CHAPTER 11 Reconstructive strategies for recovery of hand function
Summary box
Introduction
Neonatal brachial plexus palsy (NBPP) has a frequency of 0.63 to 2.6 per 1000 live births.1,2 The incidence of NBPP has remained relatively stable despite awareness of the problem and improved obstetric techniques. Risk factors that may predispose to NBPP include macrosomia, multiparous pregnancies, previous deliveries resulting in NBPP, prolonged labor, breech delivery, assisted delivery (vacuum or forceps), cephalo-pelvic disproportion, gestational diabetes, and shoulder dystocia.3,4 The most common form of NBPP involves the upper trunk roots (C5,C6) (Erb palsy) (Figure 11.1), occasionally in combination with an injury to the C7 root.5,6 Less often, the entire plexus is involved (C5-T1) (Figure 11.2), and rarely, only the lower trunk roots (C8T1) (Klumpke palsy) (Figure 11.3) are affected.7
The severity of the NBPP can range from a neurapraxia type of injury, which may resolve without any deficit within three weeks, to an avulsion of the nerve root that has no potential for spontaneous recovery. Narakas and others have attempted to grade NBPP into four groups with each succeeding group representing a more severe injury.5,6 Group 1 infants have involvement of the C5 and C6 roots and represent the classic Erb palsy. The important muscles that derive their innervation solely from the C5 and C6 roots include abductors and external rotators of the shoulder (supraspinatus, infraspinatus, and deltoid), flexors of the elbow (biceps brachii, brachialis, and coracobrachialis), and the supinator muscle. Therefore infants with Erb palsy lack shoulder abduction and external rotation, elbow flexion, and forearm supination. However they have good hand and wrist function. (Figure 11.1).
Group 2 includes infants that have involvement of the C7 root in addition to C5 and C6. This leads to paralysis of the subscapularis, teres major, clavicular fibers of the pectoralis major, brachioradialis (BR), extensor carpi radialis longus (ECRL) and brevis (ECRB), and occasionally the extensor carpi ulnaris (ECU) and extensor digitorum communis (EDC). These infants have good flexion of the wrist and digits, but lack extension. This combined with the impairment caused by the C5 and C6 palsy leads to the classic ‘waiter’s tip’ posture. Group 3 infants have involvement of all roots (C5–T1) and present with a flail extremity (Fig. 11.2). Group 4 infants have in addition to involvement of all roots, injury to the second order preganglionic sympathetic fibers. These fibers exit the spinal cord at the level of T1, and enter the cervical sympathetic chain, where they are in close proximity to the lower roots of the brachial plexus (C8, T1). This results in a constellation of signs (ptosis, myosis, enophthalmos, and anhydrosis) on the ipsilateral side of the face better known as Horner syndrome after Johann Friedrich Horner, the Swiss ophthalmologist who first described the syndrome in 18698 (Figure 11.4). Groups 1 and 2 account for 73%, whereas Groups 3 and 4 account for 20–25% of all NBPP.9
Unlike adult palsy, children with NBPP do not have neuropathic pain.10 The absence of pain when the injury is distal to the dorsal ganglion has been attributed to the delayed maturation of peripheral nerves, especially at the nodes of Ranvier. Conduction velocity studies in human subjects have shown that sensory and motor conduction in the median nerve is <50% of normal values at birth, progressing to normal values at ~2 years of age. Chronic neuropathic pain syndromes are not apparent in humans before nerve conduction velocities approach adult values. The absence of long-term central pain of a nerve root avulsion injury in children is believed to be due to the excellent plasticity of the central nervous system (CNS) in infants. The change of limb dominance, where the injured side becomes non-dominant and the excellent recovery of sensation in NBPP has also been attributed to this CNS plasticity.10,11,12 Long term follow-up of children with NBPP has shown that there is a marked difference between motor and sensory function. Sensory neurons have a better survival than motor neurons and are less dependent on trophic factors from target cells for survival. In the young, sensory neurons always project to several spinal segments, in contrast to motor neurons, which are restricted to 2–3 segments.11,12 In general, sensory disturbance of the forearm and hand does not seem to be a significant problem in most children with late NBPP. However, there have been reports of self mutilation13,14 and the denervated limb is theoretically at risk of contact burns caused by decreased sensibility and poor motor control.15
Forearm and hand problems are seen with involvement of the middle and lower roots (C7–T1), and the nature of the deformity corresponds to the child’s age at presentation. Children who present for treatment in infancy (infant or early NBPP) have flail hands and wrists, which are functionally and cosmetically disruptive. They are suitable for microsurgical nerve reconstruction surgery, which should be done early to maximize functional return to the hand.16 The other group of children presents later in life with sequelae (sequelae or late NBPP with deformity).17 They are no longer suitable for nerve reconstruction procedures and treatment consists of secondary procedures to improve or enhance shoulder, elbow, forearm, and hand function. The options for secondary procedures has remained relatively unchanged over time, and consists essentially of soft tissue releases, corrective osteotomies, tendon transfers, joint fusions, and free muscle transfers. Rehabilitation and physical therapy treatment are critical after these secondary procedures.
