Renal and Genitourinary Diseases
Billy S. Arant Jr.
In most newborns, the kidneys are able to maintain body fluid homeostasis within certain limits. However, these organs may be inadequate to support extraordinary demands placed on them by disease, by the treatment of disease, or by the misinterpretation of normal responses to extrarenal stimuli. This chapter will discuss structural and functional abnormalities of the renal system of neonates.
NORMAL STRUCTURE AND FUNCTION
Embryogenesis and Morphology
Formation of the human metanephric kidney begins during the fifth week of gestation when the ureteric bud, an ectodermal outgrowth of the Wolffian or mesonephric duct that will develop into the renal collecting system, makes direct contact with the caudal mesenchyma of the nephrogenic cord to induce the formation of glomeruli, proximal and distal tubules, and loops of Henle. Failure of these two distinctly different embryonic tissues to establish intimate contact results in failure of the ipsilateral kidney to form normally. All glomeruli are located within the renal cortex. The first ones formed take their final position in the deep or juxtamedullary region. They contribute most to the function of the developing kidney until nephrogenesis is completed at approximately 34 weeks’ gestation. They are nearly equal in size at 40 weeks’ gestation to mature glomeruli and have the structures of the renal countercurrent multiplier mechanism—long loops of Henle and vasa rectae that extend into the inner medulla and papilla. The final group of glomeruli formed occupies the most superficial position of the subcapsular region of the cortex. They do not exhibit filtration before 34 weeks’ gestation and are only 25% or so of the size of juxtamedullary glomeruli at 40 weeks’ gestation. In contrast to deeper glomeruli, they have short loops of Henle that extend only into the outer medulla without vasa rectae and contribute little to the urinary concentrating mechanism. Nephrogenesis follows a similar pattern after birth if the fetus is born prematurely: The infant born at 26 weeks’ gestation may exhibit continued nephrogenesis for approximately 8 weeks after birth. Differences in morphologic arrangement in the developing human renal cortex before and after the completion of nephrogenesis are illustrated in Fig. 61.1.
Cyclooxygenase-2 (COX-2), but not COX-1, is important, especially in the final phase of nephrogenesis, and prostanoids in general seem essential to normal renal development. Angiotensin II is also an important growth factor. Nutrition is also important. If pregnant rats are provided a protein-restricted
diet, the total complement of nephrons in the offspring’s kidneys is reduced. Some have suggested that prematurity alone may result in less than a full complement of 1 million nephrons in each kidney. Alternatively, postnatal management of a high-risk infant may interfere with the completion of nephrogenesis. For example, indomethacin therapy in the preterm infant may imperil nephrogenesis. A reduced number of nephrons may place the individual at later risk of glomerular hyperfiltration, renal insufficiency, or hypertension.
diet, the total complement of nephrons in the offspring’s kidneys is reduced. Some have suggested that prematurity alone may result in less than a full complement of 1 million nephrons in each kidney. Alternatively, postnatal management of a high-risk infant may interfere with the completion of nephrogenesis. For example, indomethacin therapy in the preterm infant may imperil nephrogenesis. A reduced number of nephrons may place the individual at later risk of glomerular hyperfiltration, renal insufficiency, or hypertension.
Filtration by newly formed nephrons has been observed as early as 9 weeks’ gestation; however, the ureter is patent only from the eleventh week. The transient hydronephrosis during this 2-week interval distends the proximal ureter to give the pelvicaliceal system its characteristic shape. Once the ureter is canalized, urine drains into the urogenital sinus and then into the amniotic sac. The urinary bladder is formed from the lower portion of the allantois and the urogenital sinus. The upper portion of the allantois closes by 32 weeks’ gestation to form a fibrous cord. If the bladder outlet is obstructed, the allantois may persist as a patent urachus. The fetal ureter is composed of connective tissue stroma lined by epithelium. The smooth muscle layers that allow the ureter to constrict and shorten during peristalsis and the elastic fibers that permit the ureter to resume its normal caliber and shape are formed between 36 and 48 weeks of gestation. This is complete by the second postnatal month in infants born at term.
