Pulmonary function testing encompasses a variety of techniques and tests. The indications for pulmonary function testing include: (1) documenting the presence of obstructive or restrictive abnormalities in the course of establishing a diagnosis, (2) monitoring the course of a known pulmonary disease (e.g. cystic fibrosis, asthma), (3) monitoring for pulmonary toxicity related to treatment (e.g. amiodarone, radiation, chemotherapy), (4) monitoring response to therapy, and (5) describing normal and abnormal lung growth.
A common use of pulmonary function tests in the inpatient setting is to monitor response to therapy. For example, patients with cystic fibrosis receiving intravenous antibiotics for a pulmonary exacerbation of their disease are usually monitored weekly with spirometry. The patient’s prior baseline (or previous best) lung function is a helpful target when determining the duration of therapy. Measurement of spirometry or exhaled nitric oxide may help determine duration of prednisone therapy for an acute exacerbation of asthma. Pulmonary function tests may also assist in preoperative planning, such as for patients with restrictive lung disease undergoing scoliosis repair. A less common scenario would be to monitor the progression of muscle weakness in a patient with Guillain-Barre syndrome. Additionally, these tests have important uses in the evaluation of respiratory symptoms (shortness of breath, cough, wheeze) when there is no specific diagnosis.
The tests described herein require specialized equipment and experienced personnel. The equipment and laboratory should conform to the standards published by the American Thoracic Society,1 including daily calibration, biologic controls, and infection control practices. A stadiometer for accurate height measurement is required. The personnel should be patient, have the appropriate training, and be comfortable working with children of a variety of ages, who often require different coaching strategies. Most children by the age of 6 years, are able to follow directions and perform spirometry maneuvers. Some children as young as 3 to 4 years are able to perform spirometry.2 The space for testing should be quiet and free of distractions. Recently, some primary care physicians have begun to use spirometry in the office setting for patients with asthma or chronic obstructive pulmonary disease. The accuracy of testing in this situation is not well documented.
The values measured in the laboratory are usually normalized with the use of reference equations, most commonly based on the subject’s height, gender, age and ethnicity. With these equations, a predicted value can be calculated for each parameter, and the measured flows can be reported as a percentage of the predicted value or as a standardized deviation score (Z-score). Test results from different pulmonary function laboratories may not be directly comparable if different reference equations are used.
Spirometry is the measurement of airflow during a maximally forced exhalation. The test is informative because airflow rates are inversely proportional to the fourth power of the radius of the airway; small or obstructed airways result in reduced airflow rates. Indeed, the hallmark of obstructive lung diseases such as asthma and cystic fibrosis is reduced airflow. Further, in small airways, flow limitation usually occurs, necessitating minimal effort to achieve a maximal flow. The parameters measured by spirometry are fairly reproducible in subjects, making them useful for assessing response to treatment over time. Revised standards for the performance of spirometry have been published.3
To perform spirometry, the subject breathes through a mouthpiece connected to a pneumotachometer while wearing nose clips. After inhalation to total lung capacity, the subject is coached to exhale rapidly and forcefully, for a minimum of 6 seconds, until the lungs have emptied. At least three efforts are required, with two efforts having an exhaled volume within 5% of each other, to meet the criteria for reproducibility.
Several parameters can be calculated from these maneuvers. First, the total exhaled volume is termed the forced vital capacity (FVC). The forced expiratory volume in the first second is the FEV1. The forced expiratory flow in the mid-expiratory phase (i.e. the airflow rate between 25% of the exhaled volume and 75% of the exhaled volume) is the FEF25%-75% (or occasionally referred to as the maximal mid-expiratory flow). Other instantaneous flow rates can also be calculated with reference to a different exhaled volume (e.g. FEF50, FEF75). The patterns of these parameters can suggest an obstructive or a restrictive defect (Table 146-1). The FEV1:FVC ratio may help distinguish obstructive from restrictive diseases, although measurement of lung volumes is required to accurately diagnose restrictive disease.
When flow is plotted against exhaled volume (Figure 146-1A), the resultant curve can help locate the site of any flow limitation. Intrathoracic obstructive defects such as asthma and cystic fibrosis usually result in a curve that is concave to the volume axis (Figure 146-1B), whereas restrictive defects such as pulmonary hypoplasia or neuromuscular weakness usually have a normally shaped but narrow curve (Figure 146-1C). Central airway obstruction can manifest as flattening of the inspiratory limb if the lesion is variable and extrathoracic (e.g. laryngomalacia or vocal cord paresis) or flattening of both the inspiratory and expiratory portions of the curve (Figure 146-1D) if the lesion is fixed in nature as in fixed subglottic stenosis.
