Fig. 1
Radial nerve anatomy and muscle innervation (Courtesy of Shriners Hospital for Children, Philadelphia) (Copyright Dan A. Zlotolow)
As it passes over the anterolateral capsule of the radiocapitellar joint and radial head, the radial nerve divides into a superficial branch (SBRN) and a deep branch, which eventually becomes the posterior interosseous nerve (PIN) (Fig. 2). This cutaneous location over the radial head can leave the radial nerve susceptible to injury from radial head dislocation. SBRN continues deep to brachioradialis muscle and innervates the radial base of the thumb and dorsal hand. PIN continues through the fibrous edge of supinator (known as the arcade of Frohse , the most common site of radial nerve compression) and pierces supinator muscle, eventually supplying the extensor muscles of the wrist and hand (Waters and Bae 2012), as depicted in Fig. 1.
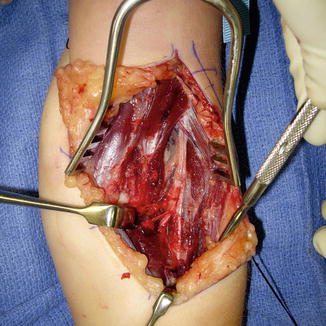
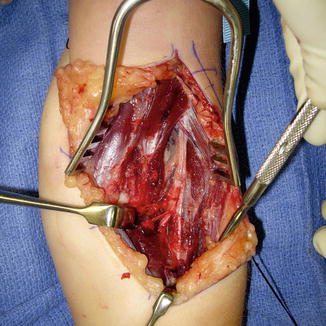
Fig. 2
The radial nerve passes over the anterolateral capsule of the radiocapitellar joint and divides into a superficial branch (SBRN) and a deep posterior interosseous nerve (PIN) (Courtesy of Shriners Hospital for Children, Philadelphia)
Presentation and Symptoms
Clinically, children with radial nerve palsy present with decreased use of the affected arm, wrist drop with weakness and/or loss of finger extension, and sensory loss (Fig. 3). They may also have deformity and swelling if a fracture is present. In patients with humeral shaft fractures, distal motor and sensory function will be lost, and some weakness of supination may be present, but elbow extension is spared, as innervation to triceps occurs proximal to the spiral groove (Hosalkar et al. 2006). In patients with axillary compression of the radial nerve, there will also be weakness of elbow extension and more proximal sensory loss, in addition to the distal deficits (Waters and Bae 2012).


Fig. 3
Seven-year-old status post left humerus fracture with high radial nerve palsy (Courtesy of Shriners Hospital for Children, Philadelphia). (a) Loss of wrist and digital extension. (b) Passive extension of the wrist and attempted active finger extension produces no metacarpophalangeal joint extension
Isolated PIN palsy can occur from injury to the radial head and proximal radius and ulna, as in Monteggia fractures and elbow dislocations. PIN can become entrapped within supinator muscle under the arcade of Frohse , resulting in loss of motor function (finger and thumb extension) without sensory involvement. Because the brachioradialis and extensor carpi radialis longus muscles are spared, the wrist will deviate radially upon attempted wrist extension (Waters and Bae 2012; Spinner et al. 1968).
Diagnosis of nerve injuries and specific muscle testing can be challenging in children. They may be agitated or unable to follow commands for sensory and motor testing and may simply not comprehend the meaning of tingling and numbness (Hosalkar et al. 2006). This can delay accurate diagnosis. It is important to assess all major nerves – radial, ulnar, and median – individually in order to determine motor and sensory deficits. In the older child, two-point discrimination should be tested with the child’s eyes closed, and vibrometry and Semmes-Weinstein monofilament exam can be used to test vibration and pressure thresholds, respectively (Hosalkar et al. 2006). In children with closed head injury and upper extremity fractures who are obtunded or comatose, initial fracture splinting and serial neurovascular examination is necessary to characterize potential nerve injury.
Imaging and Diagnostic Studies
In traumatic situations, radiographic imaging of the entire bone, including the joints above and below the fracture, is recommended. It is important to rule out ipsilateral limb fractures or a floating elbow, such as a concomitant proximal radius and ulna fracture with a humerus fracture. Electromyography (EMG) and nerve conduction velocity (NCV) studies can be useful both as diagnostic and as surveillance instruments for radial nerve injury (Hosalkar et al. 2006). Neurophysiologic NCV testing can help identify the nerve branches that have been affected and, combined with distal muscle response to EMG stimulation, can help determine if signal transmission can occur along a nerve. NCV differentiates demyelination from axonal loss, and EMG distinguishes between neuropraxia versus axonotmesis, or neurotmesis. When the electrical activity information from these needle electrodes is pooled, pathology can be stratified into conduction slowing, demyelinating conduction block, or failure of conduction (Hosalkar et al. 2006).
Early EMG/NCV testing done as a baseline within 10 days of injury can be used when nerve continuity is unknown, to determine if the nerve is transected or contused, which may demonstrate intact but slowed conduction. Young children often have difficulty tolerating needle studies, so anxiolytic medication or conscious sedation may be utilized. A comparison electrodiagnostic study can be repeated 6 weeks after injury to monitor the progress of radial nerve recovery and plan surgical intervention (Hosalkar et al. 2006).
Etiologies of Pediatric Radial Nerve Palsy
Traumatic injuries are the most common causes of radial nerve palsy in children. These include acute fractures of the humeral shaft, supracondylar humerus, and distal humeral condyles, as well as elbow dislocations and proximal ulna Monteggia fractures with associated radial head dislocations. These injuries are diagnosed on radiographic imaging and radial nerve injury identified on careful neurologic examination. Unfortunately, some injuries are missed, such as chronic radial head dislocation and elbow instability, leading to delayed diagnosis and treatment of nerve palsy.
