Principles of Neonatal Pharmacology
Jeffrey L. Blumer
Michael D. Reed
Introduction
The history of drug therapy is replete with examples of adverse reactions to drugs in children. Virtually all the drug-related legislation in effect in this country stems directly from these onerous experiences (1), which served as the foundation for the Pediatric Rule (1994), the Food and Drug Act, and, most recently, the Best Pharmaceuticals for Children Act (2).
In 1956, Silverman et al. (3) at Columbia reported an excessive mortality rate and an increased incidence of kernicterus among premature babies receiving a sulfonamide antibiotic compared with those receiving chlortetracycline. In 1959, Sutherland (4) described a syndrome of cardiovascular collapse in three newborns receiving high doses of chloramphenicol for presumed infections. More recently, the therapeutic misadventures experienced by low-birth-weight infants exposed to a parenteral vitamin E formulation (5) and the “gasping syndrome” in infants who received excessive amounts of benzyl alcohol (6,7) serve to underscore the generally held perception that newborn infants are more likely to experience adverse reactions to drugs.
This preconception is espoused even more ardently when the xenobiotic exposure occurs during the embryonic or fetal periods of development. The thalidomide tragedy reported in 1962, in which more than 10,000 babies were born with the rare malformation phocomelia, created an international outcry and changed forever the way in which drug safety is evaluated (8,9). More recently, therapeutic issues surrounding the retinoic acid embryopathy and maternal antidepressant drug use have refocused attention on the effects of drugs on the fetus and newborn (10).
As a result of these experiences, perinatologists, neonatologists, and pediatricians have become extremely conservative in their use of drug therapy. They have recognized that rational drug therapy for pregnant women and newborns is often confounded by a combination of unpredictable and often poorly understood pharmacokinetic and pharmacodynamic interactions (11,12,13,14). Although this conservative approach has permitted the fulfillment of the physician’s oath to “do no harm,” it also has prevented the adoption of newer therapeutic modalities and their adaptation to pediatric patients.
A more specific approach to neonatal therapeutics requires a thorough understanding of human developmental biology as well as insights regarding the dynamic ontogeny of the processes of drug absorption, drug distribution, drug metabolism, and drug excretion. In addition, there must be a rigorous appreciation of the developmental aspects of drug–receptor interactions, including the ontogenetic changes in receptor number, receptor affinity, receptor–effector coupling, and receptor modulation and regulation.
The purpose of this chapter is to develop a therapeutically relevant foundation for neonatal drug therapy based on the physiologic characteristics of extrauterine adaptation. The chapter focuses on the pharmacokinetic determinants of neonatal drug therapy rather than on any developmental variations in pharmacodynamics because only the former are amenable to clinical manipulation. Furthermore, specific discussion of the impact of growth on hepatic and renal function and the resultant important influences these ontogenic changes have on drug disposition and pediatric dosing are specifically addressed in Chapters 4 and 15, respectively, and thus are addressed only briefly here.
Drug Absorption
Absorption refers to the translocation of a drug from its site of administration into the bloodstream. Drugs administered extravascularly, including the sublingual, buccal, oral, intramuscular, subcutaneous, rectal, and topical routes, must cross multiple membranes to reach the systemic circulation and ultimately be distributed to sites of action. Absorption into the systemic circulation depends on both the physicochemical properties of a drug and a variety of host factors (Table 14.1).
Table 14.1 Factors Affecting Drug Absorption | |||||||||||||||
---|---|---|---|---|---|---|---|---|---|---|---|---|---|---|---|
|
Ontogeny of Gastric Acid Production
Hess (15) was the first to describe the presence of hydrochloric acid in the newborn stomach several hours after birth. At birth, gastric pH is usually between 6 and 8, but it falls rapidly to between 1.5 and 3.0 within several hours (16). From the available data, this fall in gastric pH is quite variable but appears to be independent of both birth weight and gestational age. Despite beliefs to the contrary, Kelly et al. (17) clearly demonstrated gastric acid secretion in preterm infants at 24 to 29 weeks of gestational age. All of the 22 preterm infants studied on multiple occasions (71 recordings) from 1 to 17 days postnatal life were able to produce and maintain an intragastric median pH below 4, with an inverse relationship between gestational age and initial acid production (17). Grahnquist et al. (18) described the presence of gastric H,K-adenosine triphosphatase in stomach biopsies from infants of 25 to 42 weeks’ gestation, with expression increasing with increasing gestational age.