The only recognized role of the kidney essential to normal fetal development is its contribution to amniotic fluid volume. Mineral and fluid balance and the excretory functions of the kidney are handled by the placenta and maternal kidney. Failure of urine formation or urinary obstruction results in oligohydramnios and may cause fetal compression. Oligohydramnios before 20 weeks’ gestation may be associated with pulmonary hypoplasia.
Glomerular Filtration Rate
The glomerular filtration rate (GFR) has been measured at birth in healthy human neonates at 24 weeks’ gestation and has been found to be approximately 0.2 mL/minute, 0.5 mL/minute/kg, or 5 mL/minute/1.73 m2. Such a low GFR in a child or adult would represent chronic renal insufficiency; therefore, not surprisingly, the neonatal kidney has been mislabeled as functionally immature. Although growth and development of the kidneys continue throughout gestation, the GFR changes little, if at all, before a postmenstrual (gestational plus postnatal) age of 34 weeks, when nephrogenesis is complete. Understanding that the neonate born at 26 weeks’ gestation will not exhibit any appreciable change in the GFR until nearly 8 weeks of age (34 weeks after conception) is clinically relevant. In contrast, when birth occurs after 34 weeks’ gestation, the GFR increases two- to threefold during the first week of life, just as in full-term infants.
The same developmental pattern determines whether infants are studied at birth at a particular gestational age or are studied after birth at the corresponding postmenstrual age (Fig. 61.2). A similar developmental pattern of GFR has been observed in all mammalian kidneys studied (Box 61.1).
Developmental increases in the GFR continue until the average normal adult GFR of 125 mL/minute is attained during adolescence, when linear growth ceases and the epiphyses close. The adult or mature value of the GFR corrected for body surface area (125 mL/minute/1.73 m2) is observed rarely before 6 months of age and sometimes only at 12 months of age in infants born at term. During this period the rate of increase in GFR exceeds the rate of body growth.
Measuring the GFR in neonates is tedious and difficult when accurately timed and complete collections of urine are required; small errors in collection can produce large errors in calculating the GFR (Box 61.2).
Urinary Volume
Every normal neonate, as well as most with congenital renal abnormalities, will void some urine within 48 hours of birth. Urinary flow rate is, at best, only an indirect measurement of overall renal function. However, because an exact measurement of the GFR in neonates is impractical, clinicians have adopted urinary volume (UV) (mL/hour/kg) as a convenient, although uninformed, indication of “renal function.” The volume of urine formed in any given period depends not only
on the GFR but also on tubular reabsorption. The final UV will be minimal and relatively concentrated during hydropenic conditions when the kidney attempts to conserve or restore extracellular fluid volume (ECFV). It is large and dilute when ECFV is excessive or in the presence of tubular dysfunction, failure of the hypothalamus to produce arginine vasopressin (AVP) (central diabetes insipidus), or failure of the collecting duct epithelium to respond to circulating AVP (nephrogenic diabetes insipidus).
on the GFR but also on tubular reabsorption. The final UV will be minimal and relatively concentrated during hydropenic conditions when the kidney attempts to conserve or restore extracellular fluid volume (ECFV). It is large and dilute when ECFV is excessive or in the presence of tubular dysfunction, failure of the hypothalamus to produce arginine vasopressin (AVP) (central diabetes insipidus), or failure of the collecting duct epithelium to respond to circulating AVP (nephrogenic diabetes insipidus).
BOX 61.1 Glomerular Filtration Rate in Developing Mammalian Kidneys
The glomerular filtration rate remains relatively constant until nephrogenesis is complete, before term birth in humans and sheep and after birth in the rat and dog. The biologic regulation of these changes is understood poorly, but because they can occur abruptly, attributing them simply to a process of maturation seems inadequate. More likely, a vasoactive mechanism is responsible; increments in renal blood flow are related inversely to changes in renal vascular resistance, plasma renin activity, circulating levels of angiotensin II, renal synthesis of vasodilator prostaglandins, and, probably, nitric oxide. Renal vascular resistance and cortical blood flow can be changed acutely by one or more of these vasoactive substances.
