Fig. 4.1
Intensity-modulated radiation therapy (IMRT) plan for a patient with a nasopharyngeal tumor. Upper right panel: dose volume histogram with doses to specific critical structures (chiasm, parotid gland, spinal cord) and differentially dosed volumes
Table 4.1
IMRT dose constraints
Structure | True structure constraint | PRV constraint |
---|---|---|
Brainstem | 54 Gy max. dose | No more than 1 % > 60 Gy |
Spinal cord | 45 Gy max. dose | No more than 1 % > 50 Gy |
Optic nerves, chiasm | 50 Gy max. dose | 54 Gy max. dose |
Mandible, TM joints | 70 Gy, if not attainable, then no more than 1 cc to exceed 75 Gy | |
Brachial plexus | 66 Gy max. dose | |
Oral cavity (excluding PTV) | Mean dose < 40 Gy | |
Each cochlea (GOAL, not hard constraint) | No more than 5 % get 55 Gy or more | |
Eyes | Max. dose < 50 Gy | |
Lens | Max. dose < 25 Gy | |
Glottic larynx | Mean dose < 45 Gy | |
Esophagus/postcricoid | Mean dose < 45 Gy | |
Parotids (note: submandibular and sublingual glands dose reduced as much as possible) | Mean dose in at least 1 gland < 26 Gy and/or 20 cc < 20 Gy and/or 50 % < 30 Gy |
Particle Beams
Charged particle beams such as protons and electrons are also ionizing radiation beams that are used in cancer treatments. The range of the particle in the body is determined by the energy of the beam of incoming particles which are accelerated. Protons as well as heavier ion beams deposit more energy as they go deeper into the body up to a sharp maximum at the end of their range. Near the end of the range of the particle, the residual energy is lost over a very short distance. This results in a steep rise in the absorbed dose, known as the Bragg peak. Beyond the Bragg peak, there is a rapid fall-off of dose to zero. Although the Bragg peak can be very narrow, it can be spread out to cover a longer distance. The distribution of radiation dose in the body of proton beam is characterized by a lower dose in the normal tissue proximal to the tumor, a high- and uniform-dose region across the tumor, and zero dose beyond the tumor. This is in contrast to photon radiation where the ionizing radiation energy passes on through the normal tissue beyond the tumor. The lack of exit dose of protons make proton beam preferable for many situations where a tumor is adjacent to a critical structure such as the spinal cord, which cannot tolerate high doses of ionizing radiation, or in the treatment of children, where avoiding normal tissues will significantly decrease the long-term side effects.
Proton beam for the treatment of head and neck malignancies in children can eliminate the exit dose into the brain, spare or limit the dose to the optic chiasm, salivary glands, and cochlea (Figs. 4.2 and 4.3). Presently, there are only a limited number of proton beam facilities, although more are in development. Nevertheless, this important technological advance has significant advantages in the treatment of children.
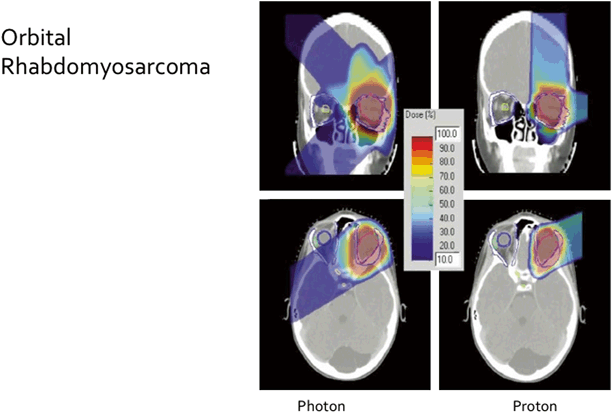
Fig. 4.2
Photon beam and proton beam radiotherapy plans for treatment of an orbital rhabdomyosarcoma (RMS) in a child. Lack of exit dose from proton beam plan is evident in the color wash [8]. (Reprinted with permission from Elsevier)
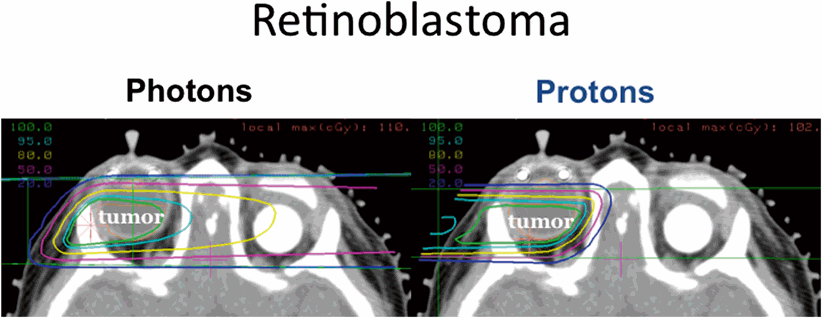
Fig. 4.3
Photon beam (left panel) and proton beam (right panel) plans for irradiation of a retinoblastoma in a child with bilateral disease who has undergone an enucleation. The proton beam plan is able to avoid irradiation of the contralateral eye; courtesy Judith Adams and Shannon MacDonald
Other particle beams such as carbon ions show similar physical advantages to protons. Carbon ions have advantages over protons in that they may be more effective against some more slow growing tumors. However, carbon ion beams are not yet used commonly in the treatment of pediatric tumors.
Specific Examples: Rhabdomyosarcoma and Nasopharyngeal Carcinoma
The indications for radiotherapy, the fields, doses, and timing of radiotherapy due vary depending upon the diagnosis. Several specific examples of two common pediatric tumors of the head and neck are presented in this section. Radiotherapy is used in the treatment of many children with RMS occurring in the head and neck. Radiotherapy is currently recommended for children with RMS whose tumors are inadequately resected as well as for all children whose tumor has alveolar histology [9]. The field design treats the initial tumor with a margin of 1.5−2 cm. Draining lymph nodes are not included unless clinically suspicious or pathologically proven to contain disease. Biopsy confirmation is indicated if nodes are clinically suspicious. The dose of radiotherapy for gross residual disease (group III) is 50.4 Gy in all sites except the orbit, where the dose is limited to 45 Gy. The doses for microscopic disease are 36 Gy for node negative microscopic disease and 41.4 Gy when nodes are positive.
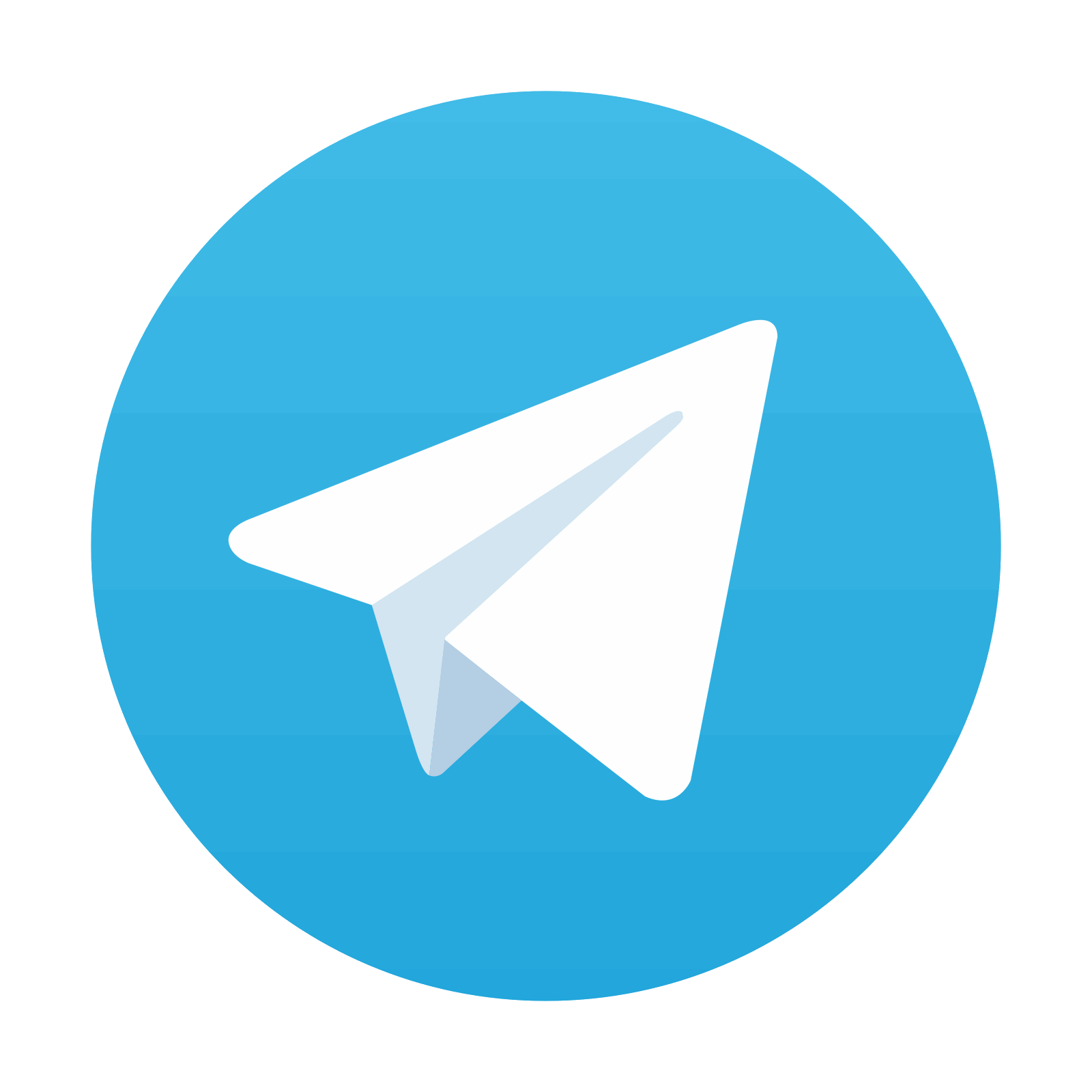
Stay updated, free articles. Join our Telegram channel

Full access? Get Clinical Tree
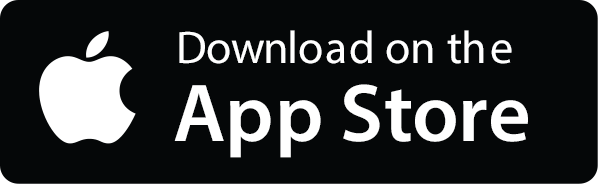
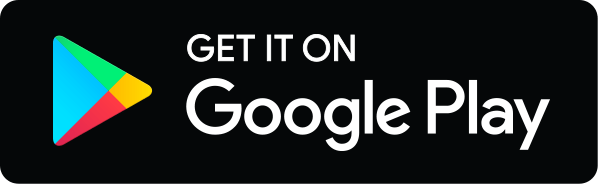