Key Terms
FGR: fetal growth restriction.
Rhizomelia: preferential shortening of the proximal segment of the limb.
Mesomelia: preferential shortening of the intermediate segment of the limb.
Micromelia: severe shortening of all segments of the limb.
Acromelia: severe shortening of the hands and/or feet.
Acromesomelic shortening: preferential shortening of the intermediate segment of the limb and hands or feet.
Amelia: absence of one or more limbs.
Hemimelia: absence or significant hypoplasia of the lower part of one or more limbs.
Phocomelia: absence of the proximal limbs with preserved distal elements.
Peromelia: malformation of one or more limbs.
Longitudinal limb deficiency: deficiency along the long axis of a limb (e.g. complete absence of the radius).
Transverse limb deficiency: deficiency along the transverse axis of a limb (e.g. congenital amputation associated with amniotic bands).
Butterfly vertebra: sagittal cleft in the vertebral body caused failure of fusion of the lateral haves due to persistent notochordal tissue between them.
Hemivertebra: abnormal vertebral development resulting in absence of one half of a vertebral body. The abnormal vertebra acts as a wedge in the spine, resulting in curvature away from where it is present.
Block vertebra: lack of proper segmentation leading to fusion of adjacent vertebral bodies.
Craniosynostosis: premature fusion of cranial sutures.
Skeletal dysplasias: group of complex and heterogeneous disorders that affect bone development and growth, resulting in alterations of bone size, shape, density and/or integrity.
Fetal skeletal abnormalities are usually first suspected during a routine obstetrical ultrasound examination. In this chapter, we will briefly review the development of the fetal skeleton, propose a diagnostic approach to evaluate fetuses with skeletal anomalies, review the most common isolated skeletal anomalies encountered in fetal imaging practice, and discuss imaging features and differential diagnosis for the most frequent skeletal dysplasias that can be diagnosed prenatally.
Humans are born with 270 bones. After fusion of several bones, 206 can be distinguished in adult life.1 The skeleton provides support and structure for the body, protects vital internal organs, such as the brain, heart, and lungs, and allows locomotion. It is composed of two distinct tissues (cartilage and bone), and three cell types (chondrocytes in cartilage and osteoblasts and osteoclasts in bone).2 Interaction of osteoblasts, osteoclasts, and chondrocytes promote skeletal development, growth, and remodeling. Multiple genes participate in the molecular control of chondrogenesis and osteogenesis, including fibroblast growth factors (FGFs), Sonic hedgehog (SHH), cartilage-derived morphogenetic protein (CDMP), Indian hedgehog (IHH), mammalian SRY box 9 (SOX-9), procollagen type 2 alpha 1(COL2A1), homeotic genes (HOX), Notch and Wnt signaling pathways, among many others. A detailed description of genetic control of bone formation is beyond the scope of this chapter, and the interested reader is referred to the excellent review by Karsenty et al.2
The axial and appendicular skeletons are formed by endochondral ossification, a process whereby cartilaginous models (anlagen) of future bones differentiate within mesenchymal condensations, followed by the development of primary ossification centers and bone marrow. Secondary epiphyseal ossification centers develop later and can be detected by ultrasound in several bones, including the distal femur in 17% of fetuses by 30 weeks and 100% of fetuses by 37 weeks, the distal tibia in 17% of fetuses by 34 weeks and 100% of fetuses by 40 weeks, and the proximal humerus in 28% of fetuses by 38 weeks and 55% of fetuses by 40 weeks (Figure 23-1).3,4 The portion of cartilage trapped between primary and secondary ossification centers is known as physis. The physis is responsible for longitudinal growth until the epiphyses and diaphyses fuse at the end of puberty. The flat bones of the skull, face, mandible and clavicles are formed by membranous ossification, with mesenchymal cells differentiating directly into osteoblasts.5 Remodeling is a perennial homeostatic process aimed at maintaining bone mass, shape, and integrity, orchestrated by osteoblasts and osteoclasts through alternate bone deposition and resorption, respectively.6
Only a minority of fetuses diagnosed with a skeletal anomaly have a risk factor for such (eg, a family history for skeletal dysplasia). Indeed, most skeletal abnormalities are first suspected during a routine ultrasonographic examination, either because of abnormal biometric measurements or due to deviations from normal in bone mineralization, shape, and/or integrity.
Long bone shortening is a frequent feature of skeletal dysplasias. Indeed, when fetuses are investigated for possible skeletal dysplasias, all long bones, calvarium, clavicles, and scapulae should be carefully imaged and measured. However, one bone in particular, the femur, is routinely measured during routine obstetrical ultrasound examinations. Therefore, a short femur length is usually the first clue that a skeletal dysplasia may be present.
A short femur is defined by most authors as a femur length less than 5th percentile for gestational age.7,8 Accurate assessment requires a well-dated pregnancy. Only the calcified diaphysis is measured (Figure 23-2). The differential diagnosis for a short femur includes inaccurate dating, constitutional causes (eg, ethnicity and familial short stature), fetal growth restriction (FGR), chromosomal anomalies, and skeletal dysplasias.7
Three studies have looked into the relative frequency of the various etiologies for a short femur (Table 23-1).7-9 Analysis of the data provided by these studies shows that a detailed ultrasonographic examination uncovers additional structural anomalies in approximately 34% of the cases (range 22%-47%). These anomalies fall into four main categories: (1) skeletal dysplasias (24%-35%), (2) chromosomal anomalies (24%-40%), (3) multiple anomalies with no unifying diagnosis (28%-48%), and (4) single gene disorders (4%-9%). Femur length shortening is isolated in approximately 66% of the cases (range 53%-78%). The majority (38%-61%) have no identifiable cause. In an additional 13% to 20%, a false positive measurement is uncovered during a follow-up exam. For the remaining 39% to 43%, FGR is diagnosed as the cause of femur length shortening. An important observation made by Papageorghiou et al7 is that 90% of the fetuses with a femur length less than 5th percentile and true FGR have abnormal uterine artery Doppler velocimetry, defined in their study as a mean RI greater than 0.55 in the presence of bilateral diastolic notches or a mean RI greater than 0.65 regardless of the presence of diastolic notches. Using less stringent criteria to diagnose a short femur, Todros et al9 found that 50% of SGA fetuses with a femur length less than 10th percentile for gestational age have abnormal uterine and umbilical artery Doppler velocimetry.
Structurally Abnormal | Structurally Normal | ||||||||||
---|---|---|---|---|---|---|---|---|---|---|---|
Author/Year | Criteria | N | Subtotal N (%) | Skeletal Dysplasias | Aneuploidy | Genetic | Multiple Anomalies with no Unifying Diagnosis | Subtotal N (%) | SGA | Unexplained | False + |
Todros et al9/2004 | <10th percentile | 86 | 40 (47%) | 33% | 40% | – | 28% | 46 (53%) | 39% | 61% | – |
Papageorghiou et al7/2008 | <5th percentile | 129 | 46 (36%) | 35% | 22% | 9% | 35% | 83 (64%) | 40% | 47% | 13% |
Vermeer & Bekker8/2003 | <5th percentile | 112 | 25 (22%) | 24% | 24% | 4% | 48% | 87 (78%) | 43% | 38% | 20% |
Other observational studies have assessed disease associations for fetuses with isolated short femur, ie, after exclusion of those with additional anomalies and skeletal dysplasias. One of these was a large population-based study conducted in Denmark,10 a country where all pregnant women are offered first trimester risk assessment for trisomy 21 and a second-trimester scan free of charge as part of routine antenatal care. The prevalence of isolated short femur was 1.8% (2718/147,766). After excluding cases with a femur length more than 6 standard deviations below the mean for gestational age, FGR was diagnosed in 13.9% of the fetuses, 5.6% of whom delivered before 34 weeks (OR = 4.2, 95% CI 3.5-4.9). The stillbirth rate in this population was 1.8% (OR = 6.0, 95% CI 4.4-8.1). Asian ethnicity and maternal tobacco use were common among fetuses with an isolated short femur. Despite first trimester risk assessment for trisomy 21, a short femur length (with or without additional ultrasonographic markers) was present in 11 of 68 fetuses with trisomy 21 (16.2%), 3 of 25 cases of trisomies 13 or 18 (12%), 1 of 5 cases of Turner syndrome (20%), and 8 of 25 cases of unbalanced autosomal structural abnormalities (32%). Several other studies have confirmed the association between isolated short femur and FGR, with frequencies ranging between 13.8% and 39.1%.11-18
The diagnostic approach to a fetus at risk for skeletal dysplasia requires detailed measurement of all long bones. Once this is accomplished, measurements should be compared to nomograms (Tables 23-2, 23-3, 23-4) and the pattern of bone shortening classified according to the involved segments (Figure 23-3).
