Objective
We sought to investigate the effects of antenatal retinoic acid on the pulmonary vasculature and vascular endothelial growth factor (VEGF) and VEGF receptors (VEGFR) expression in a nitrofen-induced congenital diaphragmatic hernia (CDH) model.
Study Design
Rat fetuses were exposed to nitrofen at gestational day 9.5 and/or all- trans retinoic acid (ATRA) at gestational days 18.5-20.5. We assessed lung growth, airway, and vascular morphometry. VEGF, VEGFR1, and VEGFR2 expression was analyzed by Western blotting and immunohistochemistry. Continuous data were analyzed by analysis of variance and Kruskal-Wallis test.
Results
CDH decreased lung to body weight ratio, increased mean linear intercept and mean transection length/airspace, and decreased mean airspace cord length. ATRA did not affect lung growth or morphometry. CDH increased proportional medial wall thickness of arterioles while ATRA reduced it. ATRA recovered expression of VEGF and receptors, which were reduced in CDH.
Conclusion
Retinoic acid and VEGF may provide pathways for preventing pulmonary hypertension in CDH.
Congenital diaphragmatic hernia (CDH) is a defect of the diaphragm associated with a high mortality, which is increased among newborns with associated malformations or who require extracorporeal membrane oxygenation. Persistent pulmonary hypertension of the newborn is a frequent complication associated with mortality in newborns with CDH and a possible indication for extracorporeal membrane oxygenation. Optimal treatment of newborns with CDH would involve either prevention of the defect or prenatal reversal of the pulmonary structural and functional abnormalities, especially pulmonary hypertension and hypoplasia. This strategy is, however, limited by the current knowledge of the etiology of CDH and the molecular mechanisms that result in the functional abnormalities.
Even though the etiology of CDH is still unclear, increasing evidence points toward a disruption of the retinoic acid pathway. Litters from vitamin A–deficient rats have a 60% frequency of CDH and this rate is decreased by reintroduction of vitamin A early during gestation. Nitrofen, a teratogen that induces CDH in animals, not only interferes with the retinoic acid synthesis pathway but also the incidence of CDH in fetuses exposed to nitrofen decreases with vitamin A or retinoic acid treatment. More recently, silencing of genes involved in retinoic acid signaling, such as the genes for retinoic acid receptors, chicken ovalbumin upstream promoter-transcription factor (COUP-TFII), GATA4, and friend of GATA (Fog2), cause CDH and genes located in cytogenetical hotspots for CDH are also implicated in the same pathway. In human beings, low levels of retinoids have been reported in newborns with CDH.
Retinoic acid is important not only for the development of the diaphragm but also for development of the lung. It is critical for septation and alveolarization, which can be severely compromised in CDH. Prenatal treatment with retinoids has been shown to improve lung growth and development in the nitrofen-induced CDH rat model when administered up to the 13th gestational day (GD). However, the effects of retinoic acid in the vascular development of the lung are unknown. Since retinoic acid is one of the main regulators of angiogenesis and vasculogenesis it is likely to be an important element in vascularization of the lung. In CDH, disruption of vascular growth is seen as an underdevelopment of the vascular bed and thickening of the medial muscular layer of pulmonary arterioles that is directly proportional to the severity of pulmonary hypertension.
It is not clear why these histologic and functional changes take place. Vascular endothelial growth factor (VEGF) is one of the most important vascular growth factors and the isoform VEGF-A is not only essential to angiogenesis but also to survival of recently formed vessels. The VEGF receptors (VEGFR) VEGFR1 (FMS-like tyrosine kinase-1 [flt-1]) and VEGFR2 (fetal liver kinase-1 [flk-1] in rats, kinase insert domain receptor [KDR] in human beings) are responsible for signaling different intracellular effects. VEGFR1 has a higher affinity for VEGF-A but a lower tyrosine-kinase activity. In early embryonic stages, VEGFR1 is an important negative regulator of angiogenesis. On the other hand, VEGFR2 has lower affinity but higher tyrosine-kinase activity and is essential for vascular growth from early embryonic stages and is the first to be expressed in the embryo. In fact, silencing of VEGFR2 gene leads to early embryonic death due to severe deficiency in vascular formation. In this sense, not only adequate expression of VEGF but also appropriate balance of expression of VEGF receptors is likely required for normal vascular development.