BOX 61.2 Measuring the Glomerular Filtration Rate in Neonates
Developmental changes in the glomerular filtration rate (GFR) can be monitored satisfactorily in most neonates from serial measurements of serum creatinine concentration (SCr). For neonates at high risk, SCr should be measured on the first day of life to allow comparison with subsequent measurements. Creatinine is a product of normal muscle metabolism and is a small molecule that crosses all water-permeable membranes, including the placenta; therefore, SCr levels in the mother and her fetus are similar. At birth, no correlation exists between SCr and the gestational age of the infant. Hence, a single measurement of SCr at birth has no particular value for estimating the GFR. When the GFR increases rapidly in infants born after 34 weeks’ gestation, the SCr is reduced by approximately 50% at the end of the first week of life and usually reaches a stable normal value of 0.25 to 0.40 mg/dL during the second week. In contrast, the GFR does not increase appreciably during the first week of life in infants born before 34 weeks’ gestation, and the SCr actually may increase slightly during the first 48 hours after birth as a result of hemoconcentration and ongoing creatinine production of approximately 8 mg/kg/day. Only minor changes in the SCr can be expected during the first week of life in normal preterm infants. When a conceptional age of 34 weeks is reached, however, the GFR increases, and the SCr falls by approximately one-half within a few days, just as in more mature neonates during the first week of life.
GFR corrected for body surface area may also be measured from the formulas derived for premature infants or for full-term infants. The formula for both is GFR (mL/minute/1.73 m2) = k × body length (cm)/SCr(mg/dL); for preterm infants, k = 0.35, and for full-term infants after the first week of life, k = 0.45. Normal values for the GFR for a particular postmenstrual age are available in specialty texts and the literature.
For example, the GFR in the fetus is relatively low (≤30 mL/hour/kg), but the UV is high (10 mL/hour/kg). Tubular reabsorption in the fetal kidney is inefficient; it is not necessary for it to be otherwise. Production of amniotic fluid is essential. The positive fluid balance or relative excess of ECFV that is normal for the fetus is assured by placental transfer from the expanded ECFV of the mother. Diuresis in utero is maintained, in part, by altered peritubular forces that reduce proximal tubular reabsorption, by minimal circulating AVP, by fewer aquaporin channels in the principal cells of the collecting duct, and by increased renal synthesis of prostacyclin and prostaglandin E2 (PGE2). The diuresis is interrupted briefly during labor with the normal release of AVP, but is resumed after birth as AVP levels decrease again. The duration of diuresis in a normal neonate is determined by the degree of ECFV excess, by hormonal interactions, and by hemodynamic changes. Because the ECFV in the preterm infant is greater than that in the full-term infant, the postnatal diuresis and relative weight loss after birth is greater.
The normal pattern of changes in urine production begins with a rate of 10 mL/hour/kg in the fetus, at least after 30 weeks’ gestation. Output decreases to less than 0.5 mL/hour/kg during parturition and then increases again to 1 to 8 mL/hour/kg, depending on the gestational age of the neonate, until the excess extracellular fluid for that infant is excreted. Thereafter, the UV in the neonate given appropriate fluid intake remains between 0.3 and 2.0 mL/hour/kg, with urinary osmolarity between 50 and 800 mOsm/L or specific gravity of 1.001 to 1.024. AVP acts on specific receptors to increase the number of aquaporin-2 water channels in the apical membrane and aquaporin-4 channels in the basolateral membrane of collecting duct epithelial cells.