Humerus | Ulna | Radius | |||||||
---|---|---|---|---|---|---|---|---|---|
Percentile | Percentile | Percentile | |||||||
Week | 5th | 50th | 95th | 5th | 50th | 95th | 5th | 50th | 95th |
12 | — | 9 | — | — | 7 | — | — | 7 | — |
13 | 6 | 11 | 16 | 5 | 10 | 15 | 6 | 10 | 14 |
14 | 9 | 14 | 19 | 8 | 13 | 18 | 8 | 13 | 17 |
15 | 12 | 17 | 22 | 11 | 16 | 21 | 11 | 15 | 20 |
16 | 15 | 20 | 25 | 13 | 18 | 23 | 13 | 18 | 22 |
17 | 18 | 22 | 27 | 16 | 21 | 26 | 14 | 20 | 26 |
18 | 20 | 25 | 30 | 19 | 24 | 29 | 15 | 22 | 29 |
19 | 23 | 28 | 33 | 21 | 26 | 31 | 20 | 24 | 29 |
20 | 25 | 30 | 35 | 24 | 29 | 34 | 22 | 27 | 32 |
21 | 28 | 33 | 38 | 26 | 31 | 36 | 24 | 29 | 33 |
22 | 30 | 35 | 40 | 28 | 33 | 38 | 27 | 31 | 34 |
23 | 33 | 38 | 42 | 31 | 36 | 41 | 26 | 32 | 39 |
24 | 35 | 40 | 45 | 33 | 38 | 43 | 26 | 34 | 42 |
25 | 37 | 42 | 47 | 35 | 40 | 45 | 31 | 36 | 41 |
26 | 39 | 44 | 49 | 37 | 42 | 47 | 32 | 37 | 43 |
27 | 41 | 46 | 51 | 39 | 44 | 49 | 33 | 39 | 45 |
28 | 43 | 48 | 53 | 41 | 46 | 51 | 33 | 40 | 48 |
29 | 45 | 50 | 55 | 43 | 48 | 53 | 36 | 42 | 47 |
30 | 47 | 51 | 56 | 44 | 49 | 54 | 36 | 43 | 49 |
31 | 48 | 53 | 58 | 46 | 51 | 56 | 38 | 44 | 50 |
32 | 50 | 55 | 60 | 48 | 53 | 58 | 37 | 45 | 53 |
33 | 51 | 56 | 61 | 49 | 54 | 59 | 41 | 46 | 51 |
34 | 53 | 58 | 63 | 51 | 56 | 61 | 40 | 47 | 53 |
35 | 54 | 59 | 64 | 52 | 57 | 62 | 41 | 48 | 54 |
36 | 56 | 61 | 65 | 53 | 58 | 63 | 39 | 48 | 57 |
37 | 57 | 62 | 67 | 55 | 60 | 65 | 45 | 49 | 53 |
38 | 59 | 63 | 68 | 56 | 61 | 66 | 45 | 49 | 54 |
39 | 60 | 65 | 70 | 57 | 62 | 67 | 45 | 50 | 54 |
40 | 61 | 66 | 71 | 58 | 63 | 68 | 46 | 50 | 55 |
Tibia | Fibula | Femur | |||||||
---|---|---|---|---|---|---|---|---|---|
Percentile | Percentile | Percentile | |||||||
Week | 5th | 50th | 95th | 5th | 50th | 95th | 5th | 50th | 95th |
12 | — | 7 | — | — | 6 | — | 4 | 8 | 13 |
13 | — | 10 | — | — | 9 | — | 6 | 11 | 16 |
14 | 7 | 12 | 17 | 6 | 12 | 19 | 9 | 14 | 18 |
15 | 9 | 15 | 20 | 9 | 15 | 21 | 12 | 17 | 21 |
16 | 12 | 17 | 22 | 13 | 18 | 23 | 15 | 20 | 24 |
17 | 15 | 20 | 25 | 13 | 21 | 28 | 18 | 23 | 27 |
18 | 17 | 22 | 27 | 15 | 23 | 31 | 21 | 25 | 30 |
19 | 20 | 25 | 30 | 19 | 26 | 33 | 24 | 28 | 33 |
20 | 22 | 27 | 33 | 21 | 28 | 36 | 26 | 31 | 36 |
21 | 25 | 30 | 35 | 24 | 31 | 37 | 29 | 34 | 38 |
22 | 27 | 32 | 38 | 27 | 33 | 39 | 32 | 36 | 41 |
23 | 30 | 35 | 40 | 28 | 35 | 42 | 35 | 39 | 44 |
24 | 32 | 37 | 42 | 29 | 37 | 45 | 37 | 42 | 46 |
25 | 34 | 40 | 45 | 34 | 40 | 45 | 40 | 44 | 49 |
26 | 37 | 42 | 47 | 36 | 42 | 47 | 42 | 47 | 51 |
27 | 39 | 44 | 49 | 37 | 44 | 50 | 45 | 49 | 54 |
28 | 41 | 46 | 51 | 38 | 45 | 53 | 47 | 52 | 56 |
29 | 43 | 48 | 53 | 41 | 47 | 54 | 50 | 54 | 59 |
30 | 45 | 50 | 55 | 43 | 49 | 56 | 52 | 56 | 61 |
31 | 47 | 52 | 57 | 42 | 51 | 59 | 54 | 59 | 63 |
32 | 48 | 54 | 59 | 42 | 52 | 63 | 56 | 61 | 65 |
33 | 50 | 55 | 60 | 46 | 54 | 62 | 58 | 63 | 67 |
34 | 52 | 57 | 62 | 46 | 55 | 65 | 60 | 65 | 69 |
35 | 53 | 58 | 64 | 51 | 57 | 62 | 62 | 67 | 71 |
36 | 55 | 60 | 65 | 54 | 58 | 63 | 64 | 68 | 73 |
37 | 56 | 61 | 67 | 54 | 59 | 65 | 65 | 70 | 74 |
38 | 58 | 63 | 68 | 56 | 61 | 65 | 67 | 71 | 76 |
39 | 59 | 64 | 69 | 56 | 62 | 67 | 68 | 73 | 77 |
40 | 61 | 66 | 71 | 59 | 63 | 67 | 70 | 74 | 79 |
Gestational Age (wk) | Percentile | ||
---|---|---|---|
10th | 50th | 90th | |
14 | 1.6 | 1.8 | 2.1 |
15 | 1.6 | 1.9 | 2.2 |
16 | 1.8 | 2.2 | 2.8 |
17 | 1.9 | 2.2 | 2.2 |
18 | 1.9 | 2.7 | 3.0 |
19 | 2.5 | 3.0 | 3.9 |
20 | 3.3 | 3.3 | 3.3 |
21 | 2.4 | 2.4 | 2.4 |
22 | 2.5 | 3.6 | 4.0 |
23 | 4.1 | 4.1 | 4.0 |
24 | 4.6 | 4.6 | 4.6 |
25 | 4.0 | 4.7 | 5.3 |
26 | 4.0 | 4.7 | 5.4 |
27 | 4.5 | 5.0 | 5.6 |
28 | 5.1 | 5.3 | 5.5 |
29 | 4.9 | 5.4 | 5.8 |
30 | 6.1 | 6.1 | 6.1 |
31 | 5.1 | 5.6 | 5.2 |
32 | 5.4 | 5.7 | 6.2 |
33 | 5.9 | 5.9 | 5.9 |
34 | 6.0 | 6.5 | 7.1 |
35 | 7.1 | 7.1 | 7.1 |
Most nomograms available for evaluation of long bone shortening, including the ones on Tables 23-2 and 23-3, provide the mean, 5th and 95th percentiles for each biometric parameter. It is important to keep in mind that 5% of the general population will fall below the 5th percentile for gestational age. Ideally, a more stringent criterion, such as the 1st percentile of limb growth for gestational age should be used to diagnose skeletal dysplasias. Unfortunately, none of the currently available nomograms have been based on the number of patients required to provide an accurate discrimination between the 5th and 1st percentiles. A recently published international multicentric study reported the 3rd percentile for femur length based on longitudinal measurements of 4321 pregnancies from 8 countries.19 Since most skeletal dysplasias diagnosed in utero are characterized by remarkable bone shortening, the precise lower boundary may not be critical. Kurtz et al20 have shown that most fetuses with significant fetal skeletal dysplasias have a femur length more than 4 SD below the mean for gestational age (or 5 mm <2 SD). Specifically, in a study of 27 fetuses with femoral shortening, those with a femur length more than 4 SD below the mean had a skeletal dysplasia. Only one fetus with less severe femoral shortening turned out to have achondroplasia. It is well known that achondroplasia, hypochondroplasia, and mild forms of osteogenesis imperfecta may not manifest with significant bone shortening until the third trimester.21
Regarding specific patterns of long bone shortening, the term rhizomelia is used when the proximal segment of the limb is more severely affected than the intermediate and distal segments. Mesomelia implies more severe shortening of the intermediate segment (ulnae and radii, tibiae, and fibulae). In acromelia, the hands and feet are more severely affected. In micromelia, all segments of a given extremity are severely shortened. Comparisons between bone measurements of the tibia and femur, and ulna and humerus can be performed using the charts depicted in Figures 23-4 and 23-5, providing objective support to an initial gestalt assessment. Table 23-5 lists some of the more common skeletal dysplasias that can be diagnosed prenatally, grouped according to the preferentially affected segments.22
Rhizomelia |
Achondroplasia |
Atelosteogenesis |