Pathogenesis
NBPP is not a static process. As the child grows up, a variety of secondary deformities of the extremities occur. The extent and severity of these secondary deformities is related to the severity of the initial plexus lesion, the age of the child at presentation, and the degree of recovery of muscle function. They occur not only due to the brachial plexus lesion, but can occur due to lesions of the osteoarticular system (epiphyseolysis of proximal humerus, anterior subcoracoid dislocation of the shoulder etc.) and/or lesions of the muscular system (fibrosis of the shoulder girdle muscles, biceps etc. following birth trauma). Other factors that can aggravate these deformities include (1) Incorrect initial treatment by forced manipulation to correct contractures, (2) Plaster casts or splints in exaggerated position of abduction and external rotation (’Statue of Liberty’ position), and (3) Delay in surgical correction of muscular contractures leading to articular deformity and joint displacement.18
The recovery of muscle function also contributes to the development of secondary deformities. Three types of muscle impairment can be seen after recovery – paresis (incomplete recovery with weak motor strength), paralysis (no recovery with atrophy), and co-contraction (simultaneous contraction of different muscles – coactivation by simultaneous nerve input). Co-contractions can cause functional impairment, if these muscles are antagonistic. Although paresis and palsy are seen both in the adult and obstetric plexus lesion, co-contraction is a problem limited to late NBPP. The exact cause of co-contractions is still being debated. Chuang et al. believe that co-contractions are a result of aberrant reinnervation (or cross-innervation).17,19 This occurs when regenerating axons become misdirected and ultimately innervate a muscle other than their appropriate target. This causes abnormal multiple muscle contractions (co-contractions), thus antagonizing the intended action (Figure 11.5). Over time, the stronger co-contracting muscle becomes hypertrophic, and the muscle imbalance in a growing child leads to joint contractures, bony deformity and joint displacement.