High-risk neonates of every gestational age usually are treated from birth with parenteral fluids. Clinical decisions for changing the volume of the fluids given or for prescribing diuretic therapy usually are based on UV instead of changes in body weight, urinary osmolarity, or fractional sodium excretion. Because hemodynamic and hormonal changes may not be the same in every neonate, urine production will vary with factors other than the GFR. For example, before 34 weeks’ gestation, the premature neonate excretes approximately 10% of glomerular filtrate as urine. Although the GFR increases after 34 weeks’ gestation, tubular reabsorption increases as well, so that by 38 weeks, less than 3% of glomerular filtrate escapes the nephron.
In general, the higher the urinary flow rate (mL/hour/kg) is, the lower the fraction is of glomerular filtrate reabsorbed, not only water but also sodium chloride (NaCl), bicarbonate, amino acids, phosphate, calcium, and glucose. The fractional excretion of glomerular filtrate can be calculated as urinary flow rate (V) divided by GFR (V/GFR) expressed in the same units. In other words, when the urinary flow rate in a very preterm infant weighing 1.0 kg is 3 mL/hour/kg, approximately 10% of glomerular filtrate is not reabsorbed (V/GFR = 0.05 mL/minute ÷ 0.5 mL/minute = 0.10, or 10%). If this same infant were to excrete only 1% of glomerular filtrate, as does the normal adult, the UV would be only 0.3 mL/hour/kg.
The physiologic basis for or clinical relevance of maintaining UV at 1 mL/hour/kg or greater in every infant has not been documented; however, rarely would any physician delay in prescribing additional fluid therapy or diuretic agents in a neonate whose UV decreases below 1 mL/hour/kg. The importance of maintaining UV is to ensure that sufficient water is present in tubular fluid to permit excretion of the solute load imposed on the kidney. If no solute load is present in infants who are conserving NaCl and are given only dextrose in water to replace insensible water losses immediately after birth, no UV is required. When, in the past, feedings were withheld routinely from preterm infants to prevent aspiration, some infants produced no urine for up to 5 days, with no evidence of renal injury. Moreover, acute renal injury was not obvious in neonates trapped during the Mexico City earthquake who survived longer without fluid than did older victims.
Other factors that may cause a decrease in UV include unrecognized heart failure, shunting of cardiac output away from the renal circulation through a patent ductus arteriosus, and changes in intrathoracic pressure from pneumothorax or mechanical ventilation, either of which can reduce cardiac output and stimulate baroreceptor-mediated release of AVP. In each case, the neonate’s condition actually may be worsened by additional fluid therapy or furosemide administration.
Renal Handling of Sodium Chloride
The developing kidney has been characterized as inadequate to maintain NaCl balance, but the developing kidney is limited only when extraordinary demands are imposed on it. Some immature preterm infants have survived (and more will survive in the future) with little or no clinical intervention. They exhibit neither NaCl wasting nor any other tubular dysfunction. Renal tubular function in newborn infants is qualitatively similar to that in adults. When the function of the adult kidney is assessed during chronic expansion of ECFV with saline, it manifests hypotonic saline diuresis, glucosuria, hyperphosphaturia, hypercalciuria, hyperuricuria, and increased excretion of bicarbonate, just as in the human fetus and preterm infant with expanded ECFV. What is interpreted as an inappropriate or immature response by the neonatal kidney is the normal response of the mammalian kidney to changes in effective arterial blood volume (EABV). Baroreceptors in the arterial circulation sense EABV as a combined force of blood volume and blood pressure. When EABV falls because of decreased cardiac output (decreased blood volume) or decreased peripheral resistance (vasodilation), the neonatal kidney responds by conserving volume. The results are oliguria or anuria, NaCl retention, and hypertonic urine. Because this response can follow a change in blood pressure, blood volume, or both, it may be observed when total body water is increased or decreased and when blood pressure is high, normal, or low. Some of the mechanisms that maintain or restore EABV are depicted in Fig. 61.3. Other mechanisms and, in particular, the direct actions of nitric oxide on EABV have not been elucidated fully.
