Key Points
Prenatal diagnostic procedures allow prenatal detection of an ever-expanding list of fetal abnormalities by obtaining genetic, biochemical, and physiologic information about the fetus.
The specific procedure selected depends upon the gestational age and the information needed.
Genetic consultation should be available to help patients choose which diagnostic procedure is optimal for them.
Earlier prenatal diagnostic methods have become increasingly more common and are likely to increase in popularity. The most studied of these is CVS. Transcervical and transabdominal techniques are equally effective.
Early amniocentesis is not recommended now that compelling evidence regarding its disadvantages has been published.
Advances in molecular techniques have led to a declining number of reasons for using percutaneous umbilical blood sampling. It is now rarely the procedure chosen for determining fetal karyotype.
Percutaneous umbilical blood sampling is the most direct method of evaluating fetal anemia secondary to severe Rho(D) disease.
The majority of invasive diagnostic procedures are associated with low rates of complications commensurate with provider skill and experience.
Amniocentesis was first used during the 1880s for decompression of polyhydramnios (Lambl, 1881). In 1930, placental localization was achieved after the intra-amniotic injection of contrast medium (Menees et al., 1930). Aburel (1937) described the termination of a pregnancy by the intra-amniotic injection of hypertonic saline. During the 1950s, the role of amniocentesis and measurement of bilirubin concentrations in monitoring rhesus disease was reported (Bevis, 1950; Walker, 1957).
Amniocentesis for fetal chromosome analysis was also initiated in the 1950s. (Until 1956, the number of human chromosomes was not known.) The first reported application was for fetal sex determination (Fuchs and Riis, 1956). The feasibility of culturing and karyotyping amniotic fluid cells was demonstrated by Steele and Breg in 1966. The first prenatal diagnosis of an abnormal karyotype, a balanced translocation, was reported in 1967 by Jacobson and Barter. Trisomy 21 was detected prenatally by Valenti et al., in 1968. During the same year the first diagnosis of the metabolic disorder galactosemia was reported by Nadler (1968).
Indications for amniocentesis include (1) increased risk for fetal aneuploidy, (2) increased risk for known fetal genetic or biochemical abnormalities, (3) increased risk for fetal open neural tube defect (ONTD), (4) evaluation for fetal infection, and (5) documentation of fetal lung maturity.
In the United States, while it had been considered standard practice to offer genetic counseling and prenatal cytogenetic analysis to all women of advanced maternal age, (age 35 years or older at their expected date of delivery), these recommendations have changed. Most recent guidelines from the American College of Obstetricians and Gynecologists emphasize that all patients, regardless of age, should have the option for invasive prenatal cytogenetic analysis if they prefer (ACOG, 2007). It is no longer considered acceptable clinical practice to limit or recommend invasive testing of fetal karyotype purely on the basis of maternal age. Amniocentesis is the most common invasive test used for this indication. The risk of numerical chromosomal abnormalities (aneuploidy) increases with advancing maternal age as a result of nondisjunction, which occurs during maternal meiosis. The relationship between maternal age and the estimated risk of chromosomal abnormalities is shown in Table 4-1 (Hook, 1981; Hook et al., 1983). It is now also standard practice to offer invasive prenatal diagnosis to patients with increased risk for aneuploidy according to the results of first and/or second trimester screening for aneuploidy (see Chapters 2 and 3 for more details on the various methods of maternal screening for aneuploidy).
Age | Risk of Down Syndrome | Risk of Chromosomal Abnormality |
20 | 1/1667 | 1/526 |
25 | 1/1250 | 1/476 |
30 | 1/952 | 1/385 |
35 | 1/385 | 1/202 |
36 | 1 295 | 1/162 |
37 | 1/227 | 1/129 |
38 | 1/175 | 1/102 |
39 | 1/137 | 1/82 |
40 | 1/106 | 1/65 |
41 | 1/82 | 1/51 |
42 | 1/64 | 1/40 |
43 | 1/50 | 1/32 |
44 | 1/38 | 1/25 |
45 | 1/30 | 1/20 |
46 | 1/23 | 1/16 |
47 | 1/18 | 1/13 |
48 | 1/14 | 1/10 |
49 | 1/11 | 1/7 |
Similar to patients with singletons, invasive prenatal diagnosis should be considered in all patients with multiple fetuses with an increased risk for aneuploidy (Meyers et al., 1997; American Academy of Pediatrics and The American College of Obstetricians and Gynecologists, 2002; The American College of Obstetricians and Gynecologists, 2004).