In NBPP, the phenomenon of aberrant reinnervation is seen with ruptures of the upper roots (C5, C6, and/or C7). Thus co-contraction commonly impairs shoulder and elbow function. It is less noteworthy in the forearm and hand, because forearm and hand deformities result from injury to the lower roots (C8 and T1), which are more likely to be avulsed rather than ruptured.19 Chuang et al. have described four types of co-contractions in NBPP: (1) Co-contraction between shoulder abductors and adductors; (2) Co-contraction between elbow flexors and extensors (Figure 11.6); (3) Co-contraction between elbow flexors and shoulder abductors (Figure 11.5); and (4) Co-contraction in shoulder, elbow, forearm, and hand.20 Evidence in animal studies has shown that nerve regeneration differs between adults and infants and that infants’ axons are more likely to split, thus innervating different muscle groups and leading to co-contraction.21
Hentz feels that co-contractures are a learned response that can be unlearned. For example., the typical co-contracture of deltoid and biceps when the child tries to place his or her hand to the mouth (the so-called trumpet sign) occurs because the child lacking in good external rotation, ‘‘learns’’ that if he or she is to reach the mouth with the hand, the arm must be brought away from the body before the elbow is flexed (Figure 11.5). Otherwise, lacking external rotation, the chest blocks the hand’s ability to reach the mouth.22 Some believe cortical co-activation (coalescence of neighboring fields in the motor cortex) is responsible for co-contraction.23 Others feel that the aberrant re-innervation is also responsible for bad sensory feedback that could affect the central motor programming. Interrupting this feedback loop by the injection of botulinum toxin allows the muscle to recover and the child’s neuronal plasticity maintains the improvement in range of motion after the effect of botulinum toxin wears out.24
Although the shoulder is the most frequently affected joint in NBPP, the elbow, forearm, wrist, and hand also display disabling deformities. The most common sequelae in late cases are a limb length discrepancy, which is accentuated by flexion contracture of the elbow (Figure 11.7). In a relatively large series with 148 patients, Zancolli et al. indicated that the typical clinical picture includes internal rotation contracture of the shoulder (72%), flexion contracture of the elbow (62%), supination contracture of the forearm (69%), pronation contracture of the forearm (29%), ulnar deviation of the wrist (27%), and varying types of finger paralysis.18
Elbow joint
In general, deformities around the elbow are usually not as severe as those around the shoulder. The common problems seen around the elbow joint are flexion contracture (62%) (Figure 11.8), weakness of extension (46%), and weakness of flexion (18%).18 Rarely the child may present with dislocation of the proximal end of ulna or the radial head. Although weakness of extension or flexion can be explained by paresis, palsy or poor recovery of the triceps and biceps respectively, the pathophysiology of the elbow flexion contracture is poorly understood.
The paradox of development of an elbow flexion contracture in a child with a weak biceps was investigated by Aitken, who demonstrated the development of proximal ulnar curvature, radial neck clubbing, posterior subluxation of the radial head, radial head dislocation, and dislocation of both the ulna and the radius. He felt that it resulted from a combination of muscle imbalance and rigid splinting.25 Al-Quattan has noted that this deformity is often associated with a shoulder internal rotation contracture. He believes that these children stand with the shoulder slightly abducted (and hence the elbow falls into slight flexion) to place the limb away from the body in a better functional position (Figure 11.9). This constant flexed posture of the elbow contributes to the elbow flexion contracture.26 In the child with a total palsy who has recovered C5,C6 function, the flexion contracture is due to recovery of the biceps muscle in the presence of a weak or absent triceps. In addition, the brachialis may be hypertrophic following aberrant reinnervation. The unopposed muscular activity of the biceps along with the brachialis creates a progressive elbow flexion and supination contracture.27 However, this contracture can occur even in children with triceps function. In these cases it is believed to be due to insidious fibrosis of the biceps following trauma during delivery.22
Forearm
The late sequela of NBPP manifests in the forearm either as a supination contracture or a pronation contracture. In Zancolli’s series, supination contractures (69%) occurred more frequently compared to pronation contractures (28%).18 These contractures result from an imbalance between supinator muscles, namely biceps (C5,6) and supinator (C5,6) and the pronator muscles, namely pronator teres (C6,7) and pronator quadratus (C7,8T1).18,22,27
A supination deformity results from unopposed action of the supinators in the presence of paralytic pronators. It is seen in NBPP Groups 2, 3 and 4, who have had recovery of their C5,6 roots. Initially passive correction of the supination deformity is possible; however, as the child’s skeleton grows, the deformity becomes fixed because of the contracture of the interosseous membrane (supination contracture-fixed deformity). Continued growth in the presence of a contracted interosseous membrane leads to incurving of the forearm bones with bowing of the radius and ulna and possible volar subluxation/ dislocation of the distal end of ulna or head of the radius (Figure 11.10).18 The supination deformity causes a functional as well as a cosmetic disability. They are unable to perform many activities of daily living (eating, writing, and bimanual activities) that require simultaneous elbow flexion and pronation. Al-Quattan has termed this deformity the ‘beggar’s posture,’ and when it involves the right hand as the ‘unshakeable hand’.28 Children with a supination deformity compensate with flexion and internal rotation of the shoulder. This maneuver may be difficult for a child with a weak or unstable shoulder.
