CHEMISTRY, ANIMAL PHARMACOLOGY, AND MECHANISM OF ACTION
Sulthiame (STM) is a sulfonamide derivate, N-(4-sulfamoylphenyl)-1,4-butansultam (Figure 63.1), a crystalline powder, with a molecular weight of 290 g/mol (1).
STM has no antibacterial properties, and although it is a weak carbonic anhydrase inhibitor it has no diuretic activity at therapeutic doses.
35S-STM is well absorbed from the gastrointestinal tract in rats; 80% of the dose of STM is excreted unchanged in the urine during the next 48 hours and only a small amount is found as an inactive hydroxylated derivative in urine (2). STM readily penetrates into tissues and brain and reaches concentrations similar to those found in plasma. STM half-lives in animal studies are variable and range from approximately 4 hours in rabbits to 4.5 to 7.3 hours in dogs and approximately 15 to 30 hours in rats (2).
Long-term observations in rats and dogs did not show toxic effects on organs such as liver, kidney, or bone marrow. The acute toxicity after oral intake calculated as LD50 is higher than 5,000 mg/kg in rats and mice and in rabbits approximately 1,000 mg/kg (3).
Tests investigating adverse effects on reproduction were performed by feeding rats with STM at doses ranging from 30 to 300 mg/kg. With higher doses, animals became lethargic, appetite decreased, and pregnant animals gained less weight. A significant and dose-dependent decrease in fetal weight with doses higher than 100 mg/kg was observed, and even fetuses exposed to a dose of 30 mg/kg STM were, albeit not significantly, smaller than controls (D. Lorke, personal communication 1985).
In a model designed to investigate the influence of antiepileptic drugs (AEDs) on the developing brain, neuronal apoptosis increased in the brain of newborn rats exposed to standard anticonvulsants such as phenytoin (PHT), phenobarbital (PB), carbamazepine (CBZ), vigabatrin, or valproate (VPA). With STM, neuronal death was comparable with exposure to VPA or vigabatrin, but not as prominent as with PHT or PB (4,5).
STM was proven to be effective against maximal electroshock (MES) (ED50 = 35 mg/kg) and penthylenetetrazol-induced seizures (ED50 = 260 mg/kg) in mice; however, it was ineffective against strychnine-induced seizures (3). The therapeutic index (LD50/ED50) of STM in MES was 10 times higher than that of PB and 4 to 5 times higher than that of PHT. In a primate model—photosensitive baboons (20–125 mg/kg IV)—STM did not show anticonvulsant properties (6). In amygdaloid-kindled seizures in rats, Albertson and coworkers reported no efficacy (7), whereas another group found some effects, which were, however, much weaker and not comparable to those of CBZ, PHT, PB, or zonisamide demonstrated by the same group (8).
Like acetazolamide—although 10 to 20 times weaker (3)—STM has carbonic anhydrase–inhibiting properties (9,10). A familiar and still accepted explanation for the anticonvulsant effect of STM is the inhibition of carbonic anhydrase activity, mainly in glial cells. Subsequently, carbon dioxide concentrations increase, leading to acidification of both intracellular and extracellular space. By acting via N-methyl-D-aspartate (NMDA) receptors and calcium currents, this acidification reduces the excitability of neurons. In addition, there seems to be an independent effect of STM on the function of neuronal ion channels. In isolated neurons from guinea pig hippocampus, STM reduced inactivating sodium currents without changing potassium currents. Therapeutic concentrations of 1 to 10 pg/mL decreased sodium currents to about 20% and led to impairment of repetitive generation of action potentials and a reduction of the maximum discharge frequency by 20% to 40% (11).
BIOTRANSFORMATION, PHARMACOKINETICS, AND INTERACTIONS IN HUMANS
A total of 80% to 90% of oral STM is excreted mostly unchanged in urine, and 10% to 20% is lost with feces after biliary secretion (2). In humans, 32% of a single dose of STM is excreted by the urine within the first 24 hours. The protein binding of STM amounts to 29%.
FIGURE 63.1 Structural formula of STM.