Even though the importance of VEGF for normal vascular development is well established, its role in the pathogenesis of CDH is unclear. An increased VEGF expression has been observed in the postmortem examination of lungs from newborns with CDH. In animal models, the results have been conflicting with an increased expression of VEGF reported in one study with the nitrofen-induced CDH rat model, whereas other authors have reported a decrease in the expression of this protein in the same model. Few authors have looked at the expression of both VEGF and its receptors. While Hara et al have observed no change in the expression of the receptors, we have previously reported a decrease in the expression of VEGFR1 and VEGFR2 in the nitrofen-induced CDH rat model.
Considering the possible involvement of VEGF signaling in lung vascularization in CDH and the strong relationship between retinoic acid and angiogenesis we hypothesized that antenatal retinoic acid treatment in the nitrofen-induced CDH rat model would reverse epithelial and vascular abnormalities of the lung in CDH as well as recover the expression of VEGF and its receptors.
Materials and Methods
This study was submitted to and approved by the Animal Experimentation Ethics Committee of University of Campinas (research project no. 935-1).
Study groups
Pregnant Sprague-Dawley rats were divided into 6 groups that originated 8 fetal groups with 25 fetuses each:
- 1
External control: nonmanipulated.
- 2
Placebo nitrofen: received 1 mL of olive oil by gavage at GD 9.5.
- 3
Placebo retinoic acid: received 1 mL of olive oil intraperitoneally at GD 18.5, 19.5, and 20.5.
- 4
Nitrofen: Received nitrofen as described below. Fetuses were divided in 2 groups:
- a
Without CDH (N–).
- b
With CDH (N+).
- a
- 5
All- trans retinoic acid (ATRA) control (AC): received ATRA.
- 6
Nitrofen + ATRA: received both nitrofen and ATRA as described below. Fetuses were divided into 2 groups:
- a
Without CDH (NA–).
- b
With CDH (NA+).
- a
Nitrofen
Pregnant rats were given 100 mg of nitrofen (2,4-dichloro-4′-nitrodiphenyl ether; Maybridge, Cambridge, United Kingdom) dissolved in 1 mL of olive oil at GD 9.5 by gavage. This dosage of nitrofen causes left-sided CDH in 42% of the litter. During sample collection the presence of CDH was verified by direct visualization of the diaphragm by careful dissection of the abdominal wall and removal of the abdominal organs.
ATRA
Pregnant rats were given 5 mg/kg/d of ATRA dissolved in 1 mL of olive oil at GD 18.5, 19.5, and 20.5 intraperitoneally.
Samples
Fetuses were harvested at term (GD 21), weighted, and dissected. From each dam we obtained samples for morphometric, immunohistochemical, and Western blotting analysis. We measured body weight (BW), left lung weight (LLW), total lung weight (TLW), and lung to BW ratio (LBWR). Left lungs were fixated with formaldehyde 10% for at least 24 hours prior to fixation.
Airway measurements
Histologic sections were examined under light microscope at ×100 magnification. We measured the mean linear intercept (Lm) and its components: mean transection length/airspace and mean airspace cord length (Lma). Transection length/airspace translates the thickness of the septal walls, while Lma refers to the internal airspace diameter. Sections were randomly selected and sections from 5 different animals per group were analyzed by blinded investigators.
Vascular measurements
Histologic sections were analyzed under light microscope at ×200 magnification. Preacinar resistance arterioles between 30-60 μm were included. We measured the external diameter (ED), internal diameter, and proportional medial wall thickness (MWT) by the formula: MWT = (ED – internal diameter)/ED. This proportion eliminates the effects of vasodilation, constriction, or sample fixation over the morphometric variables. Vascular measurements were obtained from 42 different vessels from 5 different animals per group and performed by blinded investigators.