A pronation deformity results from unopposed action of the pronators in the presence of paralytic supinators. It is seen in NBPP Group 1 (Erb’s palsy) children, who have had none or partial recovery of their C5,6 roots. It has also been noted in total plexus injury, and in these cases, it is believed to be due to muscle imbalance during the recovery phase.29 With growth, an untreated pronation deformity may lead to a pronation contracture (Figure 11.11), and possible deformities of the radius and ulna, including dorsal dislocation of the distal ulna and posterior dislocation of the radial head. In addition to their unattractive appearance, a pronation deformity affects the ability to carry out basic hand functions needing supination, such as carrying objects, buttoning clothes, and perineal care.29
Wrist and hand
Chuang et al. reported a series of 54 children with late NBPP who underwent reconstructive procedures to improve forearm and hand function.30 In their experience, the common hand and wrist problems were extension deficit at the metacarpophalangeal joint (MCPJ)(60%), thumb instability (37%), ulnar deviation of the wrist (19%), extension deficit at the wrist (15%), digital flexion deficit (15%), and interphalangeal joint (IPJ) extension deficit (13%). The pathogenesis of most wrist and hand deformities is relatively straightforward. The ulnar deviation of the wrist results from muscle imbalance produced by paralysis of the ECRB and ECRL(C6,7), flexor carpi radialis (FCR) (C6,7) in relation to the unopposed activity of the ECU(C7,8), and the flexor carpi ulnaris (FCU) (C8T1) (Figure 11.12).18,30 This ulnar deviation of the wrist is a flexible deformity and was frequently associated (27%) with supination contractures in Zancolli’s series.18 The thumb instability is caused by lack of an abductor pollicis brevis (APB) (C6,7,8T1), abductor pollicis longus (APL) (C7,8), and extensor pollicis brevis (EPB) or extensor pollicis longus (EPL) (C7,8).30 Weak or partially absent finger flexion is often observed in total or lower plexus palsy.30 The clinical presentation in the hand varies greatly from child to child and depends on the degree of spontaneous recovery.18
Assessment of elbow and hand function
In the management of the sequela of NBPP, physical examination findings are used to determine the need for surgical intervention. Several classification and scoring systems for assessing function and predicting outcomes have been proposed. However a standard evaluation system has not been established and there is no consensus in literature regarding what constitutes a good or acceptable functional outcome.31
The Medical Research Council (MRC) grading has been the standard for the assessment of motor power (Table 11.1).32 However it cannot be used in children younger than 3 years of age, as testing against resistance is not possible. Also, the MRC grading does not assess the range of motion or overall hand function. Gilbert et al. introduced a modification of the MRC grading system for use in younger children that did not assess grade 4 and 5 (Table 11.1).33 Al-Quattan has modified the MRC grading to include a gross assessment of the range of motion (ROM) in older children (Table 11.1).34 However, his grading is different from the MRC scheme, making it difficult to remember. Bhardwaj et al. have modified the MRC scheme by adding three subgroups (A, B, and C) to grades 2, 3 and 4 respectively (Table 11.1).35 Subgroup A indicates ROM ≤ 50% of normal, subgroup B indicates ROM > 50% of normal, and subgroup C indicates full ROM. The three subgroups for grade 4 are based on percentage of load compared to the normal side that could be carried through the full ROM. We modified Bhardwaj’s modification of the MRC grade for use in older children (Table 11.1). We removed subgroup C, as full ROM in a particular grade would automatically take it to the next grade. In addition, we use ROM subgroups for grade 4 instead of the percentage of load of the normal side, because this enables testing of other muscles and not just the biceps.