STM serum peak levels were reached after 4 hours (range, 2–8 hours) after a first single oral dose of 5 mg/kg in children with epilepsy taking other AEDs (12). May et al calculated the STM interquartile range (25th to 75th percentile) of serum concentrations as being between 2.3 and 5.8 (mean, 4.7) mcg/mL in 86 patients (2–89 years) on chronic 9.1 ± 4.67 mg/kg STM therapy in combination with other AEDs, mainly CBZ, VPA, or PB (13). In various studies, a linear correlation between STM serum concentrations and the dose/body weight in children and adults could be demonstrated with an STM dose of 4 to 16.7 mg/kg (9,13,14). Children need higher doses per kilogram of body weight than adults to reach comparable serum levels (13). After a single first STM load, serum half-life was calculated to be 3.7 hours (range, 2–7 hours) (12). May et al found somewhat longer STM half-lives (8.65 ± 3.0 hours) at steady state in children and adults on stable comedication with CBZ, VPA, or PB. Half-lives are shorter in children than in adults (7 ± 2 hours versus 12 ± 2 hours) (13).
The decline of plasma concentrations followed in most cases a first-order kinetic; only a few patients’ data were in favor of saturated kinetics. Steady state is reached 3 to 4 days after beginning treatment with STM. This short half-life explains the considerable daily fluctuations in STM concentrations, which explains the failure to establish a correlation between STM drug concentrations and anticonvulsive effect (13,15).
STM is known for its interaction with PHT (16–18). In one study, adult patients with epilepsy on a stable dose of 300 mg PHT daily were started on a concomitant administration of 200 to 800 mg of STM. This caused a rise in serum PHT after one week and prolonged PHT half-life substantially (17). In some patients with already critical PHT serum concentrations, adding STM can provoke PHT intoxication (19). Olesen and Jensen already speculated that this increase of PHT levels is due to a direct inhibition by STM of the parahydroxylation of PHT (18), which was later proven (20). CBZ decreases STM concentrations, probably secondary to enzyme induction (13). STM increased CBZ-epoxide by over 30% in 6 of 39 patients, whereas CBZ levels remained unchanged, and PHT levels increased by more than 30% in 3 of 5 patients (21). No interactions with VPA were observed (13). STM inhibits the metabolism of N-desmethyl-clobazam (DMCLB), a breakdown product of clobazam, through inhibition of CYP2C19 (22).
CLINICAL EFFICACY AND SPECTRUM OF ACTIVITY
STM is one of the older AEDs, developed in the 1950s and introduced into the market in the 1960s. Therefore, much of our knowledge today comes from case studies and open trials of that period. Only two prospective studies evaluating the efficacy of STM in benign epilepsy of childhood with centrotemporal spikes (BECTS) (23) and West syndrome (24,25) were initially performed.
History
Results of the first open trials, mainly in adult patients with temporal lobe seizures, were published in 1960 (25–27); 23 of 81 patients treated with STM (200–1,200 mg/day) became seizure-free (28%), 9 of the 23 taking STM monotherapy; the mean length of follow-up was 6 months (26). Of these 9 patients, 3 stopped STM medication. Seizures relapsed but could be controlled again after reintroduction of STM. Such a high success rate of 28% could not be reproduced in other trials (27,28).
In 1960, Doose et al were the first to demonstrate the efficacy of STM in children with epilepsy (29,30); 129 children with focal, mainly “psychomotor” seizures, secondarily generalized tonic–clonic seizures, as well as generalized grand mal seizures and petit mal with or without grand mal, were treated and observed for more than 6 months. Doose et al made the surprising observation that the best effects of STM were seen in children with psychomotor seizures, being regarded as especially difficult to treat at that time. With STM monotherapy, 13 of 24 children with psychomotor seizures and a further 24 of 41 children with focal seizures (simple and complex partial seizures with or without grand mal) became seizure-free; in 7 children, a reduction in seizure frequency of more than 50% was seen. In reading this old study with our present knowledge, we understand how close Doose et al were to discover independently the concept of BECTS, which was proposed first in 1958 by Nayrac et al and 2 years later by Nayrac and Beaussart (31) and Gibbs and Gibbs (32). It is of historical interest that the focal discharges in the first electroencephalogram (EEG) of a 10-year-old patient later shown to normalize under treatment with 400 mg/day STM would be classified today as rolandic spikes (Figure 63.2) (27).
In 1974, Green et al published a double-blind, crossover study comparing the efficacy of STM with that of PHT, which was defined as the “standard of comparison for anticonvulsant testing” (16). The design of this study is quite unusual, and lack of detailed data makes it impossible to draw one’s own conclusions.