Invasive prenatal diagnosis is also indicated when there is a need to obtain fetal material for cytogenetic, biochemical, or DNA studies. Increasingly, the DNA abnormalities responsible for the etiology of many disorders are being identified. A list of some of the common genetic conditions for which DNA-based prenatal diagnosis is available is given in Table 4-2, although this list is far from complete. More than 100 abnormalities of lipid, mucopolysaccharide, amino acid, and carbohydrate metabolism are also amenable to prenatal diagnosis through the biochemical analyses of cultured amniotic fluid cells. Note, however, that the substantially larger amount of tissue obtained by chorionic villus sampling (CVS) makes CVS the preferred method of diagnosing single gene biochemical disorders in which the DNA abnormality is known.
Disorder | Mode of Inheritance | Chromosome | Disorder | Mode of Inheritance | Chromosome |
α1-Antitrypsin deficiency | AR | 14 | Marfan syndrome | AD | 15 |
α1-Thalassemia | AR | 16 | Multiple endocrine neoplasia type I | AD | 11 |
Adult polycystic kidney disease (type 1) | AD | 16 | Multiple endocrine neoplasia type IIA | AD | 10 |
β -Thalassemia | AR | 11 | Myotonic dystrophy | AD | 19 |
Congenital adrenal hyperplasia | AR | 6 | Neurofibromatosis (type 1) | AD | 17 |
Cystic fibrosis | AR | 7 | Neurofibromatosis (type 2) | AD | 22 |
DiGeorge syndrome | AD | 22 | Norrie disease | XLR | X |
Duchenne/Becker muscular dystrophy | XLR | X | Ornithine transcarbamylase deficiency | XLR | X |
Familial Alzheimer disease | AD | 21 | Phenylketonuria | AR | 12 |
Familial hypercholesterolemia | AD | 19 | Retinoblastoma | AD | 13 |
Familial polyposis coli | AD | 5 | Sickle cell anemia | AR | 11 |
Fragile X syndrome | XLR | X | Spinal muscular atrophy | AR/AD | 5 |
Gardner syndrome | AD | 5 | Tay-Sachs disease | AR | 5 |
Hemoglobin Sc | AR | 11 | von Hippel–Lindau syndrome | AD | 3 |
Hemophilia A (factor IX deficiency) | XLR | X | Wiskott–Aldrich syndrome | XLR | X |
Huntington disease | AD | 4 |
Established screening tests for fetal ONTDs include measurement of maternal serum alpha fetoprotein levels (MSAFP) during the second trimester, followed by amniocentesis in patients with elevated results (UK Collaborative Study, 1979), or sonographic screening for brain and spine malformations. The ultrasound diagnosis of fetal ONTD has been greatly enhanced by the recognition of associated abnormalities in the skull and brain. These abnormalities include cerebral ventriculomegaly, microcephaly, frontal bone scalloping (lemon sign), and obliteration of the cisterna magna with either an “absent” cerebellum or abnormal posterior curvature of the cerebellar hemispheres (banana sign) (see Chapter 19) (Nicolaides et al., 1986). Van den Hof et al. (1990) have reported on the diagnosis of ONTD in 130 fetuses among 1561 patients at high risk for fetal neural tube defects who were referred for detailed ultrasound examination. The examinations revealed associated abnormalities of the skull and brain in 129 of the 130 fetuses with ONTD. As a result of this evidence of the accuracy of ultrasound in the diagnosis of neural tube defects, the need for amniocentesis in the evaluation of an elevated MSAFP has been questioned.