Chondrodysplasia punctata (rhizomelic type) |
Congenital short femur |
Fibrochondrogenesis (severe micromelia in rhizomelic distribution) |
Larsen syndrome (rhizomelic upper limb shortening) |
Mesomelia |
Mesomelic dysplasia (Langer, Reinhardt, and Robinow types) |
Oro-facial-digital syndrome |
Acromelia |
Ellis–van Creveld syndrome (chondroectodermal dysplasia) |
Micromelia |
Achondrogenesis |
Astley-Kendall dysplasia |
Atelosteogenesis |
Blomstrand dysplasia |
Boomerang dysplasia |
Diastrophic dysplasia |
Dyssegmental dysplasia (microcampomelia) |
Fibrochondrogenesis (severe micromelia in rhizomelic distribution) |
Grebe dysplasia |
Greenberg dysplasia |
Hypophosphatasia, perinatal lethal form |
Kniest’s dysplasia |
Opsismodysplasia |
Omodysplasia, recessive type |
Osteogenesis imperfecta (type II) |
Platyspondylic dysplasia, Torrance type |
Short rib–polydactyly syndrome |
Robert’s syndrome |
Schneckenbecken dysplasia |
Thanatophoric dysplasia |
Evaluation of bone morphology encompasses assessment of mineralization, bowing and/or angulations, fractures, and ancillary findings such as bony spurs and epiphyseal stippling.
Mineralization can be assessed by evaluating bone echogenicity and acoustic shadowing. Figure 23-6A shows lack of visualization of the vertebral bodies of the spine due to severe bone demineralization in a fetus with achondrogenesis type 2. More frequently, however, demineralization is first suspected by observing unusually clear images of the brain hemisphere closest to the transducer on axial images of the fetal head (Figure 23-7A). In addition, a poorly mineralized calvarium may be soft and compressible upon application of transducer pressure (Figures 23-8A and B). Unusually clear images of the brain hemisphere closest to the transducer may also be seen on axial views in case of enlarged cranial sutures and fontanels, such as in cleidocranial dysplasia (Figures 23-9A to C).
Figure 23-6.
Achondrogenesis type 2. A: Poor mineralization of vertebral bodies of the spine (arrows). B: Extremely short femur. C: Extremely short humerus. D: Extremely short ribs. E: Cystic hygroma. F: Postmortem x-rays; note the severe micromelic shortening of all long bones, metaphyseal flaring, and lack of mineralization of the vertebral bodies of the thoracic and lumbar spine with only the pedicles well visualized. G: Postmortem photographs.







Figure 23-7.
Osteogenesis imperfecta type 2. A: Poorly mineralized calvarium. Note that the hemisphere closest to the transducer (arrow) is seen as well as the hemisphere away from the transducer (arrowhead). B: Multiple rib fractures. C: Severe micromelic shortening of the femur and humerus. D: Three-dimensional rendered views of the spine show normal ossification of the vertebral bodies of the spine (differentiating it from achondrogenesis type 1A, which is characterized by membranous calvarium, extremely short long bones—such as in this case, but unossified vertebral bodies with partly ossified pedicles).




Figure 23-8.
A: Axial view of the fetal head at the level of the biparietal diameter in a case of hypophosphatasia. The brain hemisphere close to the transducer is as well seen as the distal hemisphere, suggesting either poor mineralization of the skull or unusually large sutures and fontanels (see Figure 23-9). B: The parietal bone of the side of the head close to the transducer is easily compressible with gentle transducer pressure, indicating poor mineralization of the skull. C: Short humerus with elbow spur. D: Bowed radius and ulna due to fractures. E: Ribs appear thin with subtle fractures at the lateral ends of the inferior right ribs. F: AP postmortem radiograph confirms marked demineralization of the calvarium, bowed radii and ulnae with fractures, fracture of the distal left femur, and lack of ossification of the posterior neural arches of the distal thoracic and lumbosacral spine. G: The central metaphyseal lucency described for hypophosphatasia is seen to better advantage in this view along the proximal metaphyses of the right tibia.







Figure 23-9.
Cleidocranial dysplasia. A: Unusually well-visualized cerebral hemisphere close to the transducer, this time secondary to widened cranial sutures (3D reconstructions in B and C). D: Hypoplastic clavicles. E: Postnatal skull x-rays confirming widened sutures. F: AP view of the chest showing hypoplastic clavicles (arrowheads). G: AP view of the pelvis showing lack of ossification of the symphysis pubis.






Evaluation of bone shape may be difficult prenatally, since the epiphyses are not fully ossified in utero and metaphyseal shape is difficult to assess using a reflective technology such as two-dimensional ultrasound (2DUS). In this regard, three-dimensional ultrasonography (3DUS) or computerized tomography with 3D rendering (3DCT) may be advantageous compared to 2DUS. Moeglin and Benoit,23 for example, described a pointed appearance of the proximal femur metaphysis in a fetus with achondroplasia by 3DUS. Using CT with 3D reconstruction, Boulet et al24 subsequently described an abnormal connection between the proximal femoral epiphysis and diaphysis in fetuses with achondroplasia characterized by relative overgrowth of the periosteum, which they termed the “collar hoop” sign. This sign appears at the proximal diaphyseal end as a small hyperechogenic hook. In the same paper, the authors noted that the angle formed between the proximal metaphysis and diaphysis measured by 2DUS was abnormally wide in 5 of 5 fetuses with achondroplasia but normal in 653 healthy fetuses. The mean proximal metaphyseal-epiphyseal angle in normal fetuses is 98.5° ± 6.8° at 22 weeks and 105.6° ± 7.3° at 32 weeks of gestation (Figure 23-10A). This finding has been confirmed by another group in a study of 377 normal fetuses, 6 fetuses with achondroplasia, and 70 small-for-gestational age (SGA) fetuses.25 The proximal femoral metaphyseal-diaphyseal angle was significantly wider in fetuses with achondroplasia compared to control (1.36 MoM vs 1.00 MoM, p <0.001) and SGA fetuses (1.36 MoM vs 1.04 MoM, p <0.001), respectively. Five of the 6 fetuses with achondroplasia had a proximal metaphyseal-diaphyseal angle more than 130° (Figure 23-10B).