The Mallet score, Toronto test score, and the more comprehensive active movement scale were designed to overcome the limitations of the MRC grading system.36–38 They do not require testing against resistance. The Mallet score is used mainly to assess shoulder function and needs a cooperative child. The Toronto test score and the active movement scale can be carried out in young children by appropriate limb positioning (Table 11.2). These scores can be used to quantify pre and post-operative function and to predict recovery in infants with NBPP.31 Although the active movement scale is more comprehensive, a paper comparing them found that the Toronto test score had greater intraobserver and interobserver reliability compared to the active movement score, suggesting that more complex classifications and scoring schemes may have relatively poor reliability within and among examiners.31 We use the Toronto test score in younger children. Chuang et al. has used a ‘score of 10’ in his practice.17 It combines an Erb score (assesses upper plexus) and a Klumpke score (assesses lower plexus). He uses this system to evaluate functional ability and reconstructive options. The score is not useful in pre and post-operative comparisons, but rather is a tool in understanding the sequela of late NBPP and the effect of aberrant re-innervation.17
Although the above scoring systems are useful in grading muscle power and joint ROM, they do not evaluate overall hand function including sensibility and intrinsic function. Four methods of assessment of overall hand function are available, namely the Dubousset classification,39 the Raimondi,40 the modified Raimondi classification,16 and the King Saud University hand function grading34 (Table 11.3). The Dubousset classification is too simple and does not assess intrinsic muscle function, whereas the Raimondi and modified Raimondi classification are too complex and includes evaluation of wrist and forearm function.34 The King Saud University hand function grading is simple, yet maintains adequate detail. In our practice, we do not use any of the above hand function classifications. The clinical presentation in the hand varies greatly from one child to another, and we feel that a useful comparison could not be made based on these grades.