Immunohistochemistry
Left lungs were fixated in formaldehyde 10%, dehydrated, diaphonized, and embedded in paraffin. Then, 5-μm slices were mounted on slides. Slides were treated with sodium borate (0.1 mol/L, pH 7.4) for 1 hour at room temperature, followed by sodium citrate (0.01 mol/L, pH 6.0) for antigen exposition. Then they were treated with 1% hydrogen peroxide and washed in phosphate-buffered saline (PBS) (0.01 mol/L, pH 7.4). Sections were incubated with blocking solution of bovine serum albumin (BSA) 1% for 1 hour at room temperature to block nonspecific binding sites. Sections were incubated with anti-VEGF diluted 1:100 in 0.1% PBS, or anti-flt-1 (VEGFR1) diluted 1:100 in 0.1% PBS, or anti-flk-1 (VEGFR2) diluted 1:25 in 0.1% PBS (sc-7269, sc-316, or sc-6251, respectively; Santa Cruz Biotechnology, Santa Cruz, CA) at 4°C overnight. After washing, sections were incubated with biotin-conjugated antirabbit IgG (1:200 in 1% BSA) for 1 hour at room temperature for VEGFR1 and with biotin-conjugated antimouse IgG (1:200 in 1% BSA) for 2 hours for VEGF and VEGFR2. As negative controls we omitted the primary antibody. For visualization of histochemical reaction we used the Vectastain ABC kit (Vector Laboratories, Burlingame, CA) and 3,3′-diaminobenzidine tetrahydrochloride hydrated (DAB; Sigma-Aldrich, St. Louis, MO). Sections were counterstained with Harris hematoxylin and mounted.
Semiquantitative analysis
Immunohistochemistry sections were analyzed by 2 independent blinded researchers (9 sections per group). Sections were graded according to the intensity of staining from 0-4: 0 = negative, 1 = weak staining, 2 = moderate staining, 3 = strong staining, 4 = very strong staining.
Western blotting
Left lungs from 6 animals per group were homogenized in 1 mL per lung of extraction buffer containing (mM): Tris 100 (pH 7.4), sodium pyrophosphate 100, sodium fluoride 100, EDTA 10, sodium vanadate 10, and phenylmethylsulfonyl fluoride 2, and 0.1 mg aprotinin ml−1 and 1% Triton-X100 at 4°C with a tissue homogenizer (Tecnal, Paulinia, Brazil) operated at maximum speed for 30 seconds. The extracts were centrifuged at 14,000 rpm (9000 g) at 4°C in a Mikro 200R centrifuge (Hettich, Kirchelengern, Germany) for 30 minutes to remove insoluble material, and the supernatants of these tissues were used for protein quantification using the Bradford method. Then proteins were denaturated, run on sodium dodecyl sulfate polyacrylamide gel electrophoresis, and transferred to nitrocellulose membranes. The membranes were blocked for 1 hour in 1% BSA solution and incubated with anti-VEGF, anti-flt-1 (VEGFR1) diluted 1:200 in 0.1% PBS, or anti-flk-1 (VEGFR2) diluted 1:200 in 0.1% PBS (sc-7269, sc-316, and sc-6251, respectively) at 4°C overnight. The following day, after washing, sections were incubated with biotin-conjugated antirabbit IgG (1:10,000 in 1% BSA) for 2 hours at room temperature for VEGFR1 and with biotin-conjugated antimouse IgG (1:5000 in 1% BSA) for 2 hours for VEGFR2. Afterward, membranes were probed with Supersignal Chemiluminescence kit (Pierce, Rockford, IL), exposed to radiographic films (Kodak, Rochester, NY) and developed.
Statistical analysis
Morphometrical data was compared by analysis of variance with Tukey posttest. Immunohistochemistry staining scores were analyzed by Kruskal-Wallis with Dunn posttest. A P value < .05 was considered significant. Western blotting results were analyzed by optical density of the bands and compared by analysis of variance with Tukey posttest.
Results
Litters from 21 pregnant rats were included in the study. There was no maternal mortality associated with administration of ATRA. Among fetuses alive at term that were exposed to nitrofen 40% (50/126) had CDH.
Exposure to nitrofen significantly reduced BW ( P < .05), which was not further affected by the presence or absence of CDH. Exposure to nitrofen also significantly reduced TLW, LLW, and LBWR ( P < .05). Among fetuses exposed to nitrofen, those with CDH had reduced TLW, LLW, and LBWR compared to those without CDH ( P < .05) ATRA did not affect BW, TLW, LLW, or LBWR in animals with or without CDH ( Table 1 ).
Variable | BW, mg | TLW, mg | LLW, mg | LBWR |
---|---|---|---|---|
EC | 6003 ± 950 | 131 ± 24 | 44 ± 7.7 | 0.022 ± 0.004 |
PN | 6039 ± 442 | 159 ± 23 | 54 ± 8.1 | 0.027 ± 0.002 |
PA | 6201 ± 395 | 160 ± 22 | 50 ± 5.9 | 0.025 ± 0.002 |
AC | 6230 ± 910 | 151 ± 27 | 49 ± 7.6 | 0.024 ± 0.002 |
N– | 5423 ± 373 | 104 ± 17 | 37 ± 5.7 | 0.020 ± 0.003 |
N+ a | 5526 ± 467 | 84 ± 12 | 25 ± 6.1 | 0.015 ± 0.002 |
NA– | 5040 ± 342 | 103 ± 21 | 36 ± 6.6 | 0.020 ± 0.003 |
NA+ a | 5010 ± 378 | 73 ± 12 | 23 ± 4.1 | 0.015 ± 0.002 |
a P < .05 with lower TLW, LLW, and LBWR on animals with congenital diaphragmatic hernia compared to all other groups, including animals exposed to nitrofen without congenital diaphragmatic hernia.
Airway measurements
The presence of CDH significantly increased the Lm and the mean transection length/airspace, while decreasing the Lma compared to fetuses without CDH, including fetuses exposed to nitrofen without CDH ( P < .001). Prenatal treatment with retinoic acid did not affect airway morphometry ( Table 2 ).
Variable | Lm | Lmw | Lma |
---|---|---|---|
EC | 87.7 ± 22.62 | 62.9 ± 20.16 | 24.7 ± 6.46 |
PN | 92.9 ± 15.30 | 64.5 ± 13.58 | 27.5 ± 14.29 |
PA | 87.2 ± 15.80 | 63.3 ± 11.58 | 23.9 ± 10.68 |
AC | 76.2 ± 14.03 | 51.1 ± 10.66 | 25.1 ± 8.29 |
N– | 87.4 ± 19.70 | 66.1 ± 20.19 | 21.3 ± 5.97 |
N+ a | 135.5 ± 66.70 | 117.1 ± 66.7 | 18.5 ± 6.93 |
NA– | 94.1 ± 21.97 | 73.4 ± 21.29 | 22.1 ± 5.80 |
NA+ a | 147.6 ± 67.3 | 132.6 ± 66.58 | 15.0 ± 3.99 |
Vascular measurements
Fetuses with CDH (group N+) had increased MWT compared to fetuses without CDH, whether exposed to nitrofen or not ( P < .001). However, fetuses with CDH that were treated with ATRA (group NA+) had a MWT similar to fetuses without CDH and decreased compared to fetuses with CDH ( P < .001) ( Figure 1 ).