During fetal development, body composition changes dramatically. The content of body water decreases from 96% of body weight at 8 weeks’ gestation to less than 80% at 40 weeks’ gestation, as the NaCl content decreases from 120 mEq/kg body weight to 80 mEq/kg body weight. This parallel reduction in total body water and NaCl accounts for the decrease in ECFV from 60% to 40% of fetal body weight during the last trimester of pregnancy. After birth, the ECFV is reduced further from 40% to less than 30% of body weight. This developmental decrease in relative ECFV occurs as body tissues expand with an increase in the absolute quantity of ECFV and as a constant hypotonic saline diuresis maintains amniotic fluid volume.
![]() FIGURE 61.3. Schematic representation of the physiologic response to a decrease in effective arterial blood volume. |
When infants are born prematurely, a variable period of high urinary volume and negative NaCl balance occurs postnatally in an apparent effort by the neonate to reduce its ECFV, as would have occurred had the fetus remained in utero until term. However, before the kidney of the preterm neonate can respond by conserving NaCl and water in a manner similar to that of the full-term infant, the maturational differences in ECFV and EABV between premature and mature neonates must be resolved.
Because neonates, regardless of their gestational age at birth, vary somewhat in the exact timing of renal responses to reduced ECFV, each infant must be observed closely to determine when this adaptation has been attained. The best clinical estimate of the timing is provided by changes in urinary volume and excretion of NaCl. The fractional excretion of sodium (FENa) can be calculated from the sodium and creatinine concentrations in random samples of plasma and urine. These values are substituted in the following formula: FENa (%) = urine sodium concentration × plasma creatinine concentration × 100/urine creatinine concentration × plasma sodium concentration. The relatively large volume of urine produced by the fetal kidney before birth and by the preterm neonate immediately after birth contains higher NaCl concentrations (greater than 50 mEq/L) than that of the full-term infant (less than 20 mEq/L); FENa is 12% to 15% in the fetus, more than 1% to 7% in the preterm infant, and less than 1% (usually less than 0.5%) in the full-term infant.
The continued hypotonic saline diuresis after birth results in a greater fractional loss of birth weight in preterm infants (10% to 30%) than in full-term infants (3% to 8%). When the excess ECFV has been excreted, further loss of body weight is determined by insensible water losses. This is accompanied by a second period of oliguria or anuria, with urinary volume less than 1 mL/hour/kg, urinary osmolarity greater than 300 mOsm/L, and FENa less than 1%. This occurs on the first day of life in infants born at term and 3 to 7 days after birth in those born prematurely. If only insensible water losses, not urinary volume, are replaced with dextrose in water before this pivotal point in transitional physiology, subsequent oral or parenteral fluid therapy should replace both urinary volume and insensible water losses. Then, body weight is maintained and weight gain is related to caloric intake and growth.
Although UV increases and urinary osmolarity decreases on this regimen, no increase in urinary NaCl excretion should occur. However, if fluid therapy has replaced urinary volume from birth (greater than or equal to 100 mL/kg/day), the relative excess ECFV of the fetus will be perpetuated, and the hypotonic saline diuresis will continue indefinitely, preventing the creation of a positive NaCl balance, regardless of the amount of NaCl provided. When urinary volume, but not urinary NaCl, is replaced by 5% to 10% dextrose in water, hyponatremia will develop, and clinical evaluation will suggest renal NaCl wasting, the so-called immaturity of neonatal renal tubular function.