FIGURE 146-1.
Flow-volume curves. The normal curve (A) has a smooth, linear decrease in flow from near total lung capacity (to the left) to residual volume (to the right). Note that time is not represented in these curves but is recorded in a separate volume-time curve (not shown). In a patient with obstructive lung disease (B), the curve is concave toward the volume access, such that for any given lung volume, measured flow is lower than predicted. Restrictive lung diseases (C) result in a curve that has a small, exhaled lung volume and is narrow, but without concavity. Fixed airway obstruction (D) results in a curve that is flattened in both inspiration and exhalation.

If an obstructive defect is documented, reversibility can also be assessed using spirometry. A bronchodilator is administered, and the test is repeated in 15 to 20 minutes. Commonly, a 12% increase in the FEV1 is considered indicative of a significant response. Similarly, in patients with suspected airway hyperreactivity, spirometry can be performed before and after provocative agents such as methacholine, cold air, or exercise.4 In this case, a decrease in the FEV1 of 10% to 15% indicates airway hyperreactivity.
Peak expiratory flow is measured during spirometry but is also commonly measured in the ambulatory setting using a hand-held flow-measuring device. Subjects inhale maximally (to total lung capacity, TLC) and then exhale quickly with maximal force, and the resulting maximum, or peak flow is indicated on the device. The convenience of this relatively inexpensive modality makes it potentially beneficial for monitoring daily symptoms in patients, especially those that are poor perceivers or in research studies. It can also be used to serially assess patients in the emergency department or hospital, to assist in monitoring recovery. However, peak expiratory flow is dominated by flows in large airways, and as such, it is very effort dependent. Additionally, peak expiratory flow can be preserved in the presence of significant small airway obstruction. Several studies have shown subjective reporting of symptoms to be as reliable as peak flow monitoring in identifying and treating asthma exacerbations.
Lung volume measurements are necessary to diagnose restrictive lung disease. Disease states that affect lung growth alter both airway caliber and lung volume. These diseases include pulmonary hypoplasia due to severe oligohydramnios or space-occupying lesions (e.g. diaphragmatic hernia, cystic adenomatoid malformation), bronchopulmonary dysplasia, and conditions that alter growth of the rib cage (e.g. thoracic dystrophies, scoliosis, radiation). Restriction may also be due to interstitial lung diseases or extrinsic factors such as obesity or neuromuscular weakness. The volume of gas in the lung can be measured using two techniques: dilution and plethysmography.
Dilutional techniques use the principle of conservation of mass. That is, the subject breathes through a mouthpiece connected to a closed system into which a known concentration of a marker gas (usually helium) is added. After several minutes to allow for equilibration, the final concentration of the marker gas is measured, permitting calculation of the volume added to the system (the patient’s lung volume). One notable limitation of dilutional techniques for measuring lung volumes is that significant obstruction in the airway results in underestimation of the true lung volume, because portions of the lung distal to the obstruction do not participate in the gas mixing and dilution.
Plethysmography uses the principle of Boyle’s law: in a closed system, pressure and volume change inversely when temperature is constant. With the subject sitting in a fixed-volume chamber (box) and breathing through a mouthpiece, a shutter is closed in the inspiratory limb of the breathing circuit. The subject makes small panting maneuvers, resulting in small changes in the volume of the lung and corresponding inverse-volume changes in the box. Pressures in the box and at the mouth are measured, which allows calculation of the lung volume when the panting efforts began. The subject usually begins the maneuvers at the end of a breath, and this “resting” lung volume is termed functional residual capacity (FRC). One notable limitation of the plethysmographic technique is that in the presence of significant obstruction, lung volume may be overestimated because one assumption of plethysmography—that there is no flow during the occlusion, and alveolar pressure and mouth pressure equilibrate—may not be true. Revised standards for plethysmography have been published.5
A lung capacity is the sum of two or more lung volumes; in the case of FRC, it is the sum of residual volume (RV; the amount of gas remaining in the lung after maximal exhalation) and expiratory reserve volume (ERV; the amount of gas exhaled from resting lung volume [FRC] until the lung cannot be emptied further). In combination with spirometry, other lung volumes and capacities can be calculated (Figure 146-2).
