Reproduction and Hormonal Contraception
Mitchell D. Creinin, MD
Jeffrey T. Jensen, MD, MPH
The discovery of the central regulatory role of hormones in reproduction led directly to the development of hormonal contraception. Beginning in 1919, Ludwig Haberlandt, a professor of physiology at the University of Innsbruck, Austria, performed a series of experiment to test the hypothesis advanced by Beard and Prenant that a product of the corpus luteum and placenta prevents ovulation.1 Haberlandt transplanted ovarian tissue from a pregnant rabbit under the dorsal skin of an adult nonpregnant rabbit and prevented pregnancy. He later showed similar results after injecting extracts of the corpus luteum in mice, calling the approach “hormonal sterilization.” The Viennese gynecologist Otfried Otto Fellner reported similar findings when administering ovarian and placental extracts to a variety of animals.2 Haberlandt proposed injection or oral administration of an agent as a technique for fertility control and collaborated with the Hungarian pharmaceutical company Gedeon Richter in the development of an extract named Infecundin that was never tested in women. Haberlandt’s early death of a heart attack in 1932, at age 47, brought an end to this effort, and Fellner disappeared after the annexation of Austria to Hitler’s Germany.
Although world events contributed to the delay of these research advances, the limited availability and low potency of natural steroid hormones (SHs) from animal sources presented a more significant obstacle. This chapter tells the story of the breakthroughs leading to the wide availability of synthetic steroids and the development of hormonal contraception. To provide a framework to understand how hormonal contraception works, we first review the normal events that control the reproductive cycle.
Reproductive Endocrinology and Physiology
A complete review of reproductive endocrinology and physiology is beyond the scope of this text. In this section, we summarize the key events that regulate the natural menstrual cycle and explain how hormonal therapy affects these processes to prevent pregnancy.
Steroid Hormones
The term steroid refers to a group of lipid hormones derived from cholesterol. The lipid-like nature of SHs allows them to easily pass through cell membranes. While many tissues can synthesize steroids, the gonads
(androgens, progesterone, estrogens) and adrenal glands (androgens, glucocorticoids, mineralocorticoids) are the primary SH endocrine organs. Most SHs circulate through the blood bound to specific carrier proteins. For example, estrogens and androgens circulate bound to sex hormone-binding globulin (SHBG), while glucocorticoids and progesterone bind to cortisolbinding globulin (CBG). Albumin binds all SHs with low affinity but high capacity. Only the free (unbound) hormone can exert a biologic response.3 Route of administration, dose, metabolism, and tissue-specific receptor activity of various natural and synthetic steroid and peptide hormones also influence the biologic response.
(androgens, progesterone, estrogens) and adrenal glands (androgens, glucocorticoids, mineralocorticoids) are the primary SH endocrine organs. Most SHs circulate through the blood bound to specific carrier proteins. For example, estrogens and androgens circulate bound to sex hormone-binding globulin (SHBG), while glucocorticoids and progesterone bind to cortisolbinding globulin (CBG). Albumin binds all SHs with low affinity but high capacity. Only the free (unbound) hormone can exert a biologic response.3 Route of administration, dose, metabolism, and tissue-specific receptor activity of various natural and synthetic steroid and peptide hormones also influence the biologic response.
The classical mechanism of SH action (Figure 2.1) requires that (1) the free SH ligand diffuses across the cell membrane; (2) the SH binds to the specific SH receptor in either the cytosol (glucocorticoids and mineralocorticoids) or cell nucleus (androgens, estrogens, progestogens); (3) the SH/receptor complex interacts with nuclear DNA to stimulate transcription of mRNA; (4) the mRNA is transported to the cytosol and ribosomes for translation; and (5) the protein synthesized causes specific cellular effects.4 The general take-home point is that classical SH action takes time (hours to days). In other words, the clinical response following administration of a steroid requires patience. Membranebound SH receptors are thought to mediate nonclassical rapid responses in some tissues through direct (nonnuclear) signal transduction pathways.
In the ovary, sex steroids are produced by ovarian stromal cells. The stromal cells that border each primary germ cell differentiate into granulosa cells to form a primordial follicle. As the follicle grows and develops, each oocyte becomes surrounded by a multilayer zone of granulosa cells bordered by theca cells. Under stimulation by gonadotropins, theca cells produce androgens and the granulosa cells aromatize these androgens to estrogens.4
Peptide Hormones
Peptide hormones also play key roles in reproduction. The major reproductive peptide hormones that control the reproductive cycle include the hypothalamic gonadotropin-releasing hormone (GnRH) and the anterior pituitary follicle-stimulating hormone (FSH) and luteinizing hormone (LH). FSH and LH are heterodimers consisting of two peptide chains. The alpha chains are nearly identical, while the beta chains differ and provide specificity. The beta chain of human chorionic gonadotropin (hCG), a related heterodimer peptide produced by syncitiotrophoblasts, is highly similar to beta LH, differing only by an additional 24 amino acids at the carboxy-terminal end that result in a longer half-life. Thus, hCG binds to the LH receptor but circulates longer with greater bioavailability.