In this study, 67 patients with partial seizures with or without secondary generalization were included. In an open phase, all patients were first placed on a monotherapy with PHT for 2 months before the blinded phase started, during which patients were randomized to either PHT or STM. Whereas PHT was titrated to an individual dose during the open phase, in the blinded phase a ratio of 4:1 STM to PHT was calculated, meaning either that patients remained taking the same dose of PHT, or that a fourfold dose of STM was added, resulting in STM doses of 3.7 to 64.0 mg/kg. In most cases, this dose was 17 to 50 mg/kg (mean, 31.2 mg/kg), being well above recommended doses even at that time. A total of 10 of 67 patients had already been lost during the first open phase on PHT monotherapy, and 36 more were lost during the blinded part of the study—11 taking PHT and 25 taking STM. Only 21 of 67 patients completed the study, 11 taking PHT, 10 taking STM. Of these 21 patients, 10 had fewer seizures with PHT, 9 with STM. Nevertheless, the authors stated that “sulthiame has very little value as a primary anticonvulsant agent” and concluded that “it seems logical … to ascribe the benefit of sulthiame in the control of seizures to the fact that it raises serum PHT levels …. [I]t is easier, more precise and less expensive to increase PHT serum level by administrating more PHT than by giving sulthiame.”
FIGURE 63.2 EEG in a 10-year-old boy (A) before and (B) after a 30-day treatment with 400 mg of STM.
Callaghan et al (14) came to similar conclusions when analyzing 10 adult patients (14–75 years of age; mean, 32.7 years) allocated to 3 to 10 mg/kg/day STM. They concluded that “STM did not appear to be of benefit to patients with severe and frequent seizures. The improvement (seen) was related to an increase in serum phenytoin levels” (14).
These interpretations stopped further clinical trials with STM for a long time in most parts of Europe and North America.
Spectrum of Efficacy
STM is proven to have specific action in BECTS. After BECTS was recognized as a distinct epileptic syndrome in children (31–33), the efficacy of STM was reevaluated by Doose et al (34). In a retrospective open study with a follow up from 7 months to 7 years, they reported that 48 of 56 children (85%) with typical benign partial epilepsy became permanently seizure-free with 4 to 6 mg/kg/day STM.
A 6-month prospective, randomized, double-blind, placebo-controlled multicenter study of STM in BECTS, following the recommendation of the International League Against Epilepsy (ILAE), was published in 2000 (23); 194 patients were screened, and 66 children (40 males, 26 females; age 8.3; range, 3.1–10.7 years) with the diagnosis of BECTS and two or more seizures during the past 6 months were randomized to either STM or placebo for 6 months of treatment. The primary effectiveness was the rate of treatment failure events per group, which were defined as the first seizures after a 7-day run-in period, intolerable adverse effects, development of another epileptic syndrome, or termination of the study by the parents or the investigator. The trial was stopped when the first interim analysis showed a significant superiority of STM treatment; 25 of 31 patients in the STM group (80.6%) but only 10 of the 35 placebo-treated group (28.6%) completed the trial (P < .0001). In only 4 children taking STM but in 21 taking placebo, the failure event was a seizure. Two parents of the placebo group withdrew. All other failure events were due to termination of the trial by the investigators after the interim analysis.
The efficacy of STM in typical and atypical benign idiopathic partial epilepsies was shown by case reports from single centers and in some prospective as well as retrospective open studies (35–37).
In an open prospective trial between 1988 and 2001 and a follow-up of more than 12 months, 21 children with BECTS, atypical rolandic epilepsy, Landau–Kleffner syndrome, or continuous spike waves during slow sleep (CSWS) were treated with STM. In 15 children, STM was the first and only treatment, whereas 10 children had had another treatment before STM. Of 21 children with seizures, 13 became seizure-free, including 10 of 16 patients with BECTS. In 6 of 10, STM was the first drug (37).
A total of 125 children with various epileptic syndromes treated with STM as monotherapy or add-on were analyzed retrospectively in a multicenter study; 24 of 26 patients with benign rolandic epilepsy of childhood (including 3 atypical cases) and all 13 children with benign occipital epilepsy of childhood were treated with STM only. A total of 29 of these 39 children became seizure-free, and a further 6 of 39 showed a reduction of seizures by more than 50% (38).