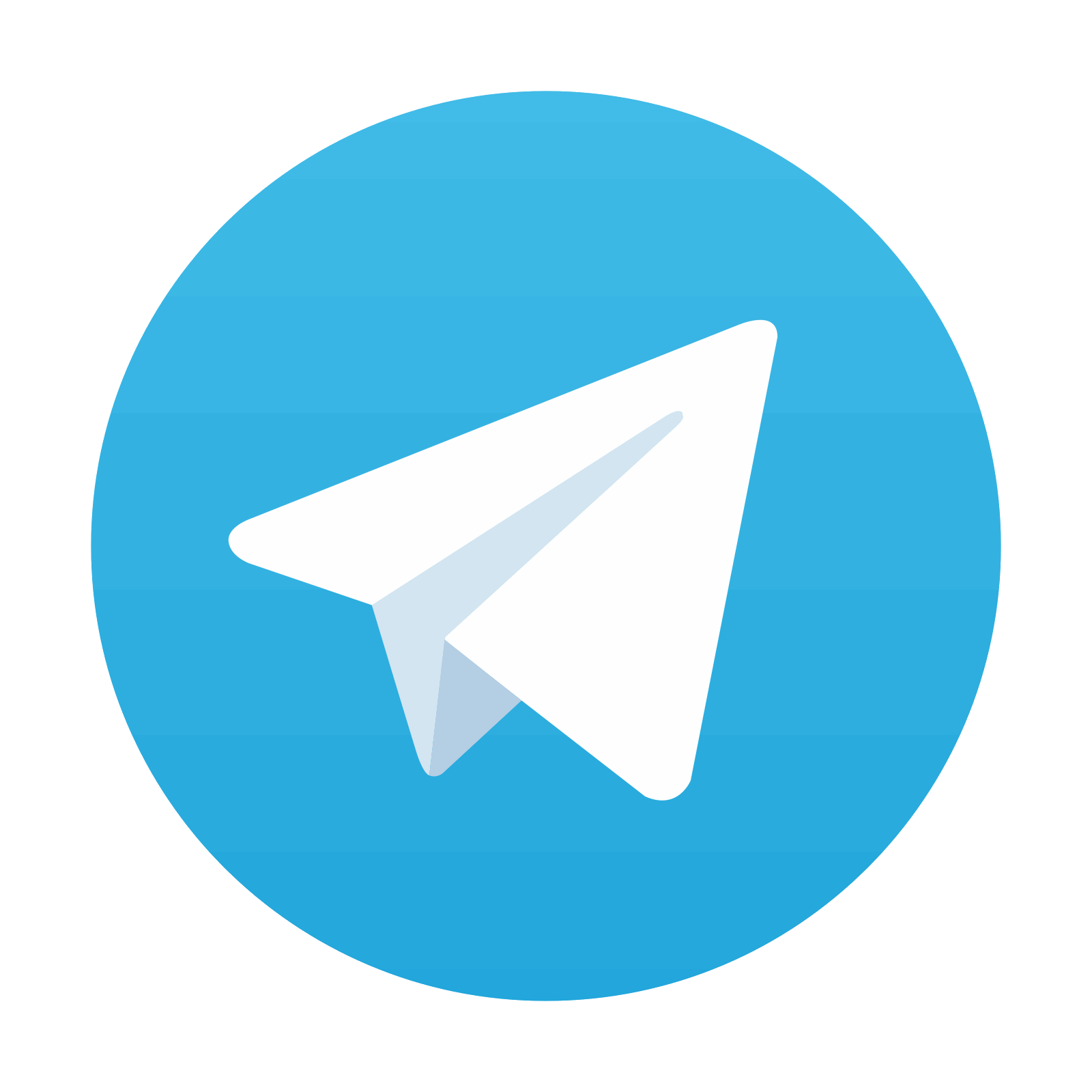
Stay updated, free articles. Join our Telegram channel

Full access? Get Clinical Tree
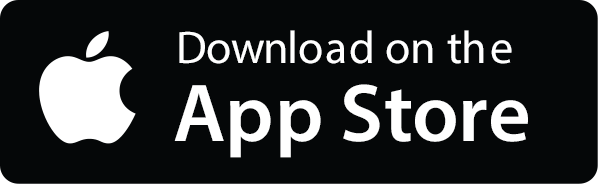
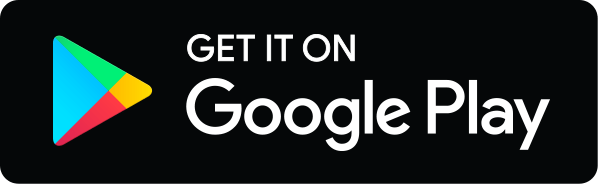