Occasionally iatrogenic damage is a cause of radial nerve injury, for example, in the percutaneous treatment of supracondylar and condylar fractures, as well as in correctional osteotomies of the ulna or humerus (Fig. 4). Tumors, vascular malformations, and congenital or newborn pathologies are also rare etiologies that are encountered (Fig. 5). The literature review below summarizes the outcomes of both operative and nonsurgical management of these diverse etiologies of radial nerve palsy. Traumatic causes are discussed anatomically from proximal to distal.
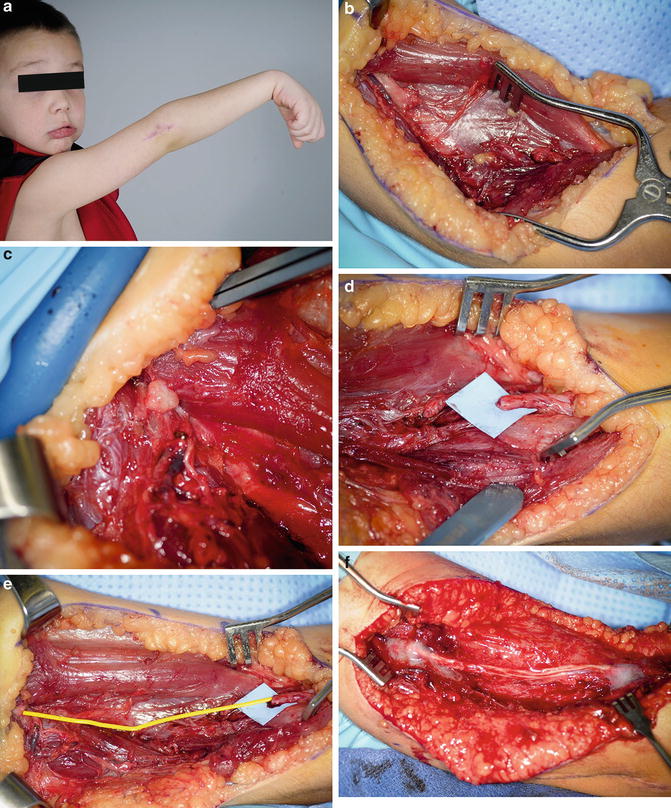
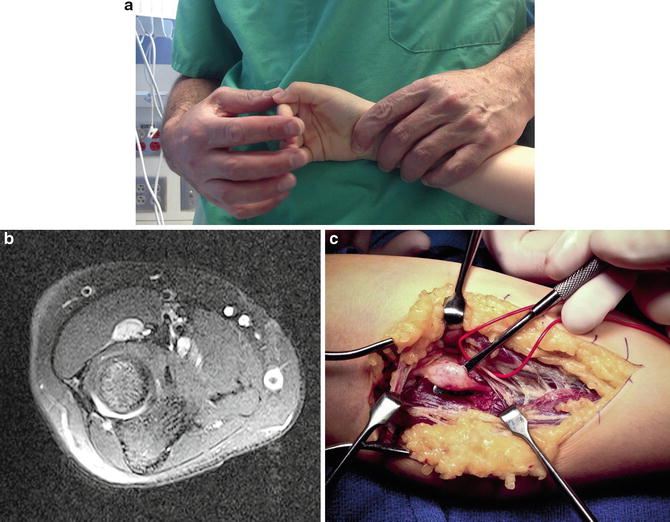
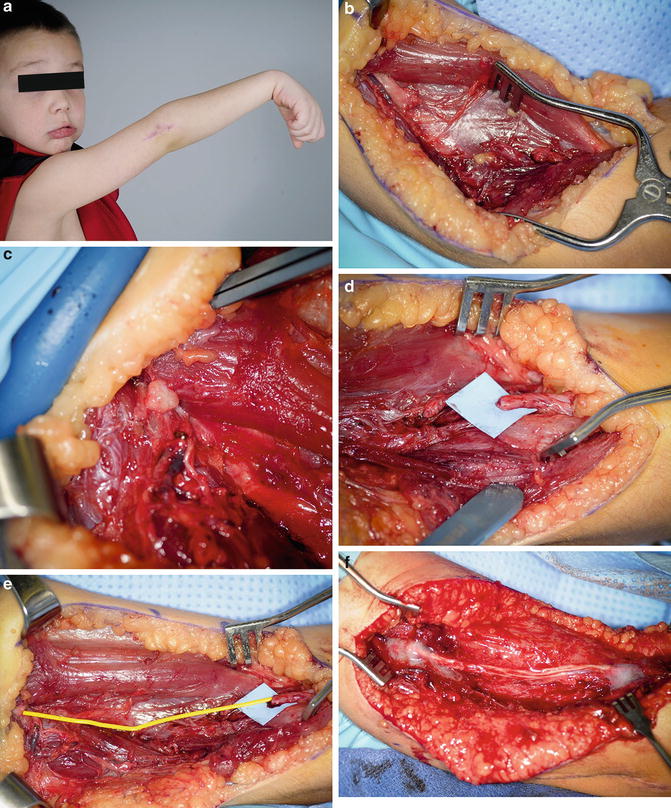
Fig. 4
Four-year-old status post open reduction and internal fixation of a lateral condyle fracture (Courtesy of Shriners Hospital for Children, Philadelphia). (a) High radial nerve palsy with loss of wrist and digital extension. (b) Lateral exploration with nerve discontinuity along with large proximal and distal neuromas. (c) Proximal nerve resected until good viable axons. (d) Distal nerve resected to good distal axons. (e) Long defect between proximal and distal stumps. (f) Sural nerve cable grafting
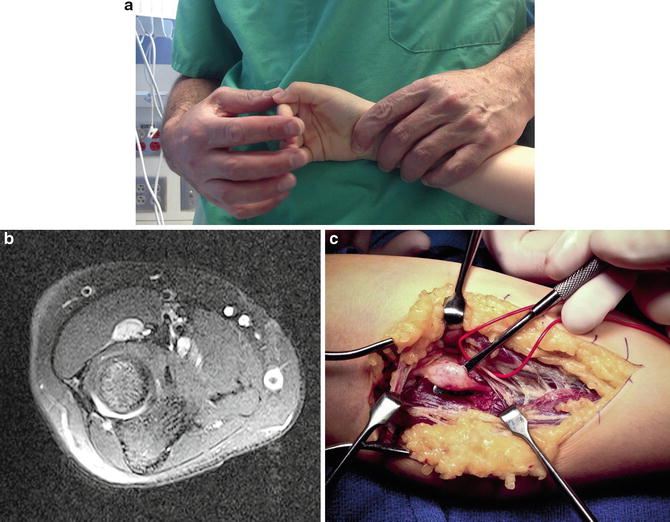
Fig. 5
Ten-year-old with progressive loss of finger and thumb extension (Courtesy of Shriners Hospital for Children, Philadelphia). (a) Video examination with intact wrist extension, but no extrinsic thumb or finger extension. (b) MRI reveals lesion within the posterior interosseous nerve. (c) Surgical exploration with large Schwannoma infiltrating interosseous nerve. Radial sensory nerve is spared (red vessel loop)
Humeral Shaft Fractures
Fracture of the humeral diaphysis is the most common long bone fracture associated with nerve palsy, and the radial nerve is at highest risk, particularly in transverse or spiral fractures of the middle to distal third of the humeral shaft (Shao et al. 2005). Most radial nerve palsies associated with this fracture are from stretch or contusion and do not require acute exploration. However, early surgical exploration is warranted for open humeral shaft fractures and should be considered when nerve palsy occurs after a reduction attempt (Hosalkar et al. 2006). The Holstein-Lewis fracture , a distal third spiral oblique diaphyseal humerus fracture, has a higher incidence of radial nerve injury, 22 % compared to 8 % for other shaft fractures (Ekholm et al. 2008).