In a retrospective analysis, 111 consecutive patients with BECTS seen at five pediatric neurology outpatient clinics and one private clinic were collected. All patients were treated with either STM or CBZ according to the institutional policy. Efficacy was defined by seizure freedom for more than 2 years. In 67% of patients treated with STM, “seizures disappeared,” whereas in 74% treated with CBZ, “seizures abated.” Authors stated no significant difference regarding efficacy between the two drugs. It is noteworthy that 5 of 8 patients who were switched to STM after failing CBZ became seizure-free, whereas none of the 3 patients having failed STM became seizure-free after treatment was changed to CBZ (39).
In another single-center study, 35 patients with typical rolandic epilepsy treated either by STM (n = 17), or CBZ (n = 16), or other AED (n = 2; primidone, PHT) were analyzed. A total of 15 of 17 patients in the STM group, but only 9 of 16 in the CBZ group, became seizure-free. The author speculated that in 3 of the 15 STM children, and in 6 of the 9 CBZ children, this outcome could have been due to the natural outcome of BECTS (35).
Of eight children with atypical benign partial epilepsy, five became seizure-free with STM, one with CBZ, and one with adrenocorticotrophic hormone (ACTH). In two children, it was believed that the epilepsy was worsened by CBZ. Both became seizure-free when switched to STM (35).
Gross-Selbeck reported further data on Landau–Kleffner syndrome (LKS) and CSWS treated with various AEDs, including STM. Two of four children with LKS showed a normalization of EEG under STM alone or in combination with clobazam; in the two others the EEG markedly improved. One of three children with CSWS on STM ± clobazam became seizure-free and showed a substantial amelioration of the EEG abnormalities (35). Wakai et al reported on a 5-year-old, previously healthy boy who developed a loss of acquired language, auditory agnosia, and atypical absences and complex partial seizures as well as CSWS. When he received 80 mg/day of STM, there was an excellent outcome regarding all clinical symptoms, accompanied by an improvement of EEG changes and disappearance of CSWS (40).
The second prospective, randomized, double-blind, placebo-controlled, multicenter study on STM, following the guidelines of the ILAE, was done in patients with West syndrome (24): 33 infants with newly diagnosed typical West syndrome (clinically infantile spasms and hypsarrhythmia in the EEG) were included in the study. To exclude pyridoxine-dependent seizures, all received pyridoxine for the first 3 days of the baseline phase, dosed at 150 to 300 mg/kg. On day 4 they were randomized to either STM or placebo. STM was started with 5 mg/kg/day and increased to 10 mg/kg/day when after 3 days no response was recorded. At the end of day 9, an EEG was recorded and study medication revealed. In the 16 patients in the placebo group, neither spasms nor hypsarrhythmia disappeared. In the STM group, 6 of 17 infants showed a complete clinical and EEG response. In the follow-up period, none of these 6 children on STM monotherapy relapsed (P < .022). Three patients of the STM group who failed during the trial period became free of seizures after the STM dose was increased to 15 to 16.4 mg/kg/day. All but one patient of the placebo group received STM in the open follow-up. Four of these 15 patients also became seizure-free.
Lerman and Nussbaum (36) reported on the use of STM in patients with epilepsies with myoclonic seizures comprising cases of Lennox–Gastaut syndrome, myoclonic petit mal, myoclonic seizures in Unverricht–Lundborg disease, and juvenile myoclonic epilepsy (JME). In the retrospective analysis of 12 adolescents and young adults with JME, 8 were controlled completely on STM alone (doses, 400–800 mg/day). In four of these eight patients, STM was the first drug; in the remaining four STM replaced previous drugs, even combinations of several AEDs (36). Ten patients with JME were treated by STM only; 7 of 10 became seizure-free, and another 3 of 10 had a reduction of seizures by more than 50% (38). Kurlemann et al, investigating STM efficacy in newly diagnosed epilepsies, reported on one child with a childhood absence epilepsy who became seizure-free by STM monotherapy as the first drug (41).
STM was shown in old (15,25–27,42–46) and new open, prospective, and retrospective studies to have effects as add-on treatment in cryptogenic or symptomatic localization-related epilepsy in adults as well as in children (21,38,41,47). A total of 17 of 42 children aged 3 to 18 years with a symptomatic localization-related epilepsy treated by STM became seizure-free, and another 12 of 42 had a reduction in seizures of more than 50% (38). Kurlemann et al reported on 48 consecutive children seen at one institution and suffering from simple or complex partial seizures; 28 of 48 (65.1%) treated by STM, which was the first AED in most patients, became seizure-free (41). In an institutional analysis of 49 children with a pharmacoresistant focal epilepsy, 8 became seizure-free after add-on STM, and 6 more patients had a reduction of seizures by more than 50% (follow-up in most cases >21 months) (47).
In a prospective open-label study with 48 adult patients with refractory epilepsy classified either as cryptogenic or symptomatic localization-related epileptic syndromes, STM was given as add-on at a dose of 200 to 600 mg/day. STM and concomitant AEDs were adjusted at the discretion of the investigator as indicated by patient tolerability. A total of 36 of 48 patients completed the titration and continuation phase and could be analyzed. Overall seizure frequency against baseline level was significantly reduced by 36%, with a significant reduction in complex partial and generalized seizures, in the number of clusters and number of days affected by seizures (P < .01 to .001). Twenty-two patients experienced at least a 50% (or more) reduction in seizure frequency; three became completely seizure-free. After more than 2 years, 17 patients having a higher than 50% reduction of seizure frequency still received STM (21).
In a recent study of 20 children with refractory epilepsy treated with STM by Swiderska, 55% of the children had a more than 50% reduction in seizures, and two were seizure-free (48). The best responder rates were seen in those with focal symptomatic or focal cryptogenic epilepsies.
Effect of STM on Epileptiform EEG Activity
The impact of focal discharges in BECTS on cognitive function, language performance, and overall intellectual development is a highly discussed issue. Transition of rolandic spikes to CSWS is possible (34,49,50). Normal neuropsychological and motor functions before and declining with the onset of CSWS have been described (51). CBZ, although effective to treat seizures in BECTS, does not substantially alter the EEG pattern and may even lead to deterioration, including transition to CSWS. Therefore, the influence of STM on the EEG abnormalities in BECTS as well as in CSWS is of special interest.
In a prospective, double-blind trial on STM versus placebo in BECTS, 179 sleep EEGs were recorded at screening and after 4 weeks, 3 months, and 6 months of therapy. All EEGs were analyzed by a blinded reviewer using a standard protocol and building a score by collecting data on epileptiform activity (number of foci, proportion, propagation). At screening, no difference was found between the two groups. A total of 80 foci were identified in 59 patients with sufficient EEG data. Most of them had only one focus, 15 had two, and 3 had three independent foci. The number of patients with EEG normalization was significantly higher in the STM group than in the placebo group. EEG normalization was transient in 12 of 31 in the STM and 4 of 35 in the placebo group, and persistent in 9 in the STM and 1 in the placebo group. Using a complex score for intraindividual comparisons during follow-up, the STM group displayed a significant improvement of this EEG score at 4 weeks and at 3 and 6 months (52).
In another study, the EEG in 21 of 25 children with BECTS and related disorders was controlled 3 to 6 months after the introduction of STM. In 4 of 21, there was a total disappearance; in a further 9 of 21, a marked decrease of discharges in the EEG was seen. Of these 13 children with EEG improvement, a second control EEG remained normal, respectively further improved, in 9 cases. In the EEG of the four remaining children, epileptiform discharges increased again compared to the first control (37). In another group of patients with benign partial epilepsies of childhood, EEG data before and during STM treatment were available in 20 of 39 patients. Complete EEG normalization was obtained in 13 patients 1 to 3 months after initiation of treatment. This EEG normalization was transient in all patients, although seizure control was maintained (38). Similar data were reported in an open trial in 27 children with BECTS; in 70.4% the EEG was normalized (41). In his retrospective study, Gross-Selbeck emphasized the EEG features and treatment-induced EEG changes in BECTS. Of 33 patients suffering from typical and atypical rolandic epilepsy, 16 were treated with CBZ. In this group, the EEG showed no clear improvement, the patients suffered even temporary EEG deterioration, whereas in 14 of 17 patients treated with STM, the EEG showed clear improvement and normalized in 7 children (35). In a study comparing CBZ and STM, EEG normalization was defined only for patients who became seizure-free and in whom an EEG was done during the first year after the last seizure. EEG data were available in 19 patients who became seizure-free with CBZ; in 8 of those 19 the EEG was normalized. In the STM group, EEG data were available for 7, who responded to STM; 5 of 7 had a normalized EEG (39).
A recent German study evaluated levetiracetam (LEV) versus STM in BECTS in 43 children. Treatment failure occurred in four patients in the LEV group versus only two in the STM group; dropout from adverse effects occurred in five in the LEV group and only one in the STM group, with a severe adverse event in one in the LEV group. Therefore, the dropout rate was higher with LEV (53). This study did not include the EEG response to either LEV or STM.
Since the first description of subclinical electrical status epilepticus during sleep (ESES) in children by Patry et al (54), later called CSWS, there has been an ongoing debate on the relationship between BECTS and related syndromes, such as atypical rolandic epilepsy, CSWS, or Landau–Kleffner syndrome. CSWS has been observed to develop in children with prior idiopathic partial epilepsies—either BECTS (35,49) or idiopathic childhood occipital epilepsy (50). It was suggested to try STM in these conditions. In a study on STM in typical and atypical rolandic epilepsy and related epilepsy syndromes (n = 21), four children with cognitive disturbances associated with “focal sharp waves” but without recognized clinical seizures were included and treated with STM. A total of 19 of 21 of all children were investigated by formal neuropsychological testing before and under STM therapy. Neither stagnation nor decline of individual performance was observed. In the four cases without seizures, an improvement of test performance could be documented, but the authors stated that it was not possible to conclude with certainty whether this was the natural evolution or the result of decreased epileptiform discharges (36). Gross-Selbeck reported improvement of language and overall intellectual development in children with atypical benign partial epilepsy and Landau–Kleffner syndrome after STM, which was accompanied by an EEG improvement not obtained with various other AEDs tried previously (35).
Wirrell reported a single case of a child with language delay and ESES, treated with STM at 10 mg/kg/day, in whom clinical improvement with a normal EEG occurred after 1 month (55). Fejerman used STM as add-on therapy for focal epilepsies with encephalopathy with ESES (56). The STM dose ranged between 5 and 30 mg/kg/day, with an average dose of 20 mg/kg in the symptomatic patients versus 14 mg/kg in the idiopathic patients. Of 10 of 28 in the symptomatic group who were seizure-free, 4 had a normal EEG and 6 had residual spikes. Nine of 28 showed a significant seizure reduction. Three of 11 children with unilateral polymicrogyria and ESES became seizure-free and another 6 had a significant improvement in seizure frequency and EEG abnormalities. Twenty-one of 25 patients in the idiopathic group became seizure-free and the EEG became normal in 11, and 10 had residual centrotemporal spikes in less than 3 months after adding STM. STM has also had a beneficial effect in Landau–Kleffner syndrome (57).
Besides its effect on idiopathic rolandic spikes, STM also seems to be effective against focal discharges in symptomatic epilepsy. In adult patients with temporal lobe epilepsy (TLE) (age, 16–43 years) the EEG showed “considerable improvement” in 20 of 35 “marked responders” (>75% reduction of seizure frequency), follow-up being at least 6 months (58). But there is also contradicting evidence. In a prospective blinded study, the number of epileptiform discharges during the first 20 minutes of each waking tracing or the first 20 minutes of sustained sleep EEG was counted. More patients treated with STM showed an increase in epileptiform patterns than those taking PHT (59). Whereas 8 of 13 patients completing the trial had fewer epileptiform patterns compared to baseline at the end of the PHT phase, in 11 of 16 patients, the number of epileptiform patterns increased when taking STM. Kurlemann et al reported on 48 children suffering from symptomatic epilepsies with simple or complex partial seizures and found—besides the clinical improvement—an EEG normalization in 65% of the patients receiving STM (41). Shamdeen et al studied the effect on EEG in 16 children treated with STM at 3 to 10 mg/kg/day (60). The EEG was improved in 75%, with complete normalization in 6 and more than 50% improvement in 5; EEG improvement correlated with improvement in behavior and learning.
Milburn-McNulty has done two Cochrane reviews of STM, one for monotherapy (61) and one for add-on therapy (62). There were only three randomized controlled trials (RCTs) using monotherapy; no meaningful conclusions could be made due to small sample sizes, risk of bias, and absence of data on outcome measures (61). There was only one RCT as add-on therapy in children with West Syndrome; this showed that STM could control seizures, but the sample size was small and was of short duration (62).
ADVERSE EFFECTS
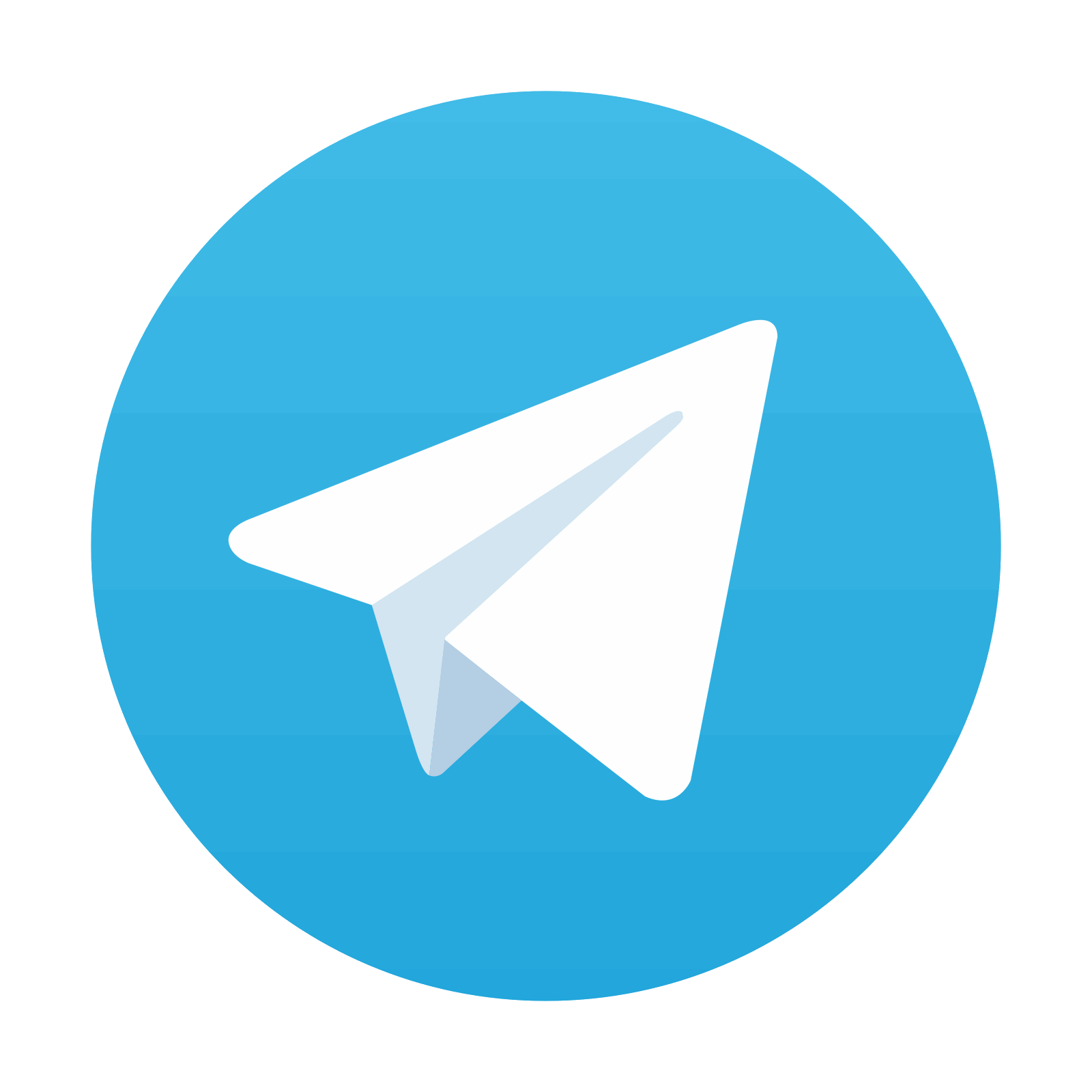
Stay updated, free articles. Join our Telegram channel

Full access? Get Clinical Tree
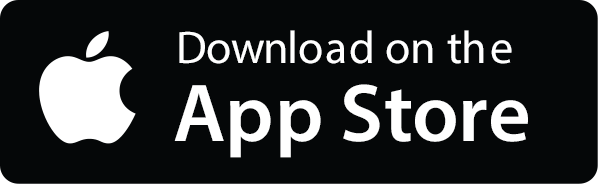
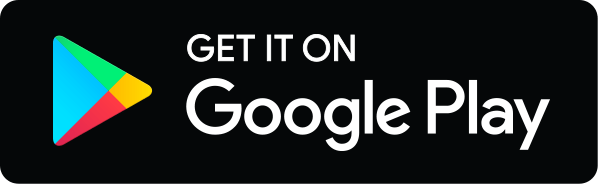