Pre-operative considerations
The treatment of NBPP focuses on early microsurgical reconstruction, because this leads to substantial functional improvement.16,41–46 However, secondary surgery still plays a critical role in improving the function of the upper extremity.47 Secondary surgery consists of muscle and tendon transfers, tenodeses, capsulodesis, corrective osteotomies, and/or arthrodesis.47–50 For secondary reconstructive procedures to be effective, the surgeon must be aware of the natural history of NBPP, the growth of the particular child, as well as the specific functional deficits presented by the child. The reconstructive possibilities available to the surgeon are dependent on the number of available active muscles and the condition of the entire limb. A multidisciplinary approach that includes various specialties, as well as a carefully timed integrated approach to treatment including conservative, primary and secondary treatment modalities, is essential in achieving optimal results. Even when undertaking early microsurgical reconstruction, the secondary reconstructive procedures must be kept in mind, in order to effectively improve the overall functional results.48
Optimum age for secondary reconstruction
Most authors agree that the best age for the reconstruction of late NBPP deformities is at age 4 or older.20,51 These children are easier to evaluate, do not have severe contractures and are able to co-operate in rehabilitation. Chuang et al. feel that the optimal age for shoulder and elbow reconstruction is during the preschool years (4-6 years), whereas the optimum age for forearm and hand reconstruction is during the school going years (6-13 years).30
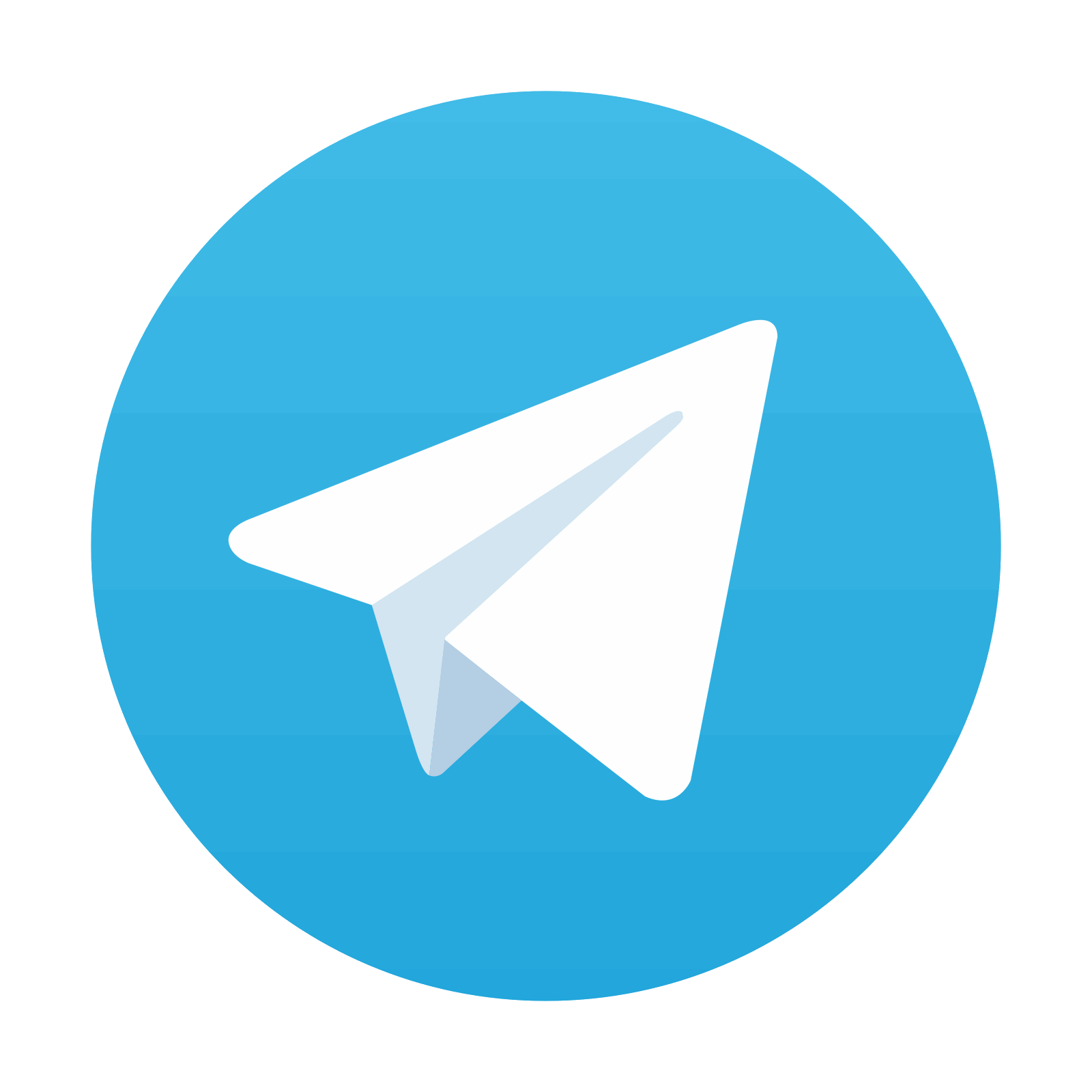
Stay updated, free articles. Join our Telegram channel

Full access? Get Clinical Tree
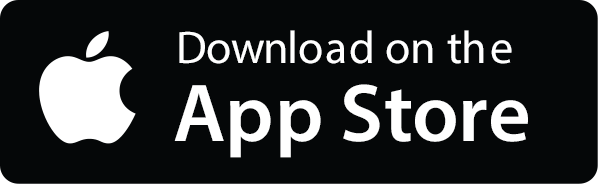
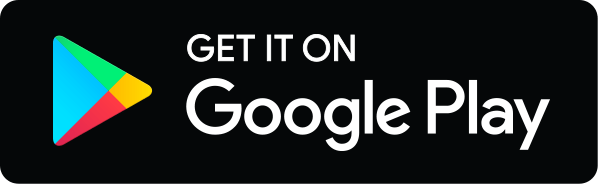