There has been considerable controversy over the last few decades regarding expectant versus early surgical management of radial nerve injury in these fractures, but a systematic review by Shao et al. found no statistically significant difference in the rate of nerve recovery with nonoperative and operative treatment (Shao et al. 2005). Although they found that the total number of pediatric patients with humerus fractures was small within the 35 papers included in the study, they recommended a conservative approach to the early management of radial nerve palsy in these fractures for both adult and pediatric patients (Shao et al. 2005). Deferring exploration up to 6 months had no effect on functional outcome, and thus, it is reasonable to delay exploration 2–4 months following injury to observe for early signs of recovery prior to exploration, since 71 % of patients treated nonoperatively recovered spontaneously, and overall recovery has been reported at 88 % (Shao et al. 2005). The authors suggested a treatment algorithm for radial nerve palsy in humeral shaft fractures, which is shown in Fig. 6.
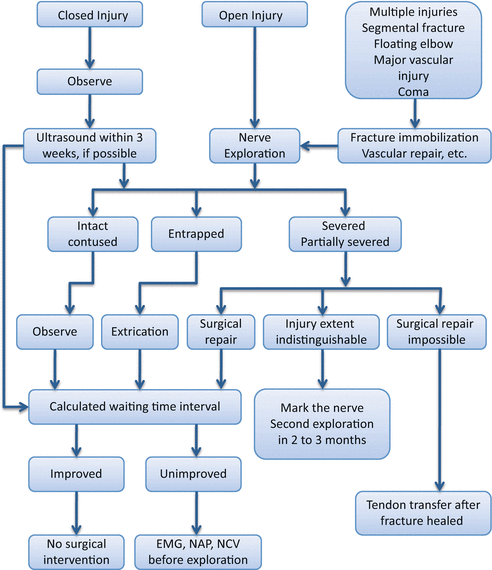
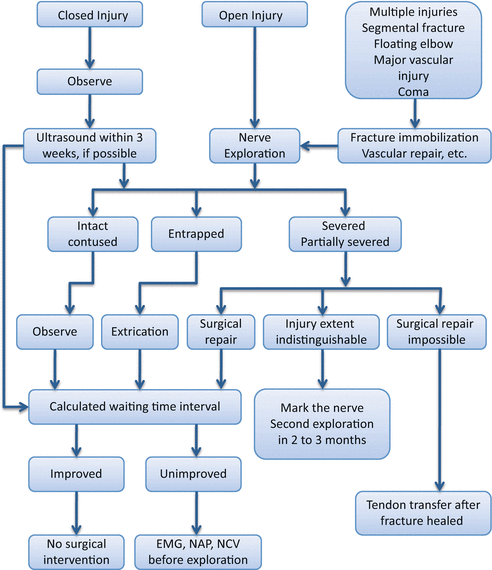
Fig. 6
Algorithm for management of radial nerve palsy caused by humerus fracture (Courtesy of Shriners Hospital for Children, Philadelphia) (Copyright Dan A. Zlotolow)
As children are becoming increasingly involved in competitive sports at an earlier age, unique fracture patterns have emerged. A spiral fracture of the humeral shaft with a high rate of radial nerve palsy has been described in throwing athletes. Ogawa and Yoshida (1998) analyzed 90 patients, 9 of which were children, who sustained these humeral fractures from throwing motions that occurred during recreational baseball. 16 % of all patients had radial nerve palsy, all of which recovered within 8 months. The injury usually occurred during the acceleration phase in an overhand or three-quarter overhand throw, which resulted in an external rotational spiral fracture of humeral shaft. Patients less than 15 years old were more likely to have fractures in the proximal half of the diaphysis. Approximately half of the patients were treated nonoperatively with a functional brace and the other half with surgical plate fixation without any nerve procedures, and all patients had full functional recovery (Ogawa and Yoshida 1998).