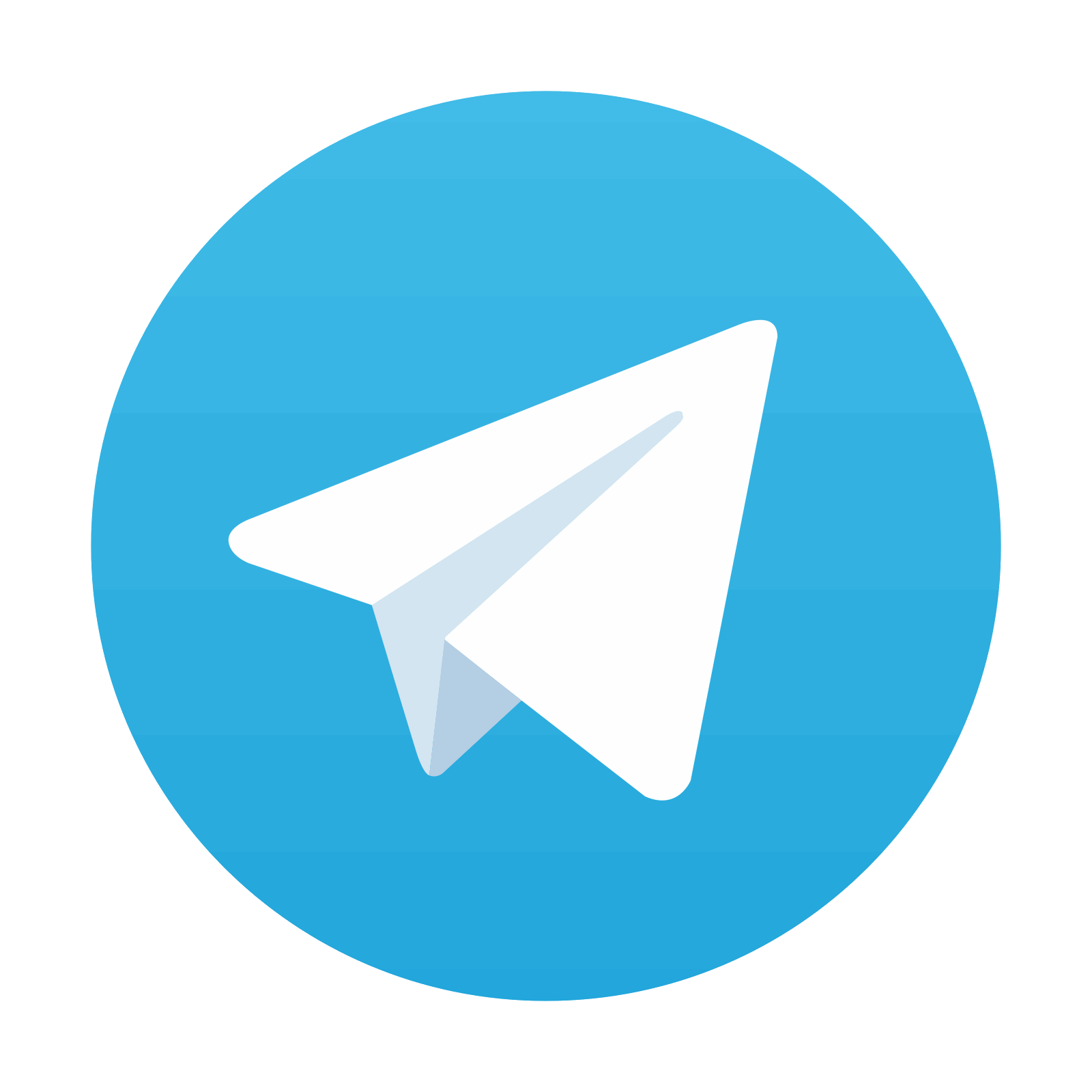
Stay updated, free articles. Join our Telegram channel

Full access? Get Clinical Tree
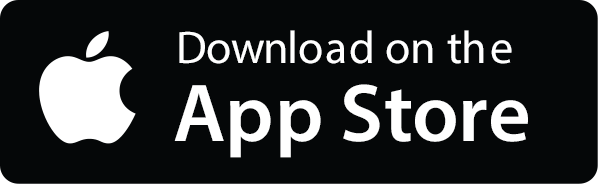
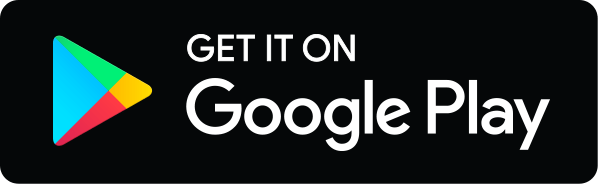