Extrauterine factors (e.g., nutrition) are most likely responsible for initiating acid production, as basal acid output correlates with postnatal but not postconceptual age. The subsequent pattern of gastric acid secretion remains controversial. Initial descriptions suggested that, postnatally, acid secretion displays a biphasic pattern; the highest acid concentrations occur within the first 10 days and the lowest between the 10th and 30th day of extrauterine life. Agunod et al. (19), using betazole stimulation, essentially confirmed these early descriptions and found that the volume of gastric juice and acid concentration were dependent on age, and that gastric acid secretions (corrected for body weight) approached the lower limit of adult values by 3 months of age. Secretion of pepsin and intrinsic factor was also found to parallel that of gastric acid. In contrast, the longitudinal data of Kelly et al. (17) demonstrated a more constant amount of gastric acid (intragastric pH 0.6 to 3.9) over the first 17 days of life in very premature infants. Clearly, more study is necessary to fully elucidate the ontogeny of gastric acid secretion during the first month of life (18). However, these data also demonstrate the large degree of variability in the intragastric pH over the first 30 days of life and its dependence upon extrauterine factors.
Gastric and duodenal pH values affect drug solubility and ionization as well as gastrointestinal motility (20,21). An acid pH favors absorption of acid drugs (low pKa) because in such an environment, the drug will be largely in an unionized, more lipid-soluble form. In contrast, a relatively high pH (as in state of achlorhydria) will enhance the translocation of basic drugs and retard the absorption of acidic drugs.
Gastric Emptying
Because most orally administered drugs are absorbed in the small intestine, the rate of gastric emptying is an important determinant of the rate and extent of drug absorption. If it is slowed down, the rate of intestinal drug absorption may be reduced; this will, in turn, reduce the peak serum drug concentration. On the other hand, if gastric emptying is hastened, the extent of intestinal absorption may be reduced as a result of decreased contact time with the absorptive surface. Of course, both these effects presuppose that intestinal motility remains constant.
Gastric emptying rate during the neonatal period is variable and is characterized by irregular and unpredictable peristaltic activity (22,23,24). It is prolonged relative to that of the adult. The rate of gastric emptying appears to be directly affected by gestational and postnatal age as well as the type of feeding use (16,22,23,24) (Table 14.2). Gastric emptying time appears to approach adult values within the first 6 to 8 months of life. Similarly, small intestinal motility in the perinatal period is variable and is influenced by the presence or absence of food (23,25).
An inverse relationship between gestational age and the amount of gastric retention 30 minutes after a 5% glucose-in-water feeding has been demonstrated. This relationship was independent of intrauterine growth. With human milk feedings, a characteristic biphasic pattern of gastric emptying in both preterm and term infants, with an initial rapid phase followed by a slower prolonged second phase, is observed. In contrast, a liner pattern of gastric emptying was noted when infant formula was used (22,23).
Table 14.2 Factors Affecting Gastric Emptying Rate | ||||||||||||||||||
---|---|---|---|---|---|---|---|---|---|---|---|---|---|---|---|---|---|---|
|
Gastric emptying is also affected by the composition of the meal. Slower gastric emptying times have been reported with increasing caloric density in premature infants (gestational age 25 to 35 weeks). Significant differences were noted between formulas containing 0 and 6.5, 6.5 and 13, and 13 and 20 calories per 100 g. These differences were significant at all times following the meal (20, 40, 60, 80, and 100 minutes). The difference in gastric emptying between a 20- and a 24-calories/oz (71.5 and 86 calories per 100 g, respectively) formula was significant only at 80 minutes.
Similar findings with slower gastric emptying times have been reported in infants given a 10% glucose solution versus a 5% solution. Interestingly, although reduction in emptying rate is observed with higher caloric density substrates, the quantity of calories delivered to the duodenum from the stomach increased with increasing formula concentration (23).
Similar findings with slower gastric emptying times have been reported in infants given a 10% glucose solution versus a 5% solution. Interestingly, although reduction in emptying rate is observed with higher caloric density substrates, the quantity of calories delivered to the duodenum from the stomach increased with increasing formula concentration (23).