Other important peptide hormones include inhibin B, a product of granulosa cells; activin, produced by both the pituitary and granulosa cells; and the neuropeptides kisspeptin, dynorphin, and neurokinin B.
Peptide hormones are water soluble and cannot pass through lipid membranes. They bind to membrane receptors and influence cellular processes by acting through a second messenger (Figure 2.2). Thus, unlike SHs, the
effects of peptide hormones are rapid. For example, binding of GnRH to the GnRH receptor of gonadotropic cells of the anterior pituitary leads to activation of a G protein and triggers events leading to the immediate release of FSH stored in lysosomes.
effects of peptide hormones are rapid. For example, binding of GnRH to the GnRH receptor of gonadotropic cells of the anterior pituitary leads to activation of a G protein and triggers events leading to the immediate release of FSH stored in lysosomes.
Receptor Agonists and Antagonists
We use the term ligand to refer to a molecule that binds to a receptor. Ligands that bind to receptors to directly effect a physiologic response are called agonists, while those that block the response are called antagonists. The configurational changes of synthetic steroids may increase binding at the target receptor to produce a pharmacologic response as either agonists or antagonists. Some molecules also have effects on nontarget receptors. For
example, levonorgestrel (LNG), a potent progestin, has weak activity at the androgen receptor.5
example, levonorgestrel (LNG), a potent progestin, has weak activity at the androgen receptor.5
Some ligands bind to a receptor and cause an agonist or antagonist result by modulating the receptor. As a result, agonist or antagonist action from the same ligand may be tissue specific. Moieties that act on estrogen receptors are called selective estrogen receptor modulators (SERMs), and those that act on the progesterone receptor are called selective progesterone receptor modulators (SPRMs). For example, ulipristal acetate (UPA) is an SPRM that acts like progesterone at the hypothalamus to block the LH surge but opposes the action of progesterone at the level of the follicle to prevent follicle rupture.6 While UPA also blocks progesterone receptor activity at the endometrium, this effect is less robust than another progesterone receptor modulator mifepristone.7
Tissue-specific reaction occurs due to the activity of cofactors that modulate the binding of the SH to its receptor. Two main distinct isoforms exist for both the estrogen (ERα and ERβ) and progesterone (PR-A and PR-B) receptors. In addition, some SH action is thought to occur through rapid signaling pathways (similar to peptide hormones) through membrane-bound receptors.4
The Hypothalamic-Pituitary-Ovarian Axis
Women experience regular menstrual cycles due to a highly regulated feedback loop known as the hypothalamic-pituitary-ovarian (HPO) axis (Figure 2.3). The feedback control resides within centers located in the hypothalamus that respond to endocrine feedback by regulating the pulsatile release of GnRH. Several important neuropeptides participate in these pathways and affect the regulation of the reproductive cycle in a variety of ways. For example, dopamine participates in the regulation of prolactin and gonadotropin suppression important for lactational amenorrhea during breast-feeding, catecholamines modulate GnRH pulsatility and may be a factor in stress-related amenorrhea, neuropeptide Y likely participates in cycle suppression seen with poor nutrition, and opioid peptides modulate GnRH response.4
Many of the effects of neuropeptides are mediated through their regulation of kisspeptin neurons in the hypothalamus. These neurons respond to the low levels of ovarian steroids that are present prior to the onset of menstruation and stimulate the pulsatile release of GnRH from GnRH neurons.8 GnRH travels thorough the hypophyseal portal vein to the anterior pituitary triggering the release of FSH. FSH stimulates granulosa cells of antral follicles to produce estradiol and inhibin B. FSH levels peak at about cycle day 4 to 6 of the follicular phase and then decline as rising levels of estradiol and inhibin B turn off the kisspeptin neurons, in turn reducing the stimulation of GnRH neurons though negative feedback. During this time, one follicle becomes dominant, likely due to paracrine signaling. The dominant follicle continues to grow and secrete estradiol and inhibin as the other follicles in the developing pool
regress and undergo apoptosis. This intricate control is critical for mono-ovulation and the successful production of singleton pregnancies. The growing dominant follicle also develops LH and progesterone receptors.4
regress and undergo apoptosis. This intricate control is critical for mono-ovulation and the successful production of singleton pregnancies. The growing dominant follicle also develops LH and progesterone receptors.4
As the dominant follicle grows to the preovulatory stage in the late follicular phase, estradiol negative feedback switches to positive feedback as a second population of kisspeptin neurons detect the peak estradiol level and stimulate the release of GnRH with a greater pulse frequency than in the early cycle. This in turn leads to the preferential release of LH from the anterior pituitary. This LH surge develops over approximately 50 hours.