The literature has limited information specifically regarding the surgical management of radial nerve injury from humeral shaft fractures in children. A case report by Ogawa et al. (2007) described successful nerve grafting for a 3-year-old child with a closed humerus fracture resulting in radial nerve palsy. After EMG demonstrated no recovery of function 3 months post-injury, they explored the fracture site and noted complete transection of the radial nerve with substantial retraction of the proximal end. Cable grafting with 8 cm of sural nerve autograft resulted in successful recovery of radial nerve function at 6 months (Ogawa et al. 2007).
Amillo et al. (1993) reported on 12 patients with radial nerve palsy treated surgically, 4 of which were pediatric patients. All four children presented with complete motor and sensory deficits, confirmed on EMG at 3 months post-injury. Two of the four underwent simple neurolysis for fibrosis around the radial nerve, one had sural nerve interfascicular grafting for callus entrapment, and one had tendon transfers because the nerve had been divided during the injury. Three had excellent motor and sensory recovery, and the child who underwent tendon transfers had good motor function with limited sensation. The authors emphasized the importance of surgical exploration if there are no signs of nerve recovery 3 months post-injury, and preoperative EMG offers useful information about the extent of nerve damage (Amillo et al. 1993). A similar study by Larsen and Barfred (2000) evaluated 26 patients with a median age of 21 who had radial nerve palsy after humeral shaft and supracondylar humerus fractures. They reported good nerve recovery within 4–5 months with expectant management for 21 patients, and for the 5 patients who showed no recovery, surgical exploration, sural nerve grafting, and tendon transfers also resulted in favorable outcomes (Larsen and Barfred 2000).
Supracondylar Humerus Fractures
Neurologic injuries from supracondylar humerus fractures in children are common, with rates ranging from 5 % to 22 % (Culp et al. 1990; Dormans et al. 1995; Louahem et al. 2006; Babal et al. 2010). Lesions can be seen in the ulnar, radial, median, anterior interosseous, and multiple nerves. Although older studies suggested the radial nerve was most commonly injured, recent meta-analyses have found that the anterior interosseous nerve is most frequently affected (34 %) in extension type and the ulnar nerve (91 %) in flexion-type fractures (Babal et al. 2010). Iatrogenic nerve injuries also occur in 2–3 % of patients during the treatment of these fractures (Gosens and Bongers 2003; Babal et al. 2010; Royce et al. 1991). Similar to radial nerve injuries associated with humeral shaft fractures, most radial nerve palsies recover spontaneously following closed supracondylar fractures, so clinical observation is recommended. If no recovery is seen after a period of expectant management, surgical options include exploration and neurolysis, nerve grafting, and nerve or tendon transfers. Again, nerve traction and contusion are more common than complete disruption (Louahem et al. 2006; Gosens and Bongers 2003; Galbraith and McCullough 1979).
Radial nerve injury has been reported in 6–41 % of supracondylar humerus fractures (Louahem et al. 2006; Babal et al. 2010). Posteromedial fracture displacement is more likely to result in neurologic injury (Hosalkar et al. 2006). Culp et al. (1990) retrospectively evaluated six patients with radial nerve palsy in a series of 101 consecutive supracondylar fractures. They found that four children recovered spontaneously within 2–5 months and one child required neurolysis, after which he recovered in 6 months. The final patient, who had a transected nerve, did not recover function despite sural nerve grafting and required tendon transfers (Culp et al. 1990). When combined with their data for median and ulnar nerve postsurgical outcomes, they concluded that neurolysis after a 5-month period of observation offered good results for recovery of nerve function in these injuries. Dormans et al. (1995) found comparable outcomes in their series of 200 Gartland extension type III supracondylar humerus fractures. They reported five radial nerve injuries, all of which recovered without surgery within 4 months (Dormans et al. 1995). In the series of 617 patients studied by Gosens and Bongers (2003), 10 radial nerve injuries were identified. Nine of these recovered spontaneously after closed or open reduction and pinning of the supracondylar fracture and one patient required radial nerve repair with an interposition autograft (Gosens and Bongers 2003). Three of these patients also had concomitant brachial artery injury, so it is important to monitor vascular status during the evaluation of these patients. Recent studies have emphasized the fact that incidence of vascular and neurologic injury increase with severity of Gartland classification of fracture displacement (Tomaszewski et al. 2012; Gosens and Bongers 2003). Another study from France involving 210 children with supracondylar fractures reported a high rate of neurologic injury (22 %), including 13 radial nerve palsies (Louahem et al. 2006). Prognosis is good for spontaneous functional improvement, so they encouraged a more conservative strategy. The rare complete transections, however, require repair or nerve grafting.