In contrast to the effect of caloric density on gastric emptying rates, the osmolality of the meal does not appear to be a factor. However, slower emptying is seen in feeding with long-chain fatty acids compared with medium-chain triglycerides, an important variable, considering that infant formulas differ in their fatty acid content (23).
Exocrine Pancreatic Function
The ontogeny of other physiologic processes may further influence the gastrointestinal absorption of drugs and other compounds. The rate of synthesis, pool size, and intestinal transport of bile acids are less in neonates than in adults (26), as is their character. At birth, pancreatic enzyme activity is low, and enzyme activities are lower in premature than in full-term neonates. Interestingly, at 1 week of postnatal age, fluid output and pancreatic enzyme activity are greater in premature than in full-term neonates. Lipase activity is present by 34 to 36 weeks of gestation, and increases fivefold during the first week and 20-fold during the first 9 months of postnatal life (27). In contrast, amylase activity has been detected as early as 23 weeks of gestation but remains very low even after birth (approximately 10% of adult values). Numerous investigators have shown decreased duodenal amylase activity in both fasting and fed infants during the first year of life. Trypsin secretion in response to pancreozymin and secretin administration is blunted in term infants but develops during the first year of life (16).
Duodenal contractions in term neonates appear to occur at rates similar to those observed in fasting adults, although the number of contractions per burst may be less (28). Fasting or interdigestive motor activity also appears to be shorter in children (29). A diagrammatic representation of the quantitative changes in small intestinal motility patterns in the human infant relative to postconceptional age is shown in Figure 14.1.
Phosphorylated-Glycoprotein
One area that has received considerable attention relative to drug absorption (and distribution) is the presence and functional capacity of phosphorylated-glycoprotein (P-Gp). In human, P-Gp is the most prominent member of the adenosine triphosphate–binding cassette family of proteins and is responsible for cellular drug efflux, translocating substances from the intracellular to the extracellular compartment (30,31). Initially considered to be responsible only for primary drug resistance in tumors (cellular resistance to “cancer” chemotherapeutic agents), subsequent studies confirmed that P-Gp is abundant within the human, and is normally found within the cellular membranes of the intestinal tract (duodenum, ileum, jejunum, and colon), apical membrane of heptocytes, renal proximal tubular cells, and on the luminal side of the capillary
endothelial cells that make up the blood-brain barrier (30,31,32). This efflux protein has affinity for a broad range of hydrophobic substrates, and effectively “pumps” xenobiotics out of cells, influencing the amount a drug may be absorbed into systemic circulation, influencing the rate at which a drug may be cleared by the liver or kidney, and influencing the amount of drug that enters the central nervous system (30,31,32,33). Moreover, the degree of expression and/or modulation of P-Gp activity by inhibitors or inducers is the probable basis for a number of clinically important drug–drug interactions. The well-described digoxin–quinidine/digoxin–verapamil interactions are most likely a result of the ability of quinidine and verapamil to antagonize the activity of intestinal and renal P-Gp activity.
endothelial cells that make up the blood-brain barrier (30,31,32). This efflux protein has affinity for a broad range of hydrophobic substrates, and effectively “pumps” xenobiotics out of cells, influencing the amount a drug may be absorbed into systemic circulation, influencing the rate at which a drug may be cleared by the liver or kidney, and influencing the amount of drug that enters the central nervous system (30,31,32,33). Moreover, the degree of expression and/or modulation of P-Gp activity by inhibitors or inducers is the probable basis for a number of clinically important drug–drug interactions. The well-described digoxin–quinidine/digoxin–verapamil interactions are most likely a result of the ability of quinidine and verapamil to antagonize the activity of intestinal and renal P-Gp activity.
The variability in intestinal absorption characteristics for many drugs is likely a direct result of the variability of P-Gp expression within the intestinal tract as well as the presence or absence of P-Gp modulators (30,31,34). Unfortunately, the ontogeny of P-Gp in any human organ is not described, and thus any influence P-Gp ontogeny has on drug absorption and distribution in the neonate, infant, and child remains to be elucidated. The importance of this cannot be overemphasized considering the important role that P-Gp plays in the absorption, distribution, and clearance of many clinically important drugs.
Additional Physiologic Processes
Colonization of the gastrointestinal tract by bacteria, a process that influences the metabolism of bile salts and drugs as well as intestinal motility, varies with age, type of delivery, type of feeding, and concurrent drug therapy (35,36,37). All full-term, formula-fed, vaginally delivered infants are colonized with anaerobic bacteria by 4 to 6 days of postnatal life. By 5 to 12 months of age, an adult pattern of microbial reduction products is established. Despite the description of these maturational changes, there are only limited data on the metabolic activity of the gut flora (36,37). For example, children are colonized with intestinal bacteria capable of metabolizing digoxin; the capacity to inactivate the drug appears to develop gradually with age (38).
The diseases that will most likely have the greatest impact on oral drug absorption are those affecting the total surface area available for absorption (39), such as the short-bowel syndrome (Table 14.3). This syndrome often results from massive bowel resection complicating necrotizing enterocolitis, from malrotation with volvulus, or from certain congenital anomalies of the gastrointestinal tract during the neonatal period. In addition, drug absorption may be altered during periods of protein–calorie malnutrition, a condition leading to loss of available surface area due to villous atrophy, delayed gastric emptying, and increased intestinal transit time (40,41).
Multiple systemic diseases may also affect gastrointestinal drug absorption. Congestive heart failure may cause mucosal edema, or it may, by means of various hemodynamic compensations, affect drug absorption by delaying gastric emptying or by shunting blood flow away from visceral organs to accommodate the metabolite needs of the heart and the brain. In addition, hypo- or hyperthyroidism may influence drug absorption by prolonging or reducing intestinal transit time, respectively. Table 14.3 summarizes the effects of specific intestinal and extraintestinal disease states on the processes involved in oral drug absorption.
Table 14.3 Selected Disease States Affecting Gastrointestinal Absorption of Drugs | ||||||||||||||||
---|---|---|---|---|---|---|---|---|---|---|---|---|---|---|---|---|
|
Drug Absorption Following Parenteral Administration
The parenteral route of drug administration is important when oral therapy is physiologically precluded or the bioavailability of an oral formulation is poor. The intravenous route for drug delivery is preferred over intramuscular injection. In the absence of cardiovascular decompensation, intramuscular injection then becomes a viable and effective alternative for the administration of many drugs (Table 14.4). In addition to the ontogenetic factors controlling absorption addressed previously, most extravascular routes share physiochemical and physiologic constraints (Table 14.5) that may affect the rate and/or extent of drug bioavailability. The following subsections highlight pharmacokinetic considerations of several nonoral extravascular routes of administration.
Absorption of Drugs Given Intramuscularly
Curves of serum concentration versus time following intramuscular drug administration may depend on factors germane to the drug, the site of administration, the presence of concomitant pathophysiology, and/or the developmental status of the patient (Table 14.5). Both physicochemical and physiologic factors affect the rate of drug absorption from the injection site (42). Lipophilicity of a drug favors rapid diffusion into the capillaries; however, the drug must retain a degree of water solubility at physiologic pH to prevent precipitation at the injection site (Table 14.4).
Another important factor influencing absorption of drugs from an intramuscular injection site is the blood flow to and from the injection site. This may be compromised in
newborns with poor peripheral perfusion from low-cardiac-output states or the respiratory distress syndrome (43). The rate and extent of absorption from an intramuscular injection site are also influenced by the total surface area of muscle coming into contact with the injected solution (18), similar to the dependence of oral absorption on the total available absorptive area in the intestines. The ratio of skeletal muscle mass to body mass is less for neonates than for adults (43).
newborns with poor peripheral perfusion from low-cardiac-output states or the respiratory distress syndrome (43). The rate and extent of absorption from an intramuscular injection site are also influenced by the total surface area of muscle coming into contact with the injected solution (18), similar to the dependence of oral absorption on the total available absorptive area in the intestines. The ratio of skeletal muscle mass to body mass is less for neonates than for adults (43).
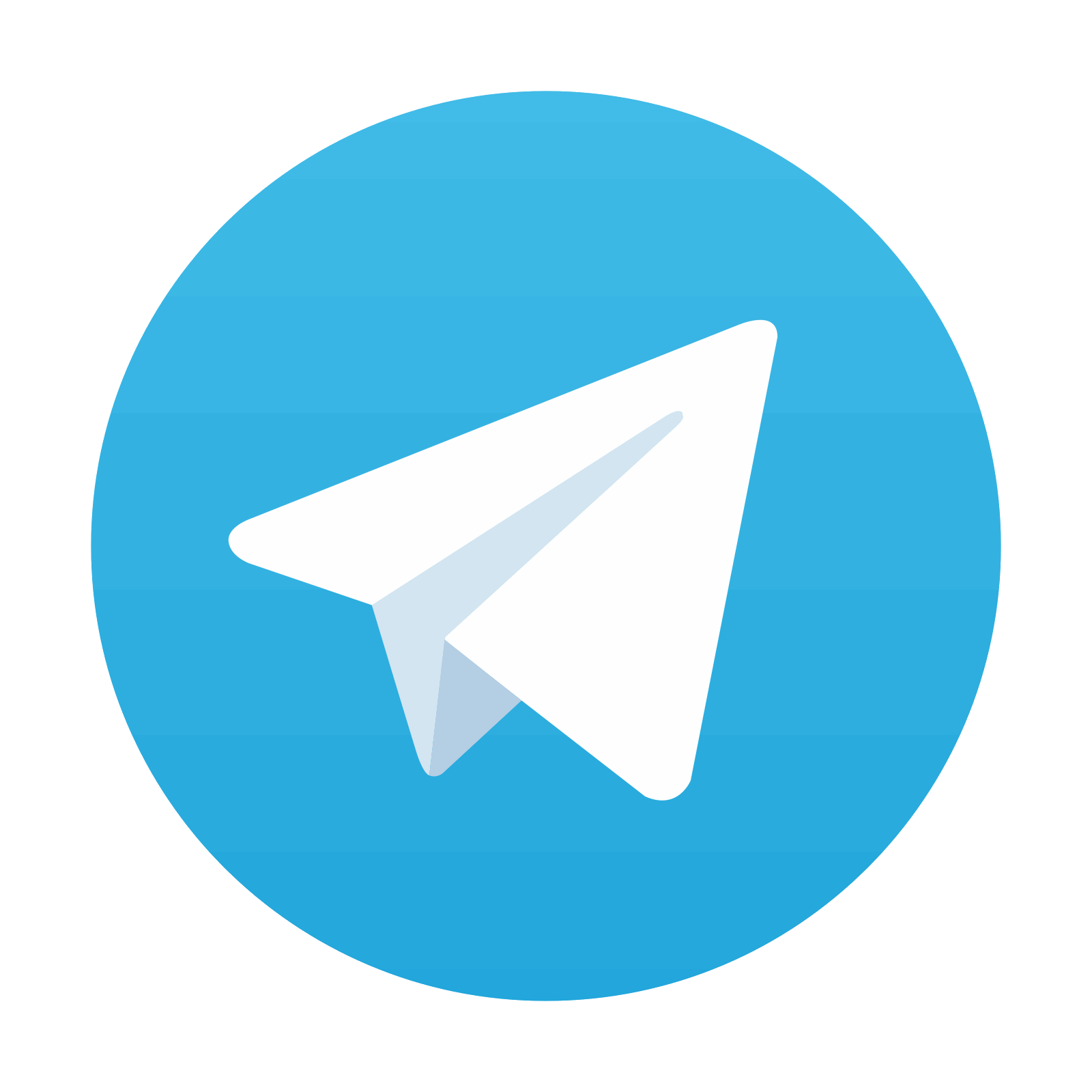
Stay updated, free articles. Join our Telegram channel

Full access? Get Clinical Tree
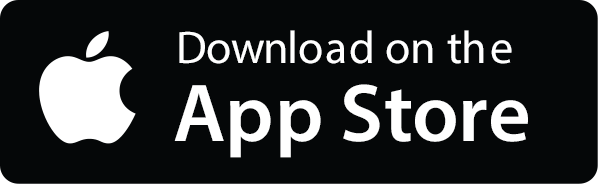
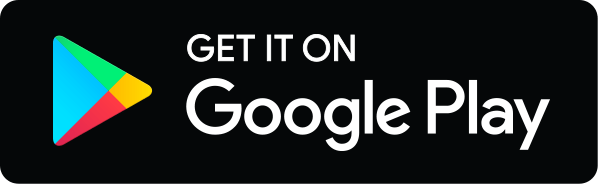