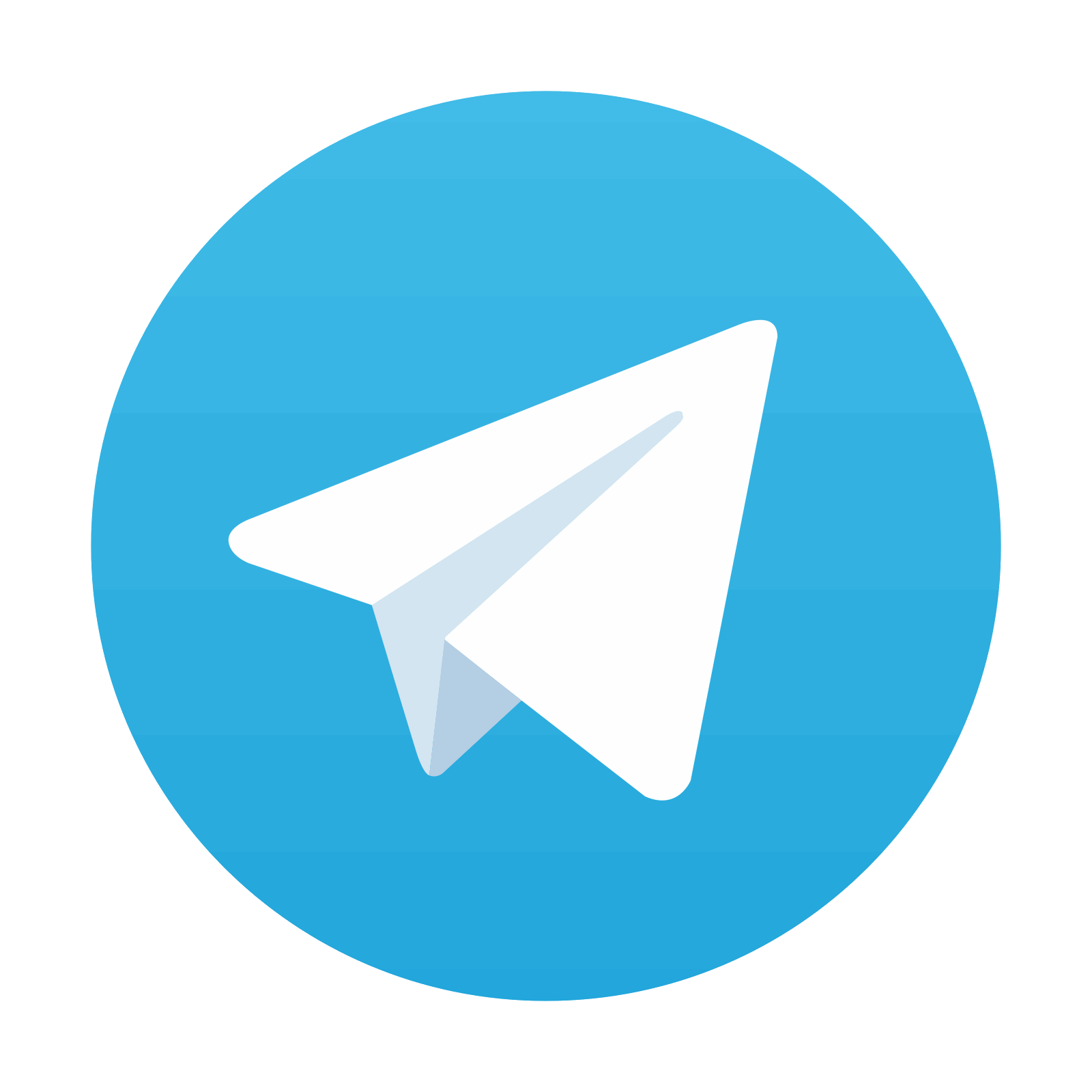
Stay updated, free articles. Join our Telegram channel

Full access? Get Clinical Tree
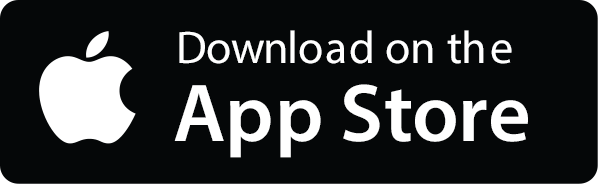
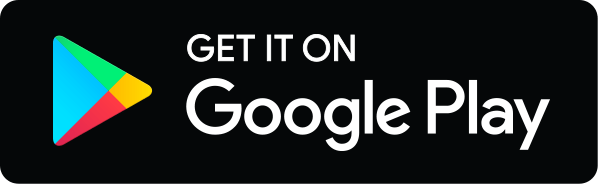