SCD | 622 | Significant | Significant | Non-significant |
Virro et al., 2004 | IVF + ICSI | SCSA | 249 | Non-significant | Significant | Significant |
Wang et al., 2014 | ICSI | SCD | 45 | Non-significant | NA | Non-significant |
Wdowiak et al., 2015 | ICSI | SCD | 165 | Significant | Significant | Significant |
Wdowiak & Bojar, 2016 | ICSI | SCD | 148 | NA | NA | Non-significant |
Xue et al., 2016 | IVF | SCD | 415 | Non-significant | Non-significant | NA |
ICSI | SCD | 135 | Significant | Non-significant | Non-significant | |
Yang et al., 2013 | ICSI | SCSA | 62 | NA | NA | Significant |
Yilmaz et al., 2010 | ICSI | SCD | 60 | Non-significant | NA | NA |
Zhang et al., 2016 | IVF | SCSA | 1316 | NA | NA | Significant |
ICSI | SCSA | 266 | NA | NA | Significant | |
Zini et al., 2005 | ICSI | SCSA | 60 | Non-significant | Significant | Non-significant |
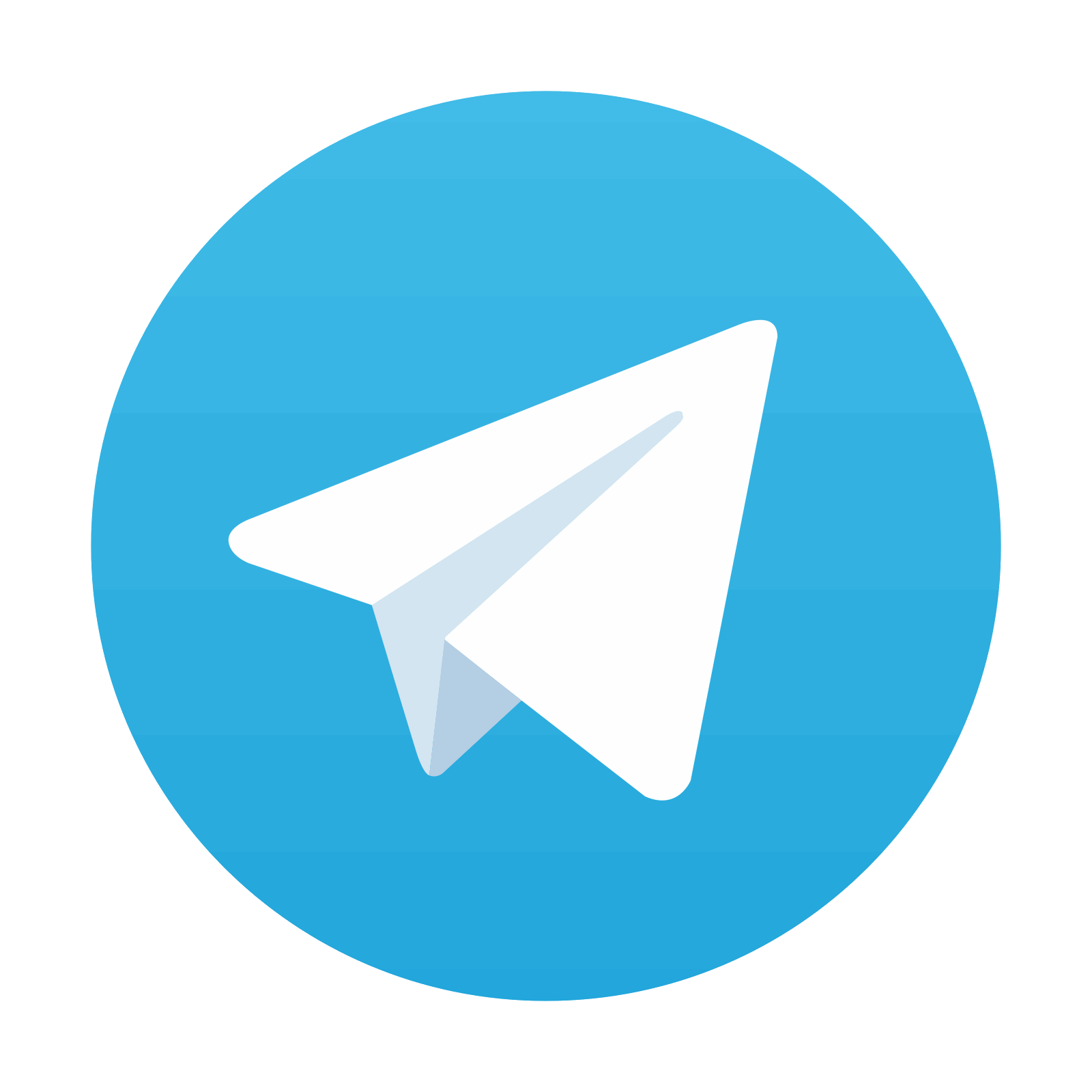
Stay updated, free articles. Join our Telegram channel

Full access? Get Clinical Tree
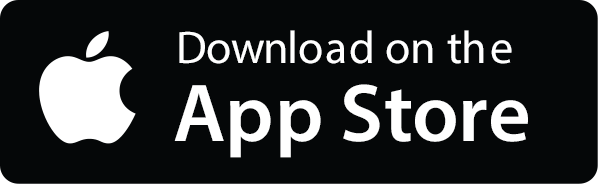
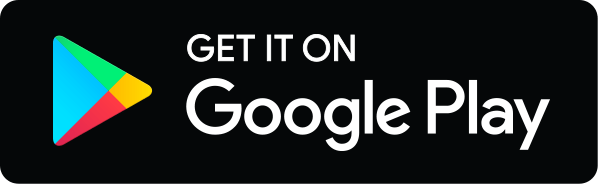