Many centers no longer offer either stand-alone MSAFP screening or amniocentesis for an elevated MSAFP level, given the significant advances in obstetric sonographic precision for this abnormality. At centers adept at diagnosing ONTD sonographically, amniocentesis can be reserved for patients with suspicious ultrasound findings or large MSAFP elevations despite a normal scan or when it is impossible to adequately visualize fetal anatomy. At such specialized centers, patients can be counseled that with a normal ultrasound examination, in which optimal views are obtained of the fetal head and spine, the risk of ONTD can be reduced by up to 95%, and therefore patients may elect not to undergo amniocentesis (American College of Obstetricians and Gynecologists, 1996). Patients who are at increased risk for neural tube defects (i.e., who have elevated MSAFP levels), who have a history of neural tube defects, or who are taking antifolate medications should be referred to centers with ultrasonographers experienced in diagnostic ultrasound. If ultrasound examination demonstrates a normal spine, cranium, and cerebellum, the chance of an undetected spinal abnormality is low. Therefore, amniocentesis, with a procedure-related risk of 0.1% up to 1%, is probably unnecessary. Some centers have calculated a revised risk of neural tube defects on the basis of the MSAFP value and normal results on ultrasound examination at their particular center (Richards et al., 1988; Nadel et al., 1990). For example, in our reference laboratory in a Caucasian patient with an a priori risk for ONTD of 1 in 1000, an MSAFP of 2.8 multiples of the median (MoM) gives a risk of 1 in 170. When an adequate ultrasound examination is performed by competent ultrasonographers and is interpreted as a normal scan, this risk may be reduced by 95%, giving a reassigned risk of approximately 1 in 3400. In our center, amniocentesis is reserved for cases in which there is incomplete visualization of the fetus (as a result of maternal obesity or fetal position), serum levels of MSAFP greater than 3.5 MoM in the presence of normal sonographic examination, or suspicious ultrasound findings.
Premature delivery is one of the leading causes of perinatal death and long-term handicap. The association between intrauterine infection and premature labor, in the presence and absence of ruptured membranes, has stimulated research into amniocentesis for the diagnosis of subclinical intrauterine infection (Romero et al., 1989; Shim et al., 2004). Amniocentesis in the clinical management of preterm labor or preterm premature rupture of membranes (PPROM) may be utilized for cases in which there is a suspicion of intrauterine infection.
Measurement of the amniotic fluid lecithin: sphingomyelin ratio (Gluck et al., 1974) and phosphatidylglycerol (Hallman et al., 1976) level is useful for the assessment of fetal lung maturity. The need for these tests has declined because of improved neonatal care and the introduction of better methods of ultrasound assessment of gestational age and fetal surveillance. Elective preterm delivery should only be performed for a significant maternal or fetal indication. Nonetheless, amniocentesis in the third trimester is a safe procedure (Hodor et al., 2006).
Assessment of the severity of Rh disease and the need for fetal blood transfusion or early delivery had previously been based on the results of amniocentesis and the interpretation of the results of spectrophotometric estimation of amniotic fluid bilirubin (Liley, 1961). However, Doppler measurements of the middle cerebral artery peak systolic velocity have now replaced amniocentesis for this indication. MCA Dopplers can now be used to noninvasively diagnose anemia in fetuses without hydrops who are at risk for anemia secondary to conditions such as Rho(D) sensitization and parvovirus B19 infection (Mari et al., 2000; Cosmi et al., 2002) (Figure 4-1). This noninvasive method of detecting fetal anemia decreases the number of amniocenteses and percutaneous umbilical blood sampling (PUBS) procedures needed in these high-risk patients. The value of the middle cerebral artery peak systolic velocity is expressed as MoM. Middle cerebral artery peak systolic velocity >1.50 MoM is considered to be suggestive of anemia and is considered an indication for PUBS (Table 4-3). In the assessment of severe Rh sensitization in the midtrimester, the only accurate method for predicting the severity of the disease is measurement of the hemoglobin concentration.
Gestational Age | 1.00 median | 1.29 | 1.50 | 1.55 |
18 | 23.2 | 29.9 | 34.8 | 36.0 |
20 | 25.5 | 32.8 | 38.2 | 39.5 |
22 | 27.9 | 36.0 | 41.9 | 43.3 |
24 | 30.7 | 39.5 | 46.0 | 47.5 |
26 | 33.6 | 43.3 | 50.4 | 52.1 |
28 | 36.9 | 47.6 | 55.4 | 57.2 |
30 | 40.5 | 52.2 | 60.7 | 62.8 |
32 | 44.4 | 57.3 | 66.6 | 68.9 |
34 | 48.7 | 62.9 | 73.1 | 75.6 |
36 | 53.5 | 69.0 | 80.2 | 82.9 |
38 | 58.7 | 75.7 | 88.0 | 91.0 |
40 | 64.4 | 83.0 | 96.6 | 99.8 |
Early attempts at genetic amniocentesis were made transvaginally. Subsequently, the transabdominal approach has been shown to be the only acceptable approach. During the 1960s amniocentesis was performed “blindly.” During the 1970s and early 1980s, ultrasonography was used to identify a placenta-free area for entry into a pocket of amniotic fluid. This position was marked on the maternal abdomen, and after a variable length of time, the operator would blindly insert the needle.
In contemporary practice, amniocentesis is always performed with continuous ultrasound guidance. An ultrasound scan is first performed to determine the number of fetuses present, to confirm gestational age and fetal viability, and to document normal anatomy. The maternal abdomen is washed with antiseptic solution; it is unnecessary for the technician to scrub and gown. Continuously guided by ultrasound, a 22-gauge needle is introduced into the amniotic cavity (Figures 4-2A and 4-2B). While the procedure may be performed either freehand or with needle guides, the vast majority of centers currently use a freehand technique (Jeanty et al., 1983; Lenke et al., 1985). The freehand technique is generally preferred because it allows easier manipulation of the needle if the position of the target is abruptly altered by a uterine contraction or fetal movement. Furthermore, this technique can be easily adapted to all ultrasound-guided diagnostic or therapeutic procedures, such as PUBS. Fetal heart rate and activity is documented immediately following the procedure.
Amniocentesis is a safe and accurate procedure for sampling twin pregnancies between 15 and 20 weeks’ gestation. Amniocentesis in multiples was first described by Elias et al. in 1980 (Elias et al., 1980). Under ultrasound guidance, a spinal needle is introduced into one sac. After aspirating fluid, a blue dye (indigo carmine) is injected into that sac to serve as a marker before removing the initial needle. A second needle is then inserted into the other sac and the aspiration of clear fluid indicates that the second sac had been successfully entered (Figure 4-3). This technique is recommended when sampling either dichorionic or monochorionic twin pregnancies (but is not necessary in monoamniotic gestations). Likewise, the procedure can be used in triplets and higher order multiples. It is essential to map and document the location of each fetus and placenta at the time of amniocentesis. If discordant results are obtained and the patient desires selective termination, the appropriate fetus can then be targeted.
While use of a marker dye is extremely helpful in performing amniocentesis on patients with multiples, the instillation of some material has been associated with toxicity. For example, methylene blue has been linked to fetal hemolysis, multiple ileal obstructions, and jejunal atresia when injected into the amniotic fluid (Cowett et al., 1976; Nicolini and Monni 1990; Van der Pol et al., 1992). Indigo carmine has been evaluated and has not been shown to be associated with abnormal fetal outcomes (Cragan et al., 1993).
Alternative techniques have been described that do not use dye instillation to be sure that both sacs have been successfully sampled (Jeanty et al., 1990; Sebire et al., 1996). Sebire et al. described a technique utilizing single entry in 176 twin pregnancies (Sebire et al., 1996). After amniotic fluid is aspirated from the sac of one twin, the syringe is removed, the stylet replaced, and the needle is then advanced through the intertwin membrane into the sac of the second twin under continuous ultrasound guidance. When aspirating fluid from the second sac, the authors recommend discarding the first 1 mL in order to avoid possible contamination from amniotic fluid of the first sac. While this method is feasible, we continue to recommend the technique described earlier, using a separate needle insertion for each sac along with instillation of indigo carmine into the first in order to eliminate any chance of contaminating the samples.
Failure to culture amniocytes occurs in less than 1% of cases. Chromosomal mosaicism occurs in approximately 0.5% of cases. Chromosomal mosaicism is the presence of two or more cell lines with different karyotypes in a single person. This occurs as a result of postzygotic nondisjunction. The observation of multiple cell lines in a prenatal sample does not necessarily mean that the fetus has mosaicism. The most common type of mosaicism detected by amniocentesis is pseudomosaicism (Hsu and Perlis, 1984). This phenomenon should be suspected when an abnormality is evident in only one of several cultures of an amniotic fluid specimen. The abnormal cell lines arise during in vitro division; therefore, they are not present in the fetus and are not clinically important. Contamination by maternal cells can be minimized by discarding the first few drops of aspirated amniotic fluid. True fetal mosaicism, diagnosed when the same abnormality is present on more than one cover slip, is rare (0.25%) but clinically important (Hsu and Perlis, 1984). The question of whether true mosaicism is present is best resolved by karyotyping fetal lymphocytes obtained by PUBS (Gosden et al., 1988). PUBS can provide results within 48 hours. Detailed ultrasound examination is also recommended to assess fetal growth and exclude the diagnosis of structural anomalies. If both ultrasound and fetal blood sampling results are normal, the parents can be reassured that the major chromosomal abnormalities have been excluded (Gosden et al., 1988).
Serious maternal complications, such as maternal hemorrhage and sepsis, are virtually unheard of with amniocentesis in contemporary practice. Amnionitis occurs in 0.1% of cases (Turnbull and Mackenzie, 1983). The development of rhesus isoimmunization (Golbus et al., 1979; Hill et al., 1980) can be avoided by prophylactic administration of anti-D immunoglobulin to Rho(D)-negative women who are about to undergo amniocentesis. Amniotic fluid leak or vaginal blood loss, noted after amniocentesis by 2% to 3% of patients, is usually self-limiting, although occasionally leakage of amniotic fluid persists throughout pregnancy (NICHD Amniocentesis Registry, 1976; Simpson et al., 1981). Other common postprocedure symptoms include abdominal/pelvic cramping for 1 to 2 hours and lower abdominal discomfort for up to 48 hours after the procedure.
The safety and accuracy of midtrimester amniocentesis was documented in the mid-1970s by three collaborative studies performed in the United Kingdom, United States, and Canada (NICHD Amniocentesis Registry, 1976; Medical Research Council, 1977; Working Party on Amniocentesis, 1978). However, the only prospective, randomized, controlled trial is a study by Tabor et al. (1986), who reported on 4606 low-risk, healthy women. Patients, aged 25 to 34 years old, were randomly allocated to a group that would have amniocentesis or to one that would have ultrasound examination. The total rate of fetal loss in the patients who underwent amniocentesis was 1.7% and in the control patients 0.7% (P < 0.01). The conclusions of this study were initially criticized because an 18-gauge needle (which is associated with higher risks than smaller needles) was documented as having been used. Tabor et al. (1988) subsequently reported that they had been mistaken in citing the use of an 18-gauge needle and had, in fact, used a 20-gauge needle for most of the procedures. The Danish study also demonstrated that there were significant associations between pregnancy loss and puncture of the placenta, high MSAFP levels, and discolored amniotic fluid (Tabor et al., 1986).
Both the UK and Danish studies (Working Party on Amniocentesis, 1978; Tabor et al., 1986) found an increase in respiratory distress syndrome and pneumonia in neonates from the amniocentesis groups. Other studies have not found this association. The UK study showed an increased incidence of talipes and dislocation of the hip in the amniocentesis group (Working Party on Amniocentesis, 1978).
Much debate has centered on the current loss rates for amniocentesis, as anecdotal reports suggested that a 1% procedure-related loss rate appeared higher than what was seen in clinical practice. It was felt by many that the loss rate of 1% reflected clinical practice in the 1980s when ultrasound equipment and skill was significantly less advanced than contemporary practice, and when needle sizes were also different from contemporary practice. However, another randomized trial of amniocentesis will never occur due to ethical concerns. The only other option to provide contemporary data on amniocentesis safety is from large-scale prospective observational trials from the general population, and in which detailed pregnancy outcome data are available. The FASTER Trial (see Chapter 2) provided an ideal opportunity to obtain such updated safety data on amniocentesis (Malone et al., 2005). This study demonstrated a much lower procedure-related loss rate of 1 in 1600 (0.06%) (Eddleman et al., 2006). While the publication of this study has provoked much debate about the true procedure-related loss rate from amniocentesis, it is now commonly accepted that the loss rate is somewhere between the initial 1% quoted by Tabor (Tabor et al., 1986) and 0.06%, perhaps closer to 1 in 1000. The most recent ACOG statement on this topic describes the loss rate as being “less than 1 in 300–500” (ACOG, 2007).
Needle puncture of the fetus, reported in 0.1% to 3.0% of cases (NICHD Amniocentesis Registry, 1976; Karp and Hayden, 1977), has been suggested as the cause of exsanguination (Young et al., 1977), intestinal atresia (Swift et al., 1979; Therkelsen and Rehder, 1981), ileocutaneous fistula (Rickwood, 1977), gangrene of a fetal limb (Lamb, 1975), uniocular blindness (Merin and Beyth, 1980), porencephalic cysts (Youroukos et al., 1980), patellar-tendon disruption (Epley et al., 1979), skin dimples (Broome et al., 1976), and peripheral-nerve damage (Karp and Hayden, 1977). Continuous use of ultrasound to guide the needle minimizes needle puncture of the fetus. Finegan et al. (1990) have reported an increased incidence of middle ear abnormalities in children whose mothers underwent amniocentesis.
Early studies suggested a higher fetal loss rate in twin pregnancies than in those with singletons (Pijpers et al., 1988; Anderson et al., 1991). These studies, however, did not address whether the fetal wastage following amniocentesis is attributable to the procedure or to the twin gestation itself. Patients with multiples are at increased risk for fetal loss. In a case–controlled study, Ghidini et al. (1993) reported similar loss rates between sampled twins and unsampled matched twin controls (3% vs. 2.8%). They concluded that second trimester amniocentesis in twins was not associated with excess pregnancy loss and that the likelihood of fetal wastage secondary to this procedure is probably the same as that in singletons. More recent studies, however, have contradicted this finding. In a retrospective study of 476 twins who underwent amniocentesis and 477 who did not, Yukobowich et al. (2001) found statistically different loss rates 4 weeks after the procedure, 2.7% versus 0.6%. Toth-Pal et al. (2004) compared pregnancy loss in 175 twin pregnancies that underwent amniocentesis to 300 controls. The loss rate between 18 and 24 weeks’ gestation was 3.87% in the amniocentesis group versus 2.39% in the control group. Although these results were not statistically significant, the authors concluded that genetic amniocentesis slightly increases the loss rate in women carrying twins.
As the technique of midtrimester amniocentesis gained rapid acceptance, interest developed in the use of the same diagnostic technique at earlier gestational ages. The term “early amniocentesis” was used to describe the performance of amniocentesis at less than 14 weeks’ gestation. An assumption was made that since amniocentesis was shown to be a safe and effective procedure in the second trimester of pregnancy, it would be equally safe and effective during the first trimester. However, with the completion of the CEMAT study (Canadian Early and Midtrimester Amniocentesis Trial) in 1998, it has become clear that EA from 11 weeks to 12 weeks 6 days is associated with significant disadvantages (CEMAT Group, 1998).
In the CEMAT study, 4374 patients were randomized to either EA (defined as amniocentesis from 11 weeks to 12 weeks 6 days) or midtrimester amniocentesis (defined as amniocentesis from 15 weeks to 16 weeks 6 days). It was found that EA was significantly more likely to be a technically difficult or unsuccessful procedure when compared with midtrimester amniocentesis (1.6% vs. 0.4%), and it was twice as likely that EA would require more than one needle insertion (Johnson et al., 1999). In addition, it was found that EA was significantly more likely to result in karyotype culture failure (2.4%) when compared with midtrimester amniocentesis (0.25%) (Winsor et al., 1999).
The CEMAT investigators also demonstrated significant safety concerns with the EA technique. There was a significantly higher rate of total pregnancy loss in the EA group (7.6%) versus the midtrimester group (5.9%) (CEMAT Group, 1998). Also of concern was a significantly higher rate of clubfoot in the EA group (1.3%) versus the midtrimester group (0.1%). EA was also associated with a significantly higher chance of postprocedural amniotic fluid leakage (3.5%) when compared with midtrimester amniocentesis (1.7%). The CEMAT study effectively ended the controversy on the role of EA, and suggested that this technique should generally be avoided before the 13th week of gestation. The safety of amniocentesis between 13 and 14 weeks is unknown. Consequently, it is best to delay amniocentesis until 15 weeks’ gestation.
Indications for amniocentesis have changed considerably in recent years and are likely to evolve even further. The most common indication for amniocentesis is to obtain a fetal karyotype in pregnant women with an increased risk for fetal aneuploidy. The use of amniocentesis in the investigation of elevated MSAFP is declining. The use of amniocentesis to test for fetal lung maturity has decreased because ultrasound dating of pregnancy is now obtained in many cases, and premature deliveries are performed only if there are maternal or fetal indications. For the assessment of fetal anemia in severe red-cell isoimmunized pregnancies in the second trimester, amniocentesis has been replaced by Doppler ultrasound of the middle cerebral artery. Contemporary loss rates secondary to amniocentesis are quite low, likely approaching 1 in 1000.
With conventional amniocentesis, karyotype results may be received as late as the middle second trimester. This is a drawback because of the medical risks of performing pregnancy termination using dilation-and-evacuation procedures late in the pregnancy. In addition, delaying such procedures until after fetal movement is perceived may be emotionally difficult for the patient. Using chorionic villi as a source for fetal karyotyping during the 10th week of pregnancy was introduced experimentally by Hahnemann and Mohr in 1969, and the use of an endoscopic transvaginal approach (Figure 4-4) was evaluated by Hahnemann in 1974. Clinical use of chorionic villi for fetal sex determination was described in 1975 by a group from China. They performed blind transvaginal aspiration without ultrasound examination (Tietung Hospital, 1975). Once an ultrasound-guided technique for aspiration was introduced, CVS became accepted for more widespread use.
Ultrasound examination immediately before the procedure confirms fetal heart activity, appropriate growth, and location of the placenta (Figures 4-4 and 4-5). The position of the uterus and cervix is determined, and the anticipated catheter path is mentally mapped. If the uterus is severely anteverted, filling of the bladder frequently straightens its position. If a uterine contraction occurs it could interfere with passage of the catheter, and a decision may be made to delay the procedure until the contraction dissipates. When the uterine condition and location are favorable, the patient is placed in the lithotomy position; and the vulva, vagina, and cervix are aseptically prepared. A speculum is inserted, and the anterior lip of the cervix may be grasped with a tenaculum to aid in manipulating the uterus. The distal 3 to 5 cm of the catheter is molded into a slightly curved shape and then gently passed through the cervix until a loss of resistance is felt at the endocervix. The operator then waits until the ultrasonographer visualizes the tip of the catheter.
The catheter is inserted parallel to the placenta and passed almost to its distal end (Figures 4-4 and 4-5). The stylet is then removed and a 20-mL heparinized syringe containing nutrient medium is attached. The syringe is used to apply negative pressure. The catheter and attached syringe are then pulled back slowly, and the syringe is visually inspected for villi, which are easily seen with the naked eye as white branching structures. Once an adequate sample is obtained, the patient is discharged. The patient is instructed to inform her physician if she develops heavy bleeding, fever, or unusual vaginal discharge. A follow-up scan and MSAFP assay are performed at 16 weeks of gestation.
Alternative approaches to transcervical CVS include use of a reusable biopsy forceps, rather than a disposable plastic catheter. There are no comparative data available guiding which transcervical instrument is optimal.
Continuous ultrasound imaging is used to direct a 19-or 20-gauge spinal needle into the long axis of the placenta (Figures 4-6 and 4-7). After removal of the stylet, villi are aspirated into a 20-mL syringe containing tissue culture medium. It may be a reasonable alternative to amniocentesis or PUBS if karyotype evaluation is needed later in pregnancy when special circumstances may exist, such as severe oligohydramnios.
CVS is considered a safe alternative to amniocentesis in multiple gestations (Brambati et al., 1991b; Pergament et al., 1992; Wapner et al., 1993). Chorionic villi for chromosome or DNA analysis can be obtained either by transcervical catheter or by transabdominal needle approaches under ultrasound guidance. The advantage of CVS over amniocentesis is the earlier availability of results and, if discordant results are obtained, selective termination can be performed early in gestation when it is associated with a lower risk of adverse outcomes. First trimester diagnosis by CVS is particularly important for women with multiple gestations who may be considering multifetal pregnancy reduction. These patients can verify that specific fetuses are euploid prior to multifetal pregnancy reduction. Similar to amniocentesis, the location of each fetus and placenta should be carefully mapped and documented at the time that CVS is performed (Appelman and Furman, 2005).
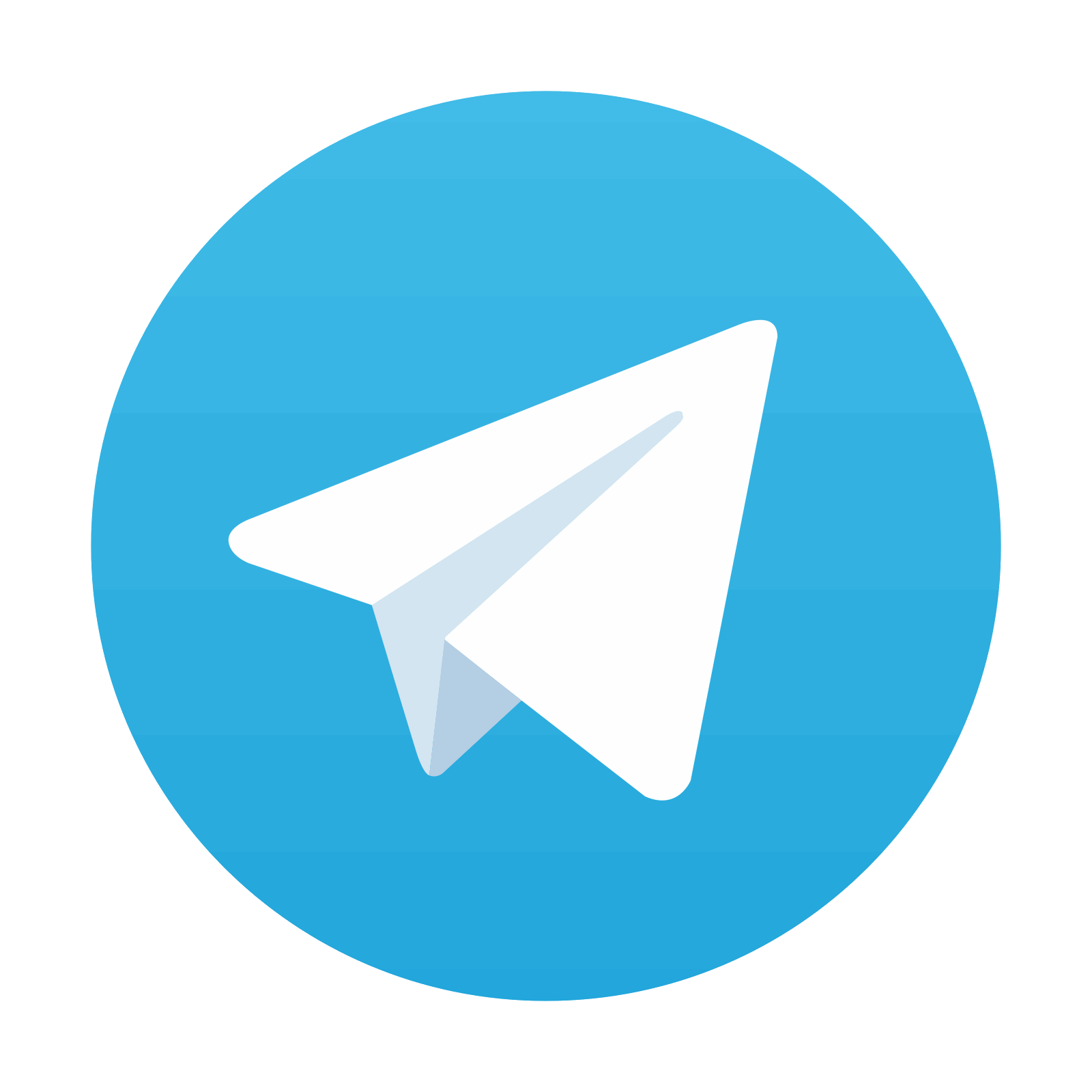
Stay updated, free articles. Join our Telegram channel

Full access? Get Clinical Tree
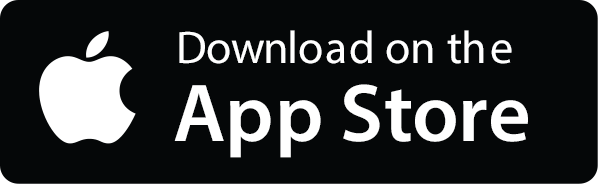
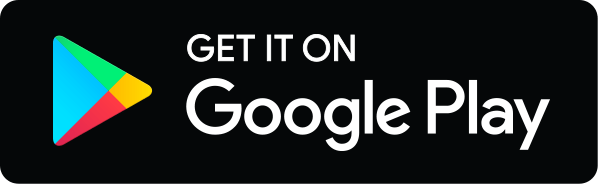