The skeletal anomalies described in the following section are the most frequently seen in clinical practice, either as isolated findings or as sentinel anomalies associated with multiple malformations, chromosomal anomalies, genetic syndromes, and skeletal dysplasias.
Clubfoot, also known as talipes equinovarus, is the most common skeletal anomaly diagnosed by prenatal ultrasonography. It occurs in 1 to 2 per 1000 pregnancies and is more frequent in males (2:1 ratio).26,27 Approximately 25% of the cases are familial, and 33% concordance has been reported among identical twins.28
On prenatal ultrasound, the normal alignment of the tibia and fibula does not allow simultaneous visualization of the long axis of the foot. In clubfoot, there is subluxation of the talo-calcaneo-navicular joint, adduction of the forefoot, heel inversion, and plantar flexion of the forefoot in relation to the ankle.29 Thus, clubfoot is recognized prenatally by simultaneous visualization of the long axis of the tibia/fibula and plantar aspect of the foot in the same plane (Figures 23-11A to C). Prenatal diagnosis of clubfoot should prompt a thorough anatomical survey, including detailed skeletal imaging and measurements. The term “complex” or “syndromic” clubfoot is used when the anomaly is associated with other structural anomalies, genetic conditions, neuromuscular disorders, and skeletal dysplasias. Distal arthrogryposis, myotonic dystrophy, myelomeningocele, trisomy 18, and amniotic band syndrome are among the most commonly associated conditions.30 Severe oligohydramnios, given the prolonged restriction of fetal movements, may also result in clubfoot. Mammen and Benson31 reported a 30% rate of aneuploidy in fetuses with complex clubfoot examined at a referral center. Isolated clubfoot is more common than complex clubfoot, with the risk of aneuploidy ranging between 3.6% and 5.9%.32,33 Some investigators, however, did not find a higher incidence of chromosomal anomalies in cases of isolated unilateral or bilateral clubfoot, within the context of a normal detailed ultrasonographic examination and no additional maternal indication for invasive prenatal testing.34 In cases of isolated clubfoot, particularly when unilateral, it is important to confirm the diagnosis on subsequent exams, as false-positive diagnoses have been reported in 10% to 19% of cases.33,35 The prognosis for complex clubfoot is dependent on the severity of associated anomalies. The prognosis for isolated clubfoot depends on whether the deformity is positional or rigid, a distinction that may be difficult to establish prenatally. After delivery, positional clubfoot is not rigid by physical examination and may not require clinical or surgical treatment. Rigid clubfoot is usually treated by casting and percutaneous Achilles tendon tenotomy if necessary. Further surgical correction may be required for selected indications such as lack of response to casting, incomplete correction, overcorrection, and residual muscle imbalance.27,30,36
Polydactyly is the most common hereditary limb malformation.37 The anomaly is characterized by an additional digit that may range from a fleshy nubbin to a complete digit with controlled flexion and extension. Polydactyly may be preaxial, central, and postaxial.38 Postaxial polydactyly is the most common type (Figure 23-12), occurs in the ulnar side of the hand or fibular side of the foot, and is 10 times more common in blacks. Preaxial polydactyly occurs in the radial side of the hand or the tibial side of the foot, is more common among whites, and is more frequently associated with syndromes, particularly when a duplicated triphalangeal thumb is present. Central polydactyly is characterized by an extra digit between the long and ring fingers, is more commonly bilateral, and may be associated with other malformations of the hands and feet.38,39
Most cases of polydactyly are isolated and autosomal dominant. However, polydactyly is a known phenotypic trait of 119 disorders, 97 of which are syndromic.40 Mutations in the downstream effector of SHH GLI3, for example, give rise to several types of polydactyly, which are classified from types I (less severe) to IV (more severe). Greig cephalopolysyndactyly consists of pre- or postaxial polysyndactyly associated with frontal bossing, hypertelorism, and occasionally agenesis of the corpus callosum, hydrocephalus, umbilical, and diaphragmatic hernia.41 A more severe disorder associated with GLI3 frameshift mutations is the autosomal dominant Pallister-Hall syndrome, characterized by hypothalamic hamartomas, craniofacial anomalies, polysyndactyly, and anal defects.42
Syndactyly is defined as fused or narrowed web space between adjacent fingers (Figure 23-13). The clinical spectrum is heterogeneous, with unilateral, bilateral, symmetric, and asymmetric presentations possible. The incidence is approximately 2 to 3 per 10,000 live births. Autosomal-dominant inheritance is often present, particularly in cases of isolated syndactyly between the second and third toes.43 According to the length of the web between the fingers, syndactyly can be classified as incomplete or complete. Bony fusion occurs in complex syndactyly whereas only the skin is fused in simple syndactyly.38 Frequently seen in isolation, syndactyly may also occur as a component of more than 300 syndromes.44 Some of the best known are triploidy, Apert, and Saethre-Chotzen syndromes.44 Apert syndrome, caused by heterozygous mutation in the FGFR2 gene, is characterized by craniosynostosis, midface hypoplasia, and polysyndactyly of the hands and feet.45 Saethre-Chotzen syndrome is caused by mutations in the TWIST1 gene. The phenotype consists of coronal synostosis (Figure 23-14), ptosis, small ear pinna with a prominent crus, and variable presence of syndactyly of digits 2 and 3. Other manifestations include parietal foramina, vertebral fusions, radioulnar synostosis, cleft palate, maxillary hypoplasia, hypertelorism, duplicated distal hallucal phalanx, and congenital heart disease.46 Acrosyndactyly, defined as digital fusion at the fingertips along with a variably patent proximal web is often seen in cases of amniotic band sequence.38
Figure 23-14.
Cranial abnormalities on 3DUS in a 19-week fetus. Rendered reformatted image of the coronal suture shows a bony bridge between the frontal and parietal bones, consistent with coronal craniosynostosis (arrow) in a case of Saethre–Chotzen syndrome. (Reproduced with permission from Gonçalves LF. Three-dimensional ultrasound of the fetus: how does it help? Pediatr Radiol. 2016 Feb;46(2):177-189.)

Limb deficiency disorders occur in 1 per 1300 to 1 per 2000 births.47 There are two basic types of limb deficiencies: longitudinal and transverse. The specific type depends on whether the deficiency occurs along the long or the short axis of the limb. Thus, complete absence of the radius, for example, is classified as a longitudinal limb deficiency, whereas congenital amputation of the distal forearm caused by amniotic bands is classified as a transverse limb deficiency. Longitudinal deficiencies can be further divided into preaxial (eg, radius or tibia affected), postaxial (eg, ulna or fibula affected), central (eg, split hand or foot deformity, whereby the central digits are affected), or a combination of pre- and postaxial limb deficiencies affecting the same limb. The following terms are commonly used in the literature to describe limb deficiencies48:
Amelia: absence of one or more limbs
Hemimelia: absence or significant hypoplasia of the lower part of one or more limbs
Phocomelia: absence of the proximal limbs with preserved distal elements
Peromelia: malformation of one or more limbs
Oligodactyly: fewer than 5 fingers or toes
Split-hand and split-foot: used interchangeably with ectrodactyly and “lobster claw” deformity to describe absence of central digits producing a cleft-like appearance of the hands or feet
The limb deficiencies comprise a very heterogeneous group of disorders. Online Inheritance in Man (OMIM) describes over 120 limb deficiency disorders, with the molecular basis currently known for approximately 40.49 These anomalies can occur in isolation or in association with other anomalies. A relatively straightforward and comprehensive way to describe congenital limb deficiencies has been proposed by Gold et al.50 The method consists in first describing the deficiency as longitudinal or transverse, followed by a well-structured description of the affected segments (Figure 23-15).