Understanding LH surge regulation is essential to hormonal contraception physiology. The kisspeptin neurons that stimulate pulsatile GnRH release causing the LH surge are regulated by the neuropeptides neurokinin B (NKB) and dynorphin (DYN), an endogenous opioid. While incompletely understood, the interaction between NKB, DYN, and kisspeptin neurons allows for the negative feedback of estradiol and inhibin B on FSH release and subsequent positive feedback that stimulates LH release.9 Dynorphin appears responsible for the progesterone-mediated negative feedback on GnRH release that terminates the LH surge,8 the basis for the use of progestogens to block ovulation.
Two key take-home points deserve emphasis: (1) FSH secretion stimulates follicle growth under a system of negative feedback in response to estrogen levels; and (2) progesterone receptor action can prevent or blunt the LH surge. These details explain why combined estrogen-progestogen hormonal contraception methods block follicle development and prevent ovulation, while progestogen-only methods allow follicle growth but block ovulation, resulting in estradiol production and persistent follicles in some cases.
While the above description provides details essential to the understanding of the mechanisms of hormonal contraception, we have greatly simplified the system. For example, low levels of LH secreted during the follicular phase stimulate androgen production in ovarian theca cells essential as the substrate for aromatization to estradiol in granulosa cells. A small midcycle surge of FSH increases LH receptors in the granulosa cells, transforming them into an important source for progesterone production in the luteal phase. Activin, produced by the granulosa cells, acts in an autocrine and paracrine fashion to inhibit progesterone production by the granulosa cells during the follicular phase, and reduce androgen synthesis in thecal cells, and in an endocrine fashion to augment the secretion of FSH by stimulating the synthesis of GnRH receptors in the pituitary. Progesterone and estrogen act at the level of the pituitary to increase the pituitary release of LH at midcycle. Catechol estrogens and opioid peptides also influence the regulation of menstrual cycles.4
Development of a Dominant Follicle
Primary germ cells cease mitotic activity early in fetal life and become arrested at prophase 1 (diplotene) of the first miotic division as primary oocytes. A single layer of granulosa cells envelops each oocyte to form a primordial follicle. While many of these follicles undergo apoptosis, growth occurs with the addition of layers of granulosa and theca cells over a process that takes
about 300 days that is independent of gonadotrophins, leading to formation of primary and then secondary follicles. These follicles secrete antimüllerian hormone (AMH); the AMH level reflects the pool of healthy follicles.10
about 300 days that is independent of gonadotrophins, leading to formation of primary and then secondary follicles. These follicles secrete antimüllerian hormone (AMH); the AMH level reflects the pool of healthy follicles.10
With continued growth, secondary follicles develop a small fluid space called the antrum over a process that takes about 65 days. At this stage, these small antral follicles become dependent on gonadotropins for further growth. Recruitment of antral follicles likely occurs in multiple waves during the cycle.11 Under the influence of FSH, antral follicles grow and secrete estradiol and inhibin. While this process requires FSH, estradiol production results from the aromatization of androgens that are synthesized in the theca cells under the influence of low levels of LH also present during this time.12 Selection of the dominant follicle occurs in the midfollicular phase with continued growth to form a preovulatory follicle and apoptosis of the remaining cohort.13 Occasionally, more than one follicle will progress to the preovulatory stage and result in ovulation of more than one oocyte (and a multiple pregnancy should both fertilize). The administration of recombinant gonadotropins in controlled ovarian stimulation protocols overrides the regulation of the dominant follicle, allowing multiple follicles to progress to the preovulatory stage.
The fact that estrogen production by the ovaries requires androgen as a substrate is a point worth emphasizing. Although we think of the follicular phase as FSH dependent, small amounts of LH induce steroid synthesis in theca cells leading to the production and secretion of androstenedione, dehydroepiandrosterone, and testosterone. Under the influence of FSH, granulosa cells aromatize these androgens to estrogens. Excessive androgen production by theca cells results in symptoms of androgen excess such as acne and hirsutism. Combined hormonal contraception dramatically improves these symptoms through multiple pathways, one of which is by reducing gonadotropin stimulation, decreasing both LH and FSH. These actions provide the clinical basis for the use of combined hormonal contraceptives to treat acne and polycystic ovarian syndrome.
Ovulation and the Development of the Corpus Luteum
The LH surge initiates a series of events in the preovulatory follicle leading to a shift in SH synthesis to progesterone, resumption of meiosis by the oocyte, and the production of a variety of proteinases, collagenases, and prostaglandins needed for follicle rupture after approximately 36 hours.4 Although serum levels of progesterone rise slowly in response to the LH surge, progesterone action within the periovulatory follicle is essential for the mediation of several pathways essential for ovulation (Figure 2.4).14 For this reason, once the LH surge initiates, ovulation will often proceed even if the LH surge subsequently becomes blunted.
Understanding this mechanism provides the explanation for why UPA, a SPRM, is more effective that LNG, a progesterone receptor agonist, for emergency contraception. LNG dosing must occur prior to the start of the LH surge to prevent ovulation, while UPA remains effective if dosed prior to the LH peak. Clinically, this extends the window of effective treatment.
Following ovulation, progesterone production rises rapidly as the follicle is transformed into the corpus luteum. The corpus luteum secretes both progesterone and estradiol in response to continued episodic release of LH pulses and these steroids act centrally to suppress FSH and new follicle growth. Luteal regression begins after about 11 days in response to a variety of events. Progesterone and estradiol levels decline precipitously leading to reestablishment of conditions for a new follicular phase. Rising levels of
hCG that occur with pregnancy prevent luteolysis and maintain progesterone levels to prevent decidual shedding and the onset of menses.
hCG that occur with pregnancy prevent luteolysis and maintain progesterone levels to prevent decidual shedding and the onset of menses.
Menstruation
The endometrium consists of a basal layer of stem cells (basalis) and a functional layer (functionalis). During menses and the follicular phase of the ovarian cycle, rising levels of estradiol act through estrogen receptor to induce mitotic activity that begins in the basalis resulting in proliferation and the development of the functional layer.15 Endometrial growth requires the formation of new blood vessels, and this neovascularization is also under hormonal regulation. In addition to mitotic activity, estrogen signaling induces endometrial cells to synthesize progesterone receptors.
Following ovulation, rising progesterone levels stimulate the endometrium to cease mitotic activity and undergo a secretory transformation suitable for implantation of the developing embryo. In the absence of pregnancy and rescue of the corpus luteum by hCG, progesterone levels drop within 10 to 14 days as luteolysis occurs.
Progesterone withdrawal leads to an irreversible series of events in the endometrium that result in the onset of menstruation. These actions occur within 36 hours following the drop in progesterone.16 A key takeaway here is that normal menstruation requires estrogen-driven endometrial proliferation but occurs in response to progesterone-induced secretory transformation followed by progesterone withdrawal. Endometrial bleeding will occur following progestin withdrawal of an estrogen-primed endometrium even in the setting of continued estrogen stimulation. This explains why women using continuous estrogen therapy for postmenopausal hormone therapy experience bleeding following the sequential administration of progesterone or a progestin.
One of the important actions of progesterone signaling in the luteal phase is the down-regulation of both estrogen and progesterone receptors in the endometrial functionalis. This point deserves emphasis, as it explains why women using combined estrogen-progestogen products continuously typically develop inactive endometrium and amenorrhea following several cycles of administration. It also explains why women using combined hormonal contraception in standard cyclic regimens generally experience reduced withdrawal bleeding compared to natural cycles. In most women using standard cycles of combined methods, endometrial proliferation occurs during the hormone-free interval due to endogenous ovarian estradiol production. Upon reinitiation of the progestogen-dominant combined hormonal product, the proliferation is attenuated through progesterone receptor-mediated down-regulation. As this effect occurs through classical steroid hormone receptor action, it requires days for down-regulation to completely arrest proliferation. So, most women using combined hormonal methods experience cyclic bleeding due to endometrial proliferation that begins during the hormone-free interval.
During use of a combined hormonal contraceptive method in a traditional 21-day regimen followed by a 7-day hormone-free interval, cyclic bleeding typically occurs. During the hormone-free interval, FSH levels begin to rise and stimulate proliferation that begins in the basalis. The
endometrial cells express ER and PR. Once the intake of the active hormone combination occurs, the estrogen component continues to stimulate proliferation working through the ER, while the progestin begins to downregulate ER and PR, limiting further proliferation and initiating a secretory transformation. Menses occurs as a result of progestin withdrawal.
endometrial cells express ER and PR. Once the intake of the active hormone combination occurs, the estrogen component continues to stimulate proliferation working through the ER, while the progestin begins to downregulate ER and PR, limiting further proliferation and initiating a secretory transformation. Menses occurs as a result of progestin withdrawal.
Women using progestin-only methods of contraception experience variable patterns of bleeding. Many women and clinicians consider amenorrhea as the optimum bleeding pattern during contraception use. Using this criterion, the LNG 52 mg intrauterine system provides the most reliable results. Users of this method have very high local levels of LNG at the endometrium, and this results in profound down-regulation of estrogen receptor and proliferation. Women using injectable depot medroxyprogesterone acetate experience high rates of amenorrhea with prolonged use, likely due to high systemic exposure leading to a profound ovarian suppression and a potent endometrial effect. However, it typically takes several months for reduced bleeding to develop. Initially, the progestogen effect results in endometrial decidualization similar to pregnancy. Over time, spotting and bleeding are common as this endometrium becomes unstable, but eventually, an inactive endometrium develops.17 Results with contraceptive implants and progestinonly pills are less consistent, possibly due to lower endometrial levels of the progestogen and variable levels of suppression of ovarian estradiol secretion.
Some users of combined hormonal contraception experience breakthrough bleeding due to the relative dominance of the progestin component of the formulation. The management of breakthrough bleeding has led to a number of different combined hormonal regimens designed to increase endometrial proliferation (see Chapter 7). The etiology of breakthrough bleeding during the use of a progestogen-dominant contraceptive may also be abnormal blood vessel development. Successful implantation and pregnancy require substantial new blood vessel development in the endometrium. This neovascularization occurs primarily during the proliferative phase under estrogen regulation. Two types of estrogen receptor have been identified, ERα and ERβ. While most of the effects of estrogens in the reproductive tract are mediated by ERα, the endothelial cells of the endometrium express only ERβ.18 Among the many strategies used clinically to manage breakthrough bleeding, the most successful involve increasing the dose of estrogen. Part of this effect may be mediated through stabilization of blood vessels. Recently, the use of tamoxifen, a SERM that is an antagonist for ERβ but a partial agonist for ERα, was shown to be effective for the management of breakthrough bleeding in users of contraceptive implants.19
The Cervix
Cervical mucus changes in response to the hormonal changes during the menstrual cycle in direct relationship to cyclic fluctuations of estrogen and progesterone. Prior to ovulation, in response to rising levels of estradiol, cervical mucus becomes fluid and penetrable by sperm. Following ovulation, in response to rising levels of progesterone, mucus thickens and becomes impenetrable. The ability of sperm to penetrate mucus is highest
with ovulatory mucus and lowest during the luteal and early follicular phases. Mucus provides an important natural barrier for the upper female genital tract; by restricting penetrability to midcycle, the system reduces the opportunity for ascending infection. The dynamic nature of cervical mucus and its hormonal regulation provide an important mechanism for contraception. Since ovulation commonly occurs in users of the LNG intrauterine system, the contraceptive effect likely results from the progestin-induced thickening of cervical mucus. Progestin-only pills that allow ovulation are thought to act by inhibiting the development of favorable mucus.20
with ovulatory mucus and lowest during the luteal and early follicular phases. Mucus provides an important natural barrier for the upper female genital tract; by restricting penetrability to midcycle, the system reduces the opportunity for ascending infection. The dynamic nature of cervical mucus and its hormonal regulation provide an important mechanism for contraception. Since ovulation commonly occurs in users of the LNG intrauterine system, the contraceptive effect likely results from the progestin-induced thickening of cervical mucus. Progestin-only pills that allow ovulation are thought to act by inhibiting the development of favorable mucus.20
Mechanisms of Action of Hormonal Contraception
Combined hormonal methods contain both estrogen and progestin components. The primary mechanism of action involves blocking ovulation through the inhibition of gonadotropin secretion via an effect on both pituitary and hypothalamic centers. The progestational agent primarily suppresses LH secretion (and thus prevents ovulation), while the estrogenic agent suppresses FSH secretion (and thus prevents the emergence of a dominant follicle). Therefore, the estrogenic component contributes to the contraceptive efficacy by attenuating follicle growth. However, an estrogenonly product would not provide a practical approach even with complete follicle suppression due to unopposed endometrial proliferation.
The estrogen in the combined hormonal methods serves two other purposes. It opposes the progestin effect on the endometrium so that the two hormones balance each other to minimize irregular shedding and unwanted breakthrough bleeding, and the presence of estrogen potentiates the action of the progestational agents. The latter function of estrogen has allowed reduction of the progestin dose in CHCs over the past few decades. The mechanism for this action is probably estrogen’s effect in increasing the concentration of intracellular progesterone receptors. Therefore, this minimal pharmacologic level of estrogen contributes to the efficacy of the combined methods.
Because the effect of a progestational agent will always take precedence over estrogen (unless the dose of estrogen is increased many, many fold), the endometrium, cervical mucus, and tubal function reflect progestational stimulation during continuous use. As noted above, the progestin strongly down-regulates proliferation and leads to a decidualized endometrium. Continuous use of a progestin leads to exhausted and atrophied glands, due to down-regulation of estrogen receptor. While it is tempting to conclude that these endometrial changes contribute to a contraceptive effect, we have no direct evidence for this action. For hormonal methods that work primarily by blocking ovulation, studies have not differentiated endometrial effects in cycles when a dominant follicle and ovulation occur from the effects seen in cycles when ovulation does not occur. Whether the endometrium that develops in an anovulatory cycle would be receptive is a moot point. We also know that the fertilized egg is capable of implanting ectopically. Suggesting that an effect on implantation is a mechanism of action for hormonal contraception without direct experimental evidence contributes to a narrative
used by special interest groups opposed to contraception to combat public funding. Even high doses of a progestin (e.g., LNG emergency contraception) or antiprogestin SPRMs (UPA) given post ovulation do not appear to alter key implantation factors.21,22 Most studies simply evaluate endometrial proliferation by ultrasound using stripe thickness as a surrogate for a contraceptive effect, an unproven hypothesis. In fact, data from a series of infertile women treated with clomiphene and intrauterine insemination found no difference in pregnancy rates with endometrial stripe thickness of less than 6 mm (15%), 6 to 9 mm (16%), or greater than 9 mm (19%).23
used by special interest groups opposed to contraception to combat public funding. Even high doses of a progestin (e.g., LNG emergency contraception) or antiprogestin SPRMs (UPA) given post ovulation do not appear to alter key implantation factors.21,22 Most studies simply evaluate endometrial proliferation by ultrasound using stripe thickness as a surrogate for a contraceptive effect, an unproven hypothesis. In fact, data from a series of infertile women treated with clomiphene and intrauterine insemination found no difference in pregnancy rates with endometrial stripe thickness of less than 6 mm (15%), 6 to 9 mm (16%), or greater than 9 mm (19%).23
The cervical mucus does become thick and impervious to sperm transport with exposure to a progestin. It is possible that progestational influences on secretion and peristalsis within the fallopian tubes provide additional contraceptive effects. Even if there is some ovarian follicular activity (especially with the lowest-dose estrogen products), these progestin actions serve to ensure good contraceptive efficacy.20
The contraceptive effects of progestin-only products differ depending on dose, potency, and rout of administration. High systemic dose agents such as depot medroxyprogesterone acetate typically block ovulation and suppress ovarian, endometrial and cervical activity. Contraceptive implants have varying effects depending on the progestin and dose; LNG implants rely primarily over years of use on cervical mucus effects although ovulation inhibition can occur initially when hormone levels are highest. Etonogestrel implants, on the other hand, reliably block ovulation and suppress follicle growth significantly, especially during the first 2 years of use. Irregular bleeding may occur as a result of endometrial exposure to variable levels of ovarian estradiol production. Progestin-only pills are less reliable in blocking ovulation and work primarily by blocking development of fertile cervical mucus. The LNG intrauterine system infrequently blocks ovulation, but very high local levels of the progestin result in a thick nonpenetrable cervical mucus (see Chapter 6, Figure 6.2). Although the high level of local hormone also causes an inactive atrophic endometrium related to down-regulation of estrogen receptors, the reality that sperm will not penetrate the mucus means that this endometrial effect is simply a means to induce a desirable “side effect” of decreased bleeding. Cervical mucus during ovulatory cycles of the LNG-IUS during year 7 of use shows modified Insler scores similar to those observed in ovulatory women using a copper IUD; but many of these ovulations appear dysfunctional.24 These data suggest that cervical mucus changes may not provide the only contraceptive effect of the LNG-IUS with extended use in women with ovulatory cycles. However, other mechanisms common to inert IUDs and lower levels of LNG likely prevent fertilization in most cases, making an endometrial contragestive effect the least likely mechanism.25
The Hormonal Contraception Story
Although early experiments demonstrated the potential of hormonal contraception, the use of products derived from animal organs resulted in tremendous variability of response. Even after the chemical structure of
sex steroids was elucidated, extraction and isolation of a few milligrams required starting points measured in gallons of urine or thousands of pounds of organs. Edward Doisy, an American biochemist who first discovered SHs (estrone in 1929), reportedly processed 80,000 sow ovaries to produce 12 mg of estradiol.26
sex steroids was elucidated, extraction and isolation of a few milligrams required starting points measured in gallons of urine or thousands of pounds of organs. Edward Doisy, an American biochemist who first discovered SHs (estrone in 1929), reportedly processed 80,000 sow ovaries to produce 12 mg of estradiol.26
Marker Degradation and Progesterone
The supply problem was solved by Russell E. Marker, an American chemist known for developing the system of octane rating of gasoline. In September 1935, Marker worked at Penn State with support mainly from research grants from the Parke-Davis pharmaceutical company.
At that time, it required the ovaries from 2,500 pregnant pigs to produce 1 mg of progesterone. For several years, Marker worked on extracting hormones from the urine from pregnant animals as he pursued the goal of an abundant and inexpensive supply of progesterone. As these efforts proved futile, Marker became convinced that the solution to the problem of obtaining large quantities of SHs was in plants that contain large amounts of the plant steroid diosgenin, a sapogenin. In 1939, Marker had figured out a method, now called the Marker degradation, to convert a sapogenin molecule into a progestin, but he still needed raw materials. Marker discovered that the roots of the Dioscorea plant (a wild Mexican yam) provided a rich source of sapogenins. Marker was unable to obtain support from the pharmaceutical industry so he used half of his life savings to return to Mexico in October 1942 to collect yams and used these to synthesize 3 kg of progesterone, the largest lot of progesterone ever produced. United States pharmaceutical companies still refused to back Marker, and even his university refused to patent the process. Marker found a Mexican hormone laboratory headed by a Hungarian lawyer, Emeric Somlo, and a German chemist, Frederick A. Lehman; the three men agreed to form a Mexican hormone production company, named Syntex (from synthesis and Mexico). Marker left Syntex in May 1945 after he learned that he was not being paid in accordance with their agreement. He started a new company called Botanica-Mex and changed to a different species of yam (Dioscorea barbasco), which gave a greater yield of diosgenin, and the price of progesterone dropped significantly.27,28
By the 1960s, several pharmaceutical companies operated competing root-gathering operations in Mexico, closely regulated by the Mexican government that imposed annual quotas to balance harvesting with the new annual growth. Mexican yams provided the starting material for the manufacture of synthetic steroids used in oral contraceptives for about 15 years, giving way to other sources, such as soya beans, methods for total synthesis, or microbial fermentation.29
The Synthetic Progestational Drugs, Norethindrone and Norethynodrel
When Marker left Syntex, he took his know-how with him. Fortunately for Syntex, there still was no patent on his discoveries. George Rosenkranz left
his native Hungary to study chemistry in Switzerland under the renowned steroid chemist Nobel Laureate Leopold Ruzicka.30 Rosenkranz emigrated from Europe to Cuba to escape the Nazi regime. In 1945, he was invited for an interview with Syntex after Marker had left and was hired on the spot. Rosenkranz gave up on reconstructing Marker’s process and developed his own commercial manufacture of progesterone and testosterone from Mexican yams. Soon, Syntex was making large profits providing the sex hormones as raw material to other pharmaceutical companies. Rosenkranz now had a large active laboratory and invited a young Bulgarian chemist, Carl Djerassi, to head a research group to concentrate on the synthesis of cortisone; Djerassi accepted the position and moved to Mexico City in the fall of 1949. Djerassi had also fled the Nazi regime, emigrating to the United States in 1939 when he was 16 years old.
his native Hungary to study chemistry in Switzerland under the renowned steroid chemist Nobel Laureate Leopold Ruzicka.30 Rosenkranz emigrated from Europe to Cuba to escape the Nazi regime. In 1945, he was invited for an interview with Syntex after Marker had left and was hired on the spot. Rosenkranz gave up on reconstructing Marker’s process and developed his own commercial manufacture of progesterone and testosterone from Mexican yams. Soon, Syntex was making large profits providing the sex hormones as raw material to other pharmaceutical companies. Rosenkranz now had a large active laboratory and invited a young Bulgarian chemist, Carl Djerassi, to head a research group to concentrate on the synthesis of cortisone; Djerassi accepted the position and moved to Mexico City in the fall of 1949. Djerassi had also fled the Nazi regime, emigrating to the United States in 1939 when he was 16 years old.
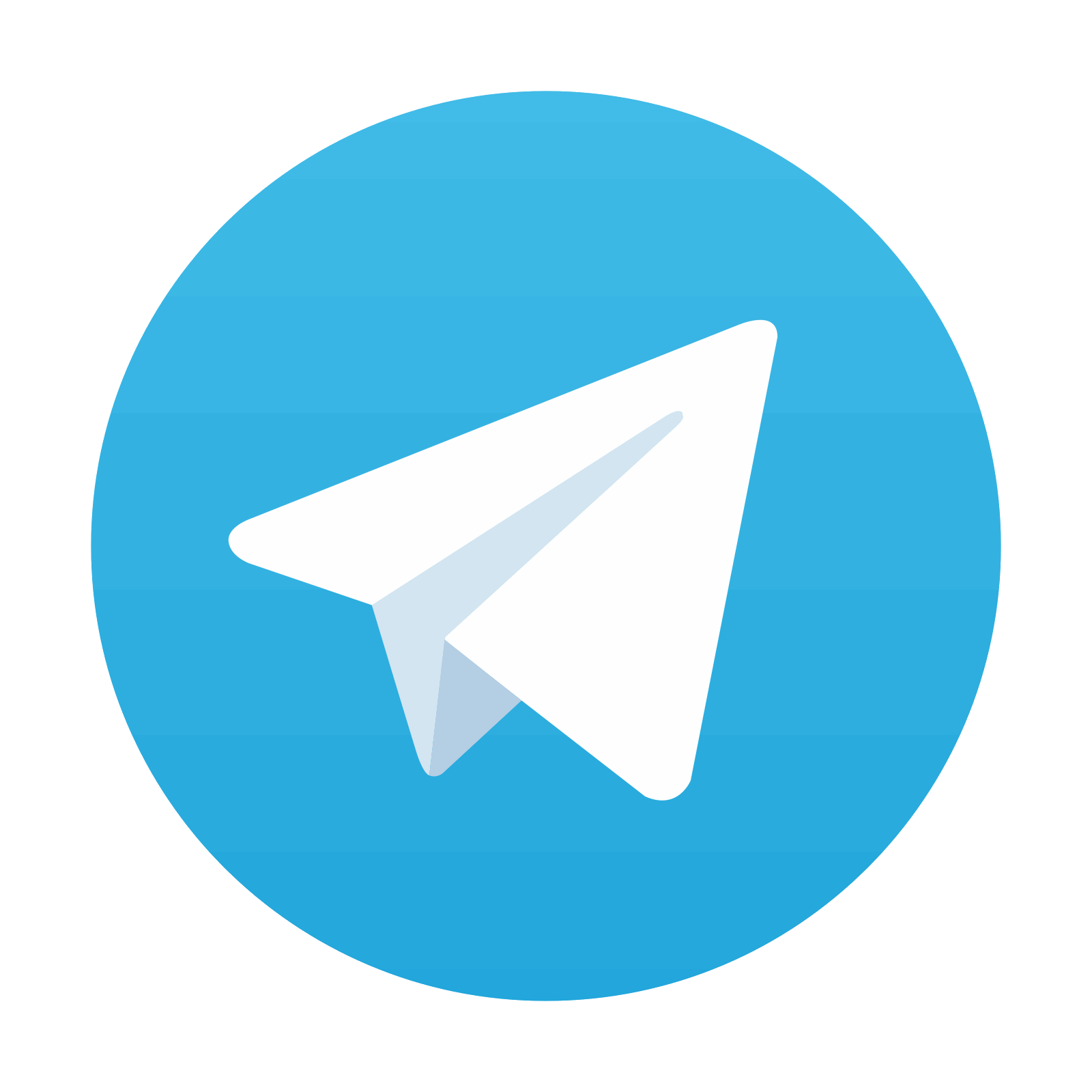
Stay updated, free articles. Join our Telegram channel

Full access? Get Clinical Tree
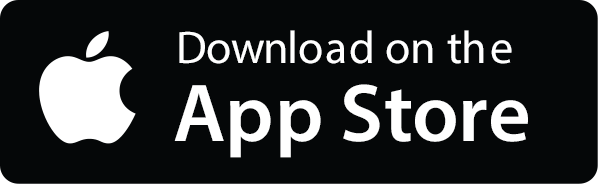
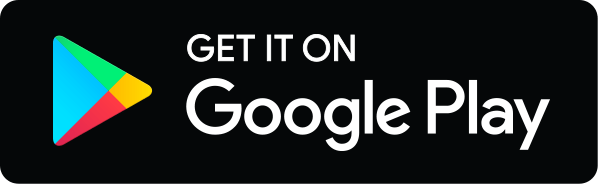