Another potential cause of radial and other nerve injuries in supracondylar humerus fractures is iatrogenic damage during fracture stabilization. Flynn et al. (1974) published a 16-year follow-up study that described a blind percutaneous pinning technique with serial intraoperative radiographs used to treat 72 children with displaced supracondylar fractures. Vascular complications occurred in 18 % of these cases and neurologic complications in 11 %, including two patients with radial nerve palsy who recovered spontaneously (Flynn et al. 1974). Since then, as fluoroscopic imaging technology has improved live fracture visualization, multiple studies have described iatrogenic complications from pinning of supracondylar fractures. Royce et al. (1991) reported four cases of iatrogenic nerve injury, including three ulnar nerve palsies and one radial nerve palsy. Injury to the radial nerve in this patient occurred during placement of the medial Kirschner wire. After starting the pin in the medial epicondyle, penetration of the pin through the lateral cortex and plunging resulted in complete radial nerve palsy post-op. The nerve was explored on the same day and found to be in continuity but contused, with functional return occurring over 3 months (Royce et al. 1991). A similar report of iatrogenic radial nerve palsy in 2012 described the exact moment when radial nerve injury occurred in one patient, when the medial K-wire exited the lateral humeral cortex and the patient’s wrist jerked into extension (Krusche-Mandl et al. 2012). Although the patient had a postoperative wrist drop, function returned in 13 weeks. Radial nerve palsy, in addition to ulnar nerve injury, has also been described during open reduction and pinning of supracondylar fractures (Brown and Zinar 1995). Medial pins started too posteriorly on the medial epicondyle can affect the ulnar nerve, and again, lateral cortical penetration can be injurious to the radial nerve (Fig. 7).
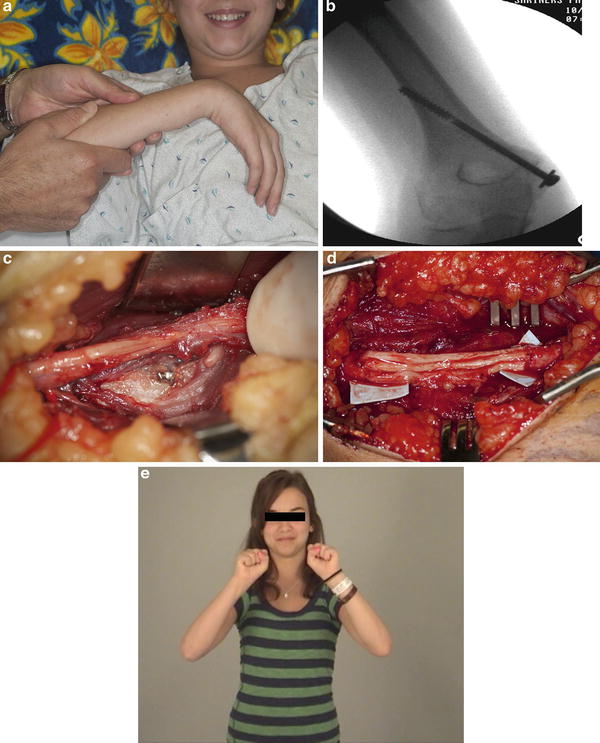
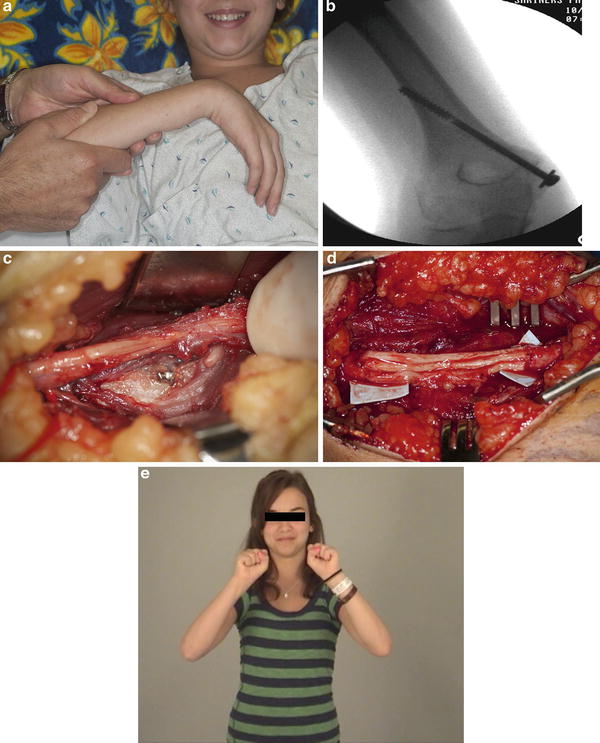
Fig. 7
Twelve-year-old female level 7 gymnast 5 months following open reduction and internal fixation of right medial epicondyle fracture associated with an elbow dislocation (Courtesy of Shriners Hospital for Children, Philadelphia). (a) Clinical examination with absent wrist and digital extension. (b) X-ray showing fixation with 3.5 mm cannulated screw. (c) Exploration revealed neuroma incontinuity. (d) Sural nerve cable grafting. (e) Video demonstrating excellent outcome
Current teaching for placement technique of the medial K-wire includes making a small incision along the medial epicondyle to openly retract the ulnar nerve, as well as to use fluoroscopic imaging to avoid plunging through the lateral humeral cortex, which can wrap up or add traction to the radial nerve (Brown and Zinar 1995; Gosens and Bongers 2003; Royce et al. 1991). Although percutaneous pinning of supracondylar fractures is a relatively safe, effective technique, surgeons must be aware of the 1–3 % risk of iatrogenic nerve injury (Gosens and Bongers 2003; Babal et al. 2010; Royce et al. 1991; Krusche-Mandl et al. 2012; Brown and Zinar 1995) and be careful in their operative technique.
Condylar Fractures and Elbow Dislocations
Dislocations of the elbow and fractures of the lateral and medial humeral condyle have been reported as less common causes of radial nerve palsy. PIN palsy was first reported in 1936 in a patient after acute lateral condyle fracture (Wilson 1936), and transient paralysis of the radial nerve was again described a decade later (McDonnell and Wilson 1948). Another study presented two patients with PIN palsy after open treatment of lateral condyle fractures (Smith and Joyce 1954). Although tardy ulnar neuropathy is a more common nerve injury that can occur from lateral condyle nonunion resulting in elbow deformity, clinicians must also evaluate the radial nerve in both nonoperative and surgical management of these fractures (Hosalkar et al. 2006).
Medial epicondyle fractures can occur in conjunction with elbow dislocations and may be associated with ulnar nerve palsy, but surgical treatment of these fractures may result in radial nerve injury. Marcu et al. (2011) describe two patients who underwent open reduction and cannulated screw fixation for medial epicondyle fractures. In the first patient, retrograde screw placement was done through a medial approach, and care was taken not to pass-point the terminally threaded guide wire beyond the lateral cortex of the humerus, but unrecognized advancement may have occurred. The patient had radial nerve palsy postoperatively despite an uneventful procedure, and she underwent screw removal and nerve grafting, eventually recovering full function at 9 months. Similar fixation was completed in the second patient, but intraoperative advancement of the guide pin occurred laterally, and twitching was noted within the mobile wad. She had spontaneous recovery of wrist extension at 3 months (Marcu et al. 2011). The authors emphasize the dangers of cannulated screw fixation of fractures, including unrecognized guide pin advancement and problems with the pin such as bending or breakage. They suggest some reasons that can be attributed to radial nerve injuries and offer techniques to avoid these problems. The use of terminally threaded pins, which make it more difficult to feel when the pin has breached a cortex, can be substituted with smooth pins, which also cause less soft tissue damage. Another problem with cannulated systems is that the drill can advance a pin unintentionally while spinning over the pin. More generous use of live fluoroscopy to avoid unwanted advancement has been recommended (Marcu et al. 2011). These challenges and technique suggestions are presented to avoid radial nerve palsy as a complication.
Other rare injuries can also lead to radial nerve injury. Abu-Jayyab et al. (2011) published a case report of an 8-year-old child with combined lateral condyle and medial epicondyle fractures with transection of the radial nerve. She underwent radial nerve primary repair and recovered full nerve function in 6 months (Abu-Jayyab et al. 2011).
Monteggia Fracture-Dislocations and Forearm Fractures
Monteggia fractures of the proximal ulna with radial head dislocation have frequently been associated with radial nerve and PIN palsy in children, even since Bado classified the lesion in 1962, with up to 43 % of patients with these fractures having neurologic injury (Jessing 1975). As the PIN travels through the supinator muscle adjacent to the radial head and neck, it is susceptible to injury with anterior or lateral radial head dislocation and often gets entrapped at the arcade of Frohse (Fig. 8; Spinner et al. 1968). If a radial head is found to be irreducible, the radial nerve may in fact be entrapped beneath it or wrapped around the radial neck, and open reduction using an anterior approach is necessary (Morris 1974). Direct nerve trauma and traction neuropraxia are both causes of palsy (Ruchelsman et al. 2009; Stein et al. 1971). Return of nerve function after closed reduction of Monteggia fracture-dislocations can either be acute within a few days or delayed, as reported by Spinner et al. in three children (1968). Two of the patients had full motor function within 14 days after the fracture; however, the other patient took 5 months to regain all function (Spinner et al. 1968).
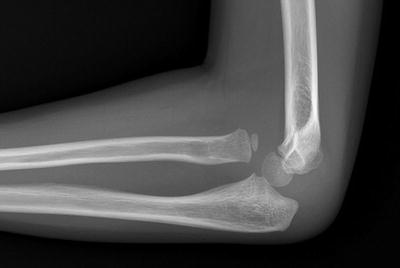
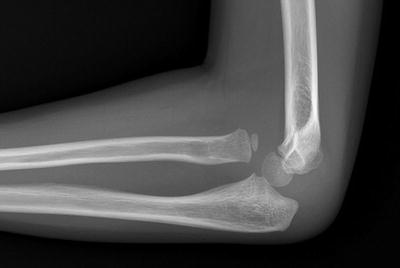
Fig. 8
Lateral x-ray demonstrating anterior dislocation of the radial head. This condition can result in injury to the PIN as it travels through the supinator muscle at the arcade of Frohse (courtesy of Shriners Hospital for Children, Philadelphia)
Wiley and Galey (1985) published a series of 46 children with Monteggia fractures, of which 4 had either radial nerve or PIN palsy. 48 % of these children sustained anterior radial head dislocation (Bado I), 39 % had lateral dislocation (Bado III), 11 % had posterior dislocation (Bado II), and 2 % had type IV injury with fracture of the proximal radius. Notably, 41 % of patients had olecranon fractures. The fractures were treated with closed or open reduction and pinning, but none of the nerve palsies required further surgery and recovered spontaneously.
A favorable prognosis is seen for most children following closed reduction (Wiley and Galey 1985; Hosalkar et al. 2006), while adults require advanced surgical intervention more frequently (Jessing 1975). Stein et al. (1971) presented seven adult soldiers who sustained Monteggia fractures with PIN and radial nerve palsy, six of which were explored operatively and three of which required tendon transfers secondary to failure of nerve recovery. They also noted an association with ulnar nerve injury (Stein et al. 1971).
Malunion of a Monteggia fracture that occurs at a young age can lead to acute or tardy progressive radial nerve palsy, with one case reported 65 years after the initial injury, demonstrating the importance of early recognition and treatment of this fracture-dislocation (Austin 1976; Holst-Nielsen and Jensen 1984). Redislocation of the radial head after closed reduction can occur with cast immobilization, so serial radiographic follow-up is required. Ulnar malunion and persistent radial head dislocation can lead to symptomatic PIN palsy in children as well, which can be treated with corrective osteotomy and neurolysis of the chronically compressed nerve. Ruchelsman et al. (2009) reported a surgical technique for management of PIN palsy in a child after chronic type I Monteggia fracture malunion with persistent anterior radial head dislocation. The patient had persistent motor weakness 9 months after injury and was treated with surgical exploration, where the radial head was found to be dislocated between the superficial radial nerve and PIN. Considerable epineural fibrosis was seen at the arcade of Frohse , and microsurgical neurolysis was completed. After extensive release of the scarred capsule of the proximal radioulnar joint, an osteotomy and plating of the proximal ulna was performed, followed by open reduction of the radial head and annular ligament reconstruction with ulnar periosteum. Radiocapitellar pinning was used to stabilize the reduction. The child had full restoration of PIN function within a year after neurolysis and reconstruction (Ruchelsman et al. 2009). Based on this, the authors favor simultaneous treatment of the malunion and nerve compression in order to avoid later tendon transfers.
In another case series of 13 children, Wang and Chang (2006) report the outcomes of a similar procedure consisting of open reduction and ulnar osteotomy to treat chronic anterior radial head dislocation. Annular ligament reconstruction was also performed. The authors found that successful reconstruction of chronic Monteggia fracture-dislocations is possible but can have complications of iatrogenic transient PIN palsy, redislocation, and delayed union of the osteotomy (Wang and Chang 2006). One pitfall to discuss is the complication of iatrogenic PIN palsy that can occur if corrective ulnar osteotomy is completed without an open reduction of the radiocapitellar joint. A case report presented a 6-year-old treated with osteotomy without exposure of the radial head (Osamura et al. 2004). The blind reduction of the radiocapitellar joint resulted in transection of the PIN. The patient had no recovery of motor function and, on exploration, the distal nerve stump could not be identified, so tendon transfers were performed (Osamura et al. 2004). This emphasizes the importance of diligent neurologic examination and surgical exposure in the care of children with this fracture pattern and radial nerve injury.
In adults, good outcomes are reported with simple neurolysis alone rather than ulnar osteotomy and ligament reconstruction for tardy PIN palsy in patients with chronic radial head dislocation (Holst-Nielsen and Jensen 1984). In children, however, restoration of anatomic relationships with reconstruction is preferred to allow normal long-term function.
Other forearm injuries, including diaphyseal radius and ulnar fractures and Galeazzi fractures of the radius with distal radioulnar joint dislocation, have also been associated with PIN palsy, but the incidence is lower secondary to the larger soft tissue envelope relative to the elbow (Hosalkar et al. 2006; Moore et al. 1985). Tubbs et al. (2013) presented a child who sustained a proximal radial shaft fracture malunion, which resulted in recurrent radial head dislocation and compression of the PIN at the arcade of Frohse . Exploration and nerve decompression led to full recovery of PIN function in 5 months (Tubbs et al. 2013). Injury to the posterior interosseous nerve occurs more often during open reduction and fixation of Galeazzi and both bone forearm fractures. Moore et al. (1985) described a large series of patients with open plating of Galeazzi fractures with an incidence of 8.5 % for iatrogenic injury, in which 8 % of subjects were children (Moore et al. 1985). Median and ulnar nerve injury or compression is more common than superficial radial nerve injury in more distal radius and ulnar fractures.
Neonatal, Congenital, and Pathologic Causes
Newborn radial nerve palsy is a rare entity, but a variety of causes can lead to infant wrist drop, including intrauterine positioning (Alsubhi et al. 2011), maternal anatomy during vaginal delivery (Morgan 1948; Alsubhi et al. 2011), reduced fetal activity from reduced amniotic fluid volume (Lundy et al. 2009), obstetric humerus fracture (Morgan 1948), constriction bands (Uchida and Sugioka 1991), infection (Lejman et al. 1995), tumors, malformations, and lesions of the spinal cord. Treatment of these palsies after birth includes splinting the arm with the wrist and fingers in extension, and therapy to encourage wrist motion. Fortunately, most newborns recover spontaneously from these in utero or birth injuries to the radial nerve.
Prolonged intrauterine hyperflexion of the wrist with persistent pressure on the flexed hand can result in wrist drop at birth. Cases of bilateral radial nerve palsy have also been reported, where prolonged vaginal delivery results in tetanic uterine spasm that creates a contraction ring of the cervix, thereby compressing the radial nerves at the level of the humeri or elbows (Morgan 1948). An abnormal uterine obstruction during labor, known as Bandl’s ring, can also have a similar effect.
Neonates with isolated radial nerve palsy are expected to recover without surgical intervention. Hayman et al. (1999) described four children who had complete recovery of radial nerve paresis within 1 month. They demonstrated EMG/NCV evidence of acute denervation and later reinnervation and also did skin biopsies of contused areas in the upper arms of these infants, which revealed subcutaneous fat necrosis (Hayman et al. 1999). A larger case series by Alsubhi et al. (2011) presented 25 children with isolated radial nerve palsy after delivery, mounting to an incidence of 2.6 % in a sample size of 953 infants. This isolated palsy may in fact be underdiagnosed or misdiagnosed, so clinical suspicion should be maintained. Radial nerve palsy was differentiated from obstetric brachial plexus palsy, which also has shoulder dysfunction and loss of elbow flexion. Seventeen of the 25 affected newborns were noted clinically to have bruising (28 %) or a firm subcutaneous nodule of fat necrosis (68 %) in the posterolateral aspect of the arm (Alsubhi et al. 2011). The major perinatal risk factor for this injury was prolonged labor or failure of labor to progress, usually requiring instrumented vaginal delivery or C-section. Protracted pressure on the arm or elbow by the pelvic brim was found to be the source of compression. All of the infants recovered full radial nerve function within 6 months, mostly before 2 months, so isolated neonatal radial nerve palsy has a much more favorable prognosis than lower brachial plexus palsy (Alsubhi et al. 2011; Lundy 2011; Hayman et al. 1999).
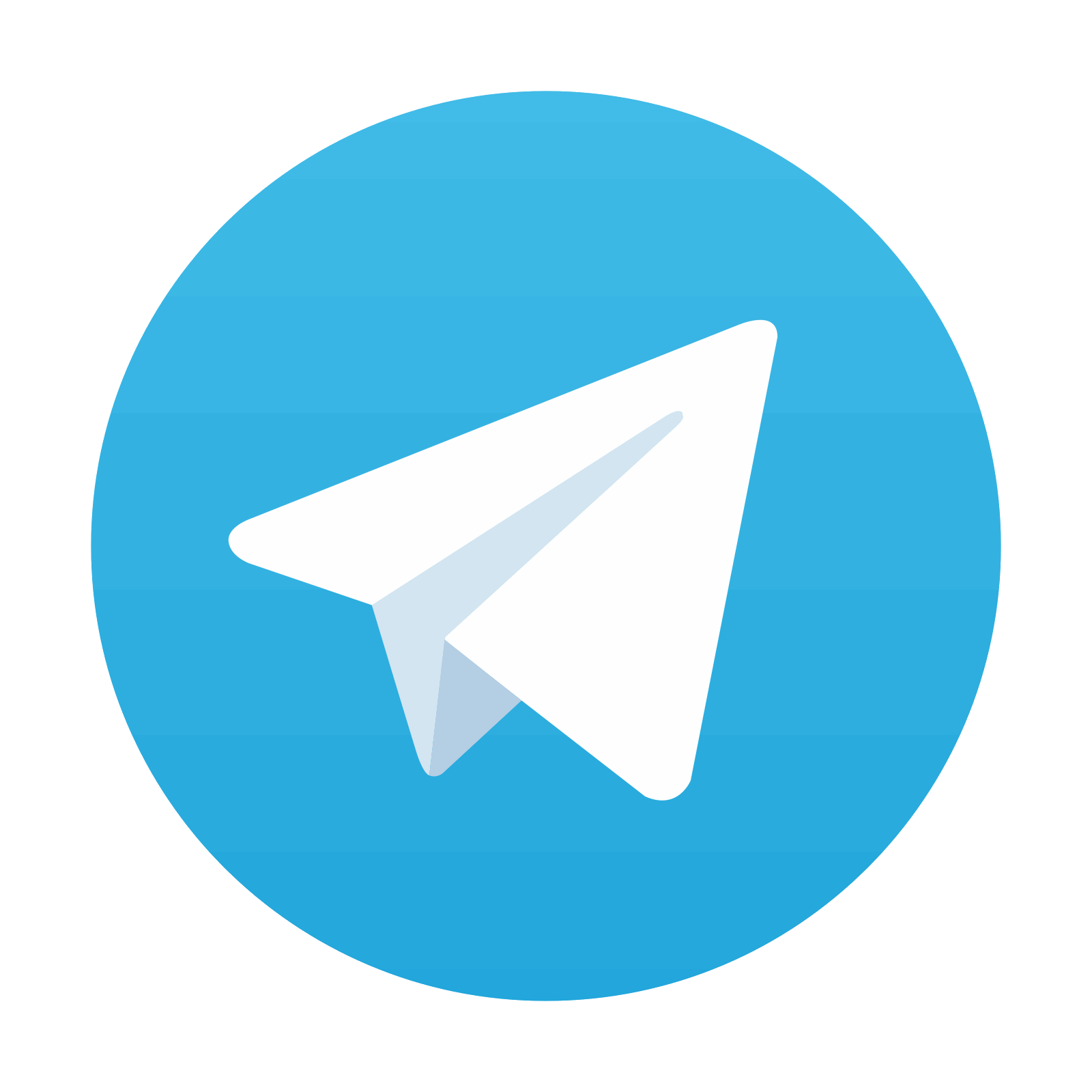
Stay updated, free articles. Join our Telegram channel

Full access? Get Clinical Tree
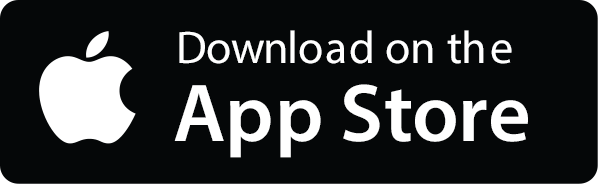
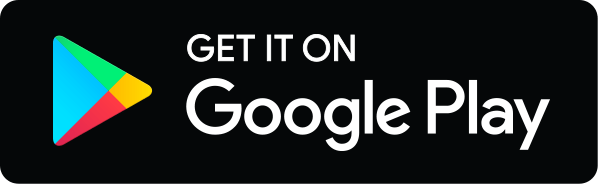