The incidence of limb deficiencies from a Hungarian registry is presented in Table 23-6. In a large European study including 709,030 births from 20 malformation registries in 12 countries, the overall prenatal detection rate for congenital limb deficiencies was 35.6% (89/250), with a higher detection rate observed among cases with associated malformations (49.1% [55/112]).51 The frequency of chromosomal anomalies in the presence of associated malformations was 14.3% (16/112), with 8 cases of trisomy 18, 2 cases of trisomy 21, and 1 case each of triple X, Klinefelter syndrome, deletion 7q, + isochromosome 12p, deletion 3q, and unbalanced translocation t(5;14)(p13;q13). Among fetuses with a normal karyotype and associated anomalies, 33.9% (38/112) had syndromic disorders or associations, including amniotic band sequence, VATER/VACTERL association, Poland syndrome, hypoglossia-hypopolydactyly, Cornelia De Lange syndrome, thrombocytopenia–radial aplasia (TAR) syndrome, limb body wall complex, caudal regression syndrome, Fanconi anemia, ulnar mammary syndrome Holt-Oram syndrome, Roberts syndrome, femur-fibula-ulna syndrome, Aarskog syndrome, Adams-Oliver syndrome, otopalatodigital syndrome type II, Robinow syndrome, myopathy-congenital nonprogressive with Moebius sequence, Robin sequence, Carey-Fineman-Ziter syndrome, and twin-reversed arterial perfusion sequence.
Transverse deficiencies, or congenital amputations, are more common than longitudinal deficiencies. A substantial proportion of these are caused by amniotic band disruption sequence. Other risk factors include chorionic villus sampling performed before 10 weeks of gestational and failed abortion attempts using misoprostol.47,50,52,53 In most cases, transverse limb deficiencies are sporadic. Sonographic findings have been reviewed in depth by Bromley and Benacerraf.54 Whenever a transverse limb deficiency is seen, an attempt should be made to identify amniotic bands by ultrasound. Even if a band is not identified, the finding of additional disruptive anomalies during the examination (eg, asymmetric cranial and facial clefts or bizarre body wall defects) should lead to a high degree of suspicion for amniotic band sequence (Figure 23-16). In case of amniotic bands, keep in mind the possibility of Adams-Oliver syndrome, an extremely rare but related disorder characterized by terminal transverse limb defects (including amputations), syndactyly, brachydactyly, and oligodactyly. This mimicker of amniotic band syndrome is associated with aplasia cutis congenita of the scalp vertex, which is unlikely to be detected by prenatal ultrasound. Congenital heart disease is seen in Adams-Oliver syndrome in 20% of the cases.55 Becker et al56 reported prenatal ultrasonographic diagnosis of two cases of Adams-Oliver syndrome in the same family, the first at 13 weeks and the second at 23 weeks of gestation. Both showed limb reduction defects and, in the second case, a scalp defect was diagnosed as an echo-free space between the scalp and bone.
Longitudinal deficiencies are less common than transverse deficiencies; however, they are often part of syndromic or chromosomal anomalies. The most common longitudinal limb deficiencies with their respective syndromic associations are discussed in the following section.
Proximal femoral focal deficiency (PFFD) may be unilateral or bilateral. When unilateral, the right femur is more frequently affected (Figure 23-17). Anomalies of the upper limbs may be present and do not exclude the diagnosis.57,58 PFFD is classified according to Aitken into four classes (A-D) based on radiographic characteristics of the acetabulum, femoral head, femoral shaft, and femoral shaft attachment at maturity.59 The distinction between the different subtypes is not possible by prenatal ultrasound as postnatal MRI is required to evaluate the cartilaginous structures of the proximal femur and acetabulum, as well as associated musculature. The disease is rare (1/52,000 births) and is thought to be sporadic, particularly when isolated.60 Except in bilateral proximal focal deficiency, which occurs in 15% of the cases, the diagnosis is usually suspected when unilateral shortening of the femur is noted in the absence of other anomalies (Figures 23-18 and 23-19).57,61,61a Associated osseous abnormalities include ipsilateral fibular hemimelia, hypoplastic patellae, agenesis of the cruciate ligaments and/or menisci, tibial shortening, tarsal coalition, equinovalgus deformity, and absent of rays in the foot. When associated with facial dysmorphism (small nose, micrognathia, and cleft palate) and other variable features such as renal anomalies, the condition is known as femoral hypoplasia-unusual facies syndrome (also known as femoral facial syndrome).58,62 A sporadic disorder known as femur-fibula-ulna syndrome should be suspected when unilateral hypoplasia or aplasia of the femur is associated with hypoplasia or aplasia of the fibula and defects of the ulna and ulnar rays (Table 23-7).47,49,58
Figure 23-17.
Right proximal femoral focal deficiency, type A. A: Three-dimensional rendered computerized tomography image at maturity shows shortened right femur and coxa vara deformity of the femoral neck. B: Besides the coxa vara, the AP radiograph of the pelvis shows moderately dysplastic acetabulum and femoral epiphysis.


Figure 23-19.
A, B: Three-dimensional surface-rendered images showing discrepancy between length, position and appearance of the lower limbs. C. Appearance of the fetus after termination of pregnancy at 19 weeks’ gestation showing typical posture of congenital deficiency of the femur. D: Radiograph demonstrating unilateral short femur and acetabular dysplasia. Arrow in each figure part indicates short femur.

OMIM# | Inheritance | Phenotypic Anomalies* | |
---|---|---|---|
Acrodysplasia with ossification abnormalities, short stature, and fibular hypoplasia | 603740 | AR | Fibular hypoplasia, epiphyseal changes at multiple sites, acrodysplasia of hands and feet, spine, and pelvic anomalies |
Acrofrontofacionasal dysostosis | 201180 | AR | Mesomelic limb shortening (including fibular hypoplasia), foot abnormalities, brachycephaly, malar hypoplasia, prognathism (some patients), hypertelorism, broad nasal bridge, cleft lip/palate, lordosis or scoliosis, hypoplastic iliac wings, and acetabular dysplasia |
Camptodactyly syndrome, Guadalajara, type I | 211910 | AR | Fibular hypoplasia, cubitus valgus, brachydactyly, camptodactyly 2nd-5th fingers, feet syndactyly, hypertelorism, depressed nasal bridge, midface hypoplasia, microcephaly, FGR |
Craniosynostosis with fibular aplasia | 218550 | AR | Fibular aplasia and craniosynostosis |
Femur-fibula-ulna syndrome | 228200 | Sporadic | Hypoplasia/aplasia of the femur, fibula, amelia/peromelia at the lower end of the humerus, humeroradial synostosis, defects of the ulna and ulnar rays |
Fibular hypoplasia and complex brachydactyly (Du Pan syndrome) | 228900 | AR | Absent fibulae, dislocated patella, complex brachydactyly, clubfeet, ball-like toes, short metatarsals |
Fibular aplasia, tibial campomelia, and oligosyndactyly | 246570 | AD | Fibular aplasia, tibial campomelia, and oligosyndactyly |
Santos syndrome | 613005 | AD, AR? | Fibular agenesis or hypoplasia, clubfeet, oligodactyly, severe nail hypoplasia or Anonychia sometimes associated with brachydactyly and occasional preaxial polydactyly |
Congenital deficiency of the fibula is the second most common pathologic entity in the spectrum of congenital limb shortening. It is unilateral in 80% of the cases and more often involves the right side.61 Congenital fibular deficiency or fibular hemimelia may present as a smaller than normal fibula (type 1A), as partial absence of the fibula (proximal absence of 30% to 50% of the length of the fibula with the distal portion present but no supported by the ankle—type 1B), or as complete absence of the fibula or only a small vestigial fragment (type 2) (Figure 23-20A).63 The disorder is frequently associated with other deformities of the lower extremity, including shortening and bowing of the tibia, postaxial oligodactyly, tarsal coalitions, ball-and-socket joint, talipes equinovarus or equinovalgus (Figure 23-20B), femoral deficiencies (PFFD in 15%-70% of the cases), and knee abnormalities (Figure 23-20C).61 Several syndromes may be associated with congenital fibular deficiency, as seen on Table 23-7.47,49 Treatment depends on the severity of the disorder and associated anomalies, including nonsurgical approach for mild forms (type IA), lengthening procedures with or without contralateral tibial epiphysiodesis (type IA or IB with a functional foot), or amputation (type II without a functional foot).47
Figure 23-20.
A: Congenital fibular deficiency (fibular hemimelia) characterized by only a small vestigial fragment. The calcaneus is dislocated posteriorly and medially from the distal epiphysis of the tibia. B: Dedicated 3DUS image of the foot shows associated clubfoot. C: High resolution magnified image of the knee shows medial dislocation at the knee, with the cartilaginous epiphysis of the femoral condyle articulating preferentially with the fibula. Findings are consistent with type II fibular hemimelia.



Tibial deficiency is less common than fibular deficiency. In partial tibial deficiency, a proximal fragment is more commonly found. The disorder is frequently associated with ipsilateral varus deformity, medial foot deficiency, as well as hypoplasia/aplasia of the fibula. Table 23-847,49 describes several conditions associated with tibial deficiency.
OMIM# | Inheritance | Phenotypic Anomalies* | |
---|---|---|---|
Acromesomelic frontonasal dysplasia | 603671 | AD | Rare variant of frontonasal dysplasia, variable CNS malformations, cleft lip and palate, and limb defects including tibial hypoplasia/aplasia, clubfeet, preaxial polydactyly of hands and feet, preaxial polydactyly of feet |
Brachyphalangy, polydactyly, and tibial aplasia/hypoplasia | 609945 | Sporadic | Absent tibia, complex preaxial polydactyly, syndactyly and brachydactyly, microcephaly, micrognathia, microphthalmia, hypertelorism, broad nasal bridge, high-arched palate, torticollis, CHD, micropenis, hypospadias, horseshoe kidney, parietal foramina, short radius and ulna, clubfeet |
Clubfoot, congenital, with or without deficiency of long bones and/or mirror-image polydactyly | 119800 | AD | Preaxial polydactyly, clubfeet |
Mesomelic dysplasia, Savarirayan type | 605274 | AR | Hypoplastic tibia, hypoplastic/absent fibula, hypoplastic acetabular roof, widened sacro sciatic notch, equinovalgus feet |
Orofacial digital syndrome type IV | 258860 | AR | Severe tibial dysplasia, preaxial and postaxial polydactyly, micrognathia, hypertelorism, cleft/high-arched palate, pectus excavatum, tongue hamartoma, cerebral atrophy, porencephaly |
Tibia, hypoplasia of, with polydactyly (includes Werner mesomelic dysplasia) | 188710 | AD | Hand polydactyly, digitalization of the thumb, short lower limbs with aplasia or the tibias, polydactyly of toes, CHD |
Tibial hemimelia | 275220 | AR | Deficiency of the tibia with relatively intact fibula; may be isolated or associated with other skeletal and extraskeletal malformations |
Humeral deficiency is rarely isolated and is usually part of disorders with more extensive limb deficiencies (see femur-fibula-ulna syndrome in Tables 23-7, 23-8, 23-9, 23-10 radiohumeral fusions with other skeletal and craniofacial anomalies in Table 23-9, facioauriculoradial dysplasia [phocomelia-ectrodactyly, ear malformation, deafness, and sinus arrhythmia] in Table 23-11 and acheiropody, aprosencephaly syndrome, mesomelic dysplasia Kantaputra type, and microgastria-limb reduction defects association in Table 23-12).47,49
OMIM# | Inheritance | Phenotypic Anomalies* | |
---|---|---|---|
Syndromes with blood dyscrasias | |||
Diamond-Blackfan anemia | 105650 | AD | Hypoplastic anemia, FGR. 30%-50% have structural anomalies, including microcephaly, micrognathia, hypertelorism, flat nose, cleft lip/palate, high-arched palate, webbed neck, ASD, VSD, coarctation of the aorta, 11 rib pairs, parietal foramina, vertebral anomalies, mild radial hypoplasia, triphalangeal/bifid/hypoplastic/absent thumbs |
Fanconi’s anemia | 227650 300514 | AR X-linked | 60%-75% of affected individuals: radial aplasia, thumb deformity/aplasia/hypoplasia/duplication, renal anomalies, congenital heart defect, microcephaly, microphthalmia |
Thrombocytopenia w/absent radius (TAR) | 274000 | AR | Thrombocytopenia, absent radius, hypoplastic or absent ulna, thumb always present (distinctive feature). Also: brachycephaly, micrognathia, small upturned nose, TOF, ASD, VSD, coarctation of the aorta, spina bifida, hip dislocation, hypoplastic cerebellum absent cavum septum pellucidum, dysgenesis of the corpus callosum |
WT limb-blood syndrome | 194350 | AD | Radioulnar synostosis, hypoplastic anemia. Also, micrognathia, retrognathia, sensorineural hearing loss |
Syndromes with congenital heart disease | |||
AVSD w/blepharophimosis and radial defects | 600123 | AR | FGR, micrognathia, microphthalmia, broad nasal bridge, AVSD, TOF, ASD, VSD, imperforate anus, VUR, radial aplasia, short ulna, 5th finger clinodactyly, hypoplastic/absent thumb, absent first metacarpal |
Holt-Oram syndrome | 142900 | AD | Characteristic: thumb anomaly (absent, triphalangeal, nonopposable, finger-like digit; first metacarpal with proximal and distal epiphyses) and atrial septal defect. Upper extremity anomaly may be more severe, including upper extremity phocomelia, radial and ulnar anomalies. Heart defects also include VSD and HLHS. Other anomalies: pectus excavatum or carinatum, absent pectoralis muscle, vertebral anomalies, and scoliosis |
Syndromes with craniofacial anomalies | |||
Acrofacial dysostosis 1, Nager Type | 154400 | AD | Malar hypoplasia causing midface retrusion and micrognathia requiring tracheostomy in early childhood; cleft lip/palate; small/absent thumbs, triphalangeal thumbs, radial hypoplasia or aplasia, radioulnar synostosis; occasionally upper limb phocomelia and lower-limb defects; conductive deafness, external auditory canal atresia; occasionally CHD; scoliosis; unilateral renal agenesis; hydrocephalus, polymicrogyria |
Baller-Gerold syndrome | 218600 | AR | Craniosynostosis (coronal, metopic, lambdoid) and radial aplasia. Overlaps with Saethre-Chotzen syndrome. Additional features: flattened forehead, micrognathia, hypertelorism, prominent nasal bridge, microstomia, high palate, CHD, anorectal malformations, renal anomalies, vertebral anomalies, absent or hypoplastic radii, absent or hypoplastic thumbs, absent carpal/metacarpal/phalanges, short and curved ulna |
Cleft lip/palate with abnormal thumbs and microcephaly (aka Jugberg-Hayward, orocraniodigital syndrome) | 216100 | AR | Cleft lip and palate, hypertelorism, aplastic/hypoplastic thumb, short radius, radial head subluxation, minor vertebral and rib anomalies, horseshoe kidneys, toe anomalies |
Cornelia de Lange | 122470 300590 | AD, also XLD (HDAC8- and SMC1A-related | Microbrachicephaly, facial dysmorphism (synophrys, long eyelashes, depressed nasal bridge, short nose with anteverted nares, high arched and cleft palate 30%, micrognathia 80%), FGR, upper limb reduction defects ranging from subtle phalangeal anomalies to oligodactyly, cardiac septal defects, hypoplastic genitalia |
Duane–radial ray syndrome (aka Okihiro syndrome, acrorenal-ocular syndrome) | 607323 | AD | Allelic disorder to IVIC syndrome, with similar phenotype. Characterized by ocular anomalies, radial ray deficiency, and renal anomalies (in some cases). Duane anomaly is a congenital and nonprogressive strabismus condition, characterized by inability to abduct and eyeball retraction with adduction. Multiple other anomalies may be present, including facial, cardiac, gastrointestinal, genitourinary, and vertebral anomalies. |
Hemifacial microsomia with radial defects (Goldenhar syndrome with ipsilateral radial defect) | 141400 | AD | Hemifacial microsomia, skin tags at mandibular angle, short mandibular ramus, microtia, external auditory canal atresia, multiple preauricular ear tags and pits, oral cleft, radial limb defects, triphalangeal thumbs, thumb duplication |
IVIC (Instituto Venezuelano de Investigaciones Cientificas) syndrome | 147750 | AD | Allelic to Duane-radial ray syndrome (OMIM 607323), characterized by radial ray deficiency, hypoplastic or absent thumbs and radial clubhands, impaired hearing, abnormal movements of extraocular muscles with strabismus. Also possible: imperforate anus, rectovaginal fistula, scoliosis, radioulnar synostosis, deltoid and pectoralis major hypoplasia. |
LADD (lacrimoauriculodentodigital) syndrome | 149730 | AD | Absent lacrimal structures, protuberant ears, abnormal teeth, short radius, short ulna, preaxial polydactyly, bifida thumb, digitalized thumb, triphalangeal thumb, syndactyly (2-3 fingers), clinodactyly (3, 5 fingers), broad halluces, toe syndactyly |
Radial aplasia, X-linked | 312190 | X-linked | Radial aplasia, hydrocephalus, hypospadias, imperforate anus |
Radial hypoplasia, triphalangeal thumbs, hypospadias, and maxillary diastema | 179250 | AD | Nonopposable triphalangeal thumbs, radial hypoplasia, hypospadias, anterior maxillary diastema |
Radial ray deficiency, X-linked | 300378 | X-linked | Ulnar hypoplasia, absent patellae, congenital heart disease, Dandy-Walker malformation |
Radiohumeral fusions with other skeletal and craniofacial anomalies | 614416 | AR | Radiohumeral fusion, oligodactyly, craniosynostosis, occipital encephalocele |
Radius aplasia with cleft lip/palate | 179400 | No genetic information | Radial aplasia, cleft lip/palate |
Robin sequence with cleft mandible and limb anomalies (Richieri-Costa-Pereira syndrome) | 268305 | AR | Robin sequence, cleft mandible, minor ear anomalies, hallux hypoplasia, may have ulnar, tibial, and fibular hypoplasia, clubfeet. Common learning disability. |
Rothmund–Thomson syndrome | 268400 | AR | Frontal bossing, prognathism, microphthalmia, forearm reduction defects, absent thumbs, clubfeet, absent patella, prematurely aged skin changes (see progeria syndromes) |
Smith-Lemli-Opitz syndrome | 270400 | AR | FGR, microcephaly, microcephaly, dysmorphic facies (hypertelorism, anteverted nares), cleft palate, 2-3 toe syndactyly, postaxial polydactyly, genital anomalies (hypospadias in males), congenital heart disease, renal agenesis, hydronephrosis |
Chromosomal anomalies | |||
Trisomy 18 | FGR, low-set ears, micrognathia, clenched hands with overlapping fingers, congenital heart disease, renal anomalies | ||
Spinal anomalies | |||
VACTERL | 192350 | Sporadic | Nonrandom association of anomalies, including vertebral anomalies, anal atresia, cardiovascular anomalies, tracheoesophageal fistula, esophageal atresia, renal anomalies, and preaxial limb anomalies |
See also: Diamond-Blackfan anemia, Baller-Gerold syndrome, Holt-Oram syndrome, Cleft lip/palate with abnormal thumbs and microcephaly, Duane radial ray syndrome, | See descriptions above |
OMIM# | Inheritance | Phenotypic Anomalies* | |
---|---|---|---|
Acromesomelic dysplasia | 609441 | AR | Short ulna, fibular aplasia, widened proximal tibial metaphyses, severe brachydactyly, radially deviated fingers, clubfeet, Müllerian anomalies |
Cornelia de Lange syndrome | 122470 300590 | AD, also XLD (HDAC8- and SMC1A-related | Microbrachicephaly, facial dysmorphism (synophrys, long eyelashes, depressed nasal bridge, short nose with anteverted nares, high arched and cleft palate 30%, micrognathia 80%), FGR, upper limb reduction defects ranging from subtle phalangeal anomalies to oligodactyly, cardiac septal defects, hypoplastic genitalia |
Femur-fibula-ulna syndrome | 228200 | Sporadic | Hypoplasia/aplasia of the femur, fibula, amelia/peromelia at the lower end of the humerus, humeroradial synostosis, defects of the ulna and ulnar rays |
Fibuloulnar aplasia with renal anomalies | 228940 | AR | Symmetric mesomelic limb shortening, fibular aplasia, hypoplastic ulna, renal anomalies, micrognathia, congenital heart disease (truncus arteriosus, VSD) |
Fibular aplasia or hypoplasia, femoral bowing and poly-,syn-, and oligodactyly (Fuhrmann syndrome) | 228930 | AR | Hypoplastic/aplastic ulnae, forearm bowing, femoral bowing, Fibular hypoplasia/aplasia, polydactyly, syndactyly, oligodactyly, clubfeet |
Hypoplasia of ulna and fibula (Reinhardt-Pfeiffer mesomelic dysplasia) | 191400 | AD | Hypoplasia of ulna and fibula; also abnormal radius, tibia, and adjacent portion of the skeleton |
Langer mesomelic dysplasia | 249700 | AR | Severe limb aplasia or severe hypoplasia of the ulna and fibula and thickened and curved radius and tibia, displacement deformities of the hands and feet |
Ophthalmomandibulomelic dysplasia (Pillay syndrome) | 164900 | AD | Absent distal third of ulna, absent olecranon, hypoplastic trochlea and proximal radius, fusion of interphalangeal joints in ulnar fingers, knee dysplasia; corneal opacities, fusion of temporomandibular joint |
Postaxial acrofacial dysostosis (Miller syndrome) | 263750 | AR | Ulnar hypoplasia, radial hypoplasia, radioulnar synostosis, syndactyly, thumb hypoplasia, absence of fifth finger and/or toe, severe micrognathia, cleft lip and/or palate, micropenis, renal anomalies |
Ulna and fibula, absence of, with severe limb deficiency | 276820 | AR | Hypoplasia of femur and fibula, oligodactyly |
Ulnar agenesis and endocardial fibroelastosis | 276822 | AR | Endocardial fibroelastosis, oligodactyly |
Ulnar/fibular ray defect and brachydactyly | 608571 | AD | Fibular hypoplasia/aplasia, brachydactyly, midface hypoplasia |
Ulnar hypoplasia with lobster claw deformity feet | 314360 | X-linked recessive versus sex-influenced AD | Ulnar hypoplasia, hypoplastic fingers 2 to 5, split-foot deformity |
Ulnar hypoplasia | 191440 | AD | Hypoplasia of the ulna with severe radial bowing |
Ulnar hypoplasia with mental retardation | 276821 | AR | Hypoplastic ulna, clubfeet, and anonychia congenita; intellectual disability |
Ulnar-mammary syndrome (Schinzel syndrome) | 181450 | AD | Hypoplasia of ulna and ulnar rays; hypoplasia of the breast and absence of apocrine sweat gland; hypoplastic scapulae and clavicles; small penis, imperforate hymen, anorectal malformations, VSD, arrhythmia |
Ulnar ray dysgenesis with postaxial polydactyly and renal cystic dysplasia | 604380 | ?AR | Ulnar ray dysgenesis, oligodactyly, polydactyly, dysplastic kidneys |
Weyers ulnar ray/oligodactyly syndrome | 602418 | AD | Single central upper incisor, cleft lip and/or palate, high arched palate, hypotelorism, deficiency of ulna and ulnar rays, short radii, oligodactyly, absent thumb, hydronephrosis, cardiac anomalies, antecubital webbing, cleft lip and palate, short sternum |
OMIM# | Inheritance | Phenotypic Anomalies* | |
---|---|---|---|
Split-hand/foot malformation | 183600 313350 246560 605289 606708 225300 | AD X-linked AD AD ? AR | Syndactyly, median clefts of the hands and feet, aplasia and/or hypoplasia of the phalanges, metacarpals, and metatarsals. Also known as ectrodactyly and “lobster claw” deformity |
Split-hand/foot malformation with other associated anomalies | |||
Acrodermatoungual- lacrimal-tooth (ADULT) syndrome | 103285 | AD | Split-hand/foot malformation and several other traits that are not detectable prenatally except for lacrimal duct atresia (eg, nail dysplasia, mammary hypoplasia, hypodontia, alopecia) |
Anonychia-ectrodactyly | 106900 | AD | Split-hand/foot malformation, anonychia. |
Brachydactyly-ectrodactyly with fibular aplasia or hypoplasia | 113310 | AD | Split-hand/foot malformation associated with fibular aplasia/hypoplasia |
Cleft palate, cardiac defect, genital anomalies, and ectrodactyly (Acrocardiofacial syndrome) | 600460 | AR | Split-hand malformation, cleft lip/palate, CHD, genital anomalies; also hypertelorism, micropenis/hypospadias |
Ectrodactyly-cleft palate syndrome | 129830 | AD | Split-hand/foot malformation and cleft palate without cleft lip of the ectodermal features that occur in EEC syndrome |
Ectrodactyly and ectodermal dysplasia without cleft lip/palate | 129810 | AD | Split-hand/foot malformation, ectodermal dysplasia (hypotrichosis, abnormal teeth) but no cleft lip/palate |
Ectrodactyly, ectodermal dysplasia, and cleft lip/palate (EEC) syndrome | 129900 | AD | Split-hand/foot malformation, ectodermal dysplasia (hypotrichosis, oligodontia), cleft lip/palate, maxillary/malar hypoplasia, genitourinary anomalies (micropenis, transverse vaginal septum, renal agenesis/dysplasia/hydronephrosis/duplex collecting system/megaureter, semilobar holoprosencephaly |
Ectrodactyly of lower limbs, congenital heart disease, and micrognathia | 601348 | Sporadic | Split-foot malformation, CHD, micrognathia |
Ectodermal dysplasia, ectrodactyly, and macular dystrophy syndrome | 225280 | AR | Split-hand malformation, syndactyly, camptodactyly, ectodermal dysplasia, macular dystrophy |
Phocomelia-ectrodactyly, ear malformation, deafness, and sinus arrhythmia (facioauriculoradial dysplasia) | 171480 | AD | Hypoplastic humerus, absent radius/asymmetric radial dysplasia, ulnar bowing, radioulnar synostosis, hypoplastic fibulae, mid face hypoplasia, ear deformities, anterior vertebral fusion, hypoplastic to aplastic thumbs, rudimentary 2nd metacarpal, clubhand, absent second finger |
Femur, unilateral bifid, with monodactylous ectrodactyly | 228250 | AR | Split-hand malformation, monodactyly of hand, absent ulna, monodactyly of feet, absent tibia, bifurcated femur |
Focal dermal hypoplasia (Goltz or Goltz-Gorlin syndrome) | 305600 | X-linked dominant (male lethal) | Multisystem disorder, affecting skin, skeletal system (oligo/syndactyly, split hand/foot malformation), eyes (anophthalmia/microphthalmia, iris and chorioretinal coloboma, lacrimal duct abnormalities) and face (facial asymmetry, cleft lip/palate, pointed chin). Occasionally, dental anomalies, abdominal wall defects, diaphragmatic hernia, intestinal malrotation, and renal anomalies |
Hypomelia with Mullerian duct anomalies (limb-uterus syndrome) | 146160 | AD | Upper limb hypoplasia, split-hand malformation, Mullerian anomalies |
Limb-mammary syndrome | 603543 | AD | Split hand/foot malformation, syndactyly, camptodactyly, mammary hypoplasia/aplasia; also cleft palate, lacrimal duct atresia |
Microphthalmia, syndromic | 601349 | ?AD | Split foot malformation, microphthalmia, cleft lip/palate, borderline microcephaly |
Split-foot deformity with mandibulofacial dysostosis | 183700 | AD | Split-hand/foot deformity, syndactyly of toes, Robin sequence, abnormal ears, hearing loss |
Split-hand with congenital nystagmus, fundal changes and cataracts (Karsh- Neugebauer) syndrome | 183800 | AD | Split-hand/foot malformation, monodactyly, cataract, congenital nystagmus |
Split-hand with obstructive uropathy, spina bifida and diaphragmatic defects | 183802 | AD | Split-hand/foot malformation, cutaneous syndactyly, spina bifida, thoracolumbar scoliosis, renal anomalies (hydronephrosis, ureteral atresia, megaloureter, obstructive uropathy, diaphragmatic defect, hydrocephaly |
Split-hand/foot malformation 1 with sensorineural hearing loss | 220600 | AR | Split-hand/foot malformation and sensorineural hearing loss; may have dysmorphic facies and abnormal ears; additionally, mild scoliosis (rare), asymmetrically short and deformed legs in some patients |
Split-hand/foot malformation with long-bone deficiency 1 | 119100 | AD? | Split-hand/foot malformation, tibial and distal femoral hypoplasia, may be bifurcated |
Split-hand/foot malformation with long-bone deficiency 2 | 610685 | ? | Split-hand/foot malformation with tibial hypoplasia |
Split-hand/foot malformation with long-bone deficiency 3 | 246560 | AD | Split-hand/foot malformation, cleft or high-arched palate, dysplastic ears, some patients with maxillary hypoplasia and micrognathia |
Split-hand/foot with hypodontia | 183500 | ?AD | Split-hand/foot malformation, hypodontia (may be part of EEC spectrum) |
Triphalangeal thumbs with brachyectrodactyly | 190680 | AD | Split-hand/foot malformation, triphalangeal thumbs, brachydactyly of index fingers and third toes |
Ulnar hypoplasia with lobster-claw deformity of feet | 314360 | X-linked | Complete absence/hypoplasia of the ulna, hypoplastic fingers 2-5 (may have split-hand), split-foot |
OMIM# | Inheritance | Phenotypic Anomalies* | |
---|---|---|---|
Acheiropody | 200500 | AR | Bilateral congenital amputations of the upper and lower extremities associated with aplasia of the hands and feet; specifically, amputation of distal humeral epiphysis, distal tibial diaphysis, and aplasia of radius, ulna, fibula, carpal, metacarpal, tarsal, metatarsal, and phalangeal bones |
Acrofacial dysostosis syndrome of Rodriguez | 201170 | AR | Mandibular hypoplasia, microtia, cleft palate, upper limb phocomelia/hypoplasia and oligodactyly, short tibia, absent fibula, clubfeet, arrhinencephaly, abnormal lung lobulation |
Acromesomelic dysplasia, Hunter-Thompson type | 201250 | AR | Hypoplastic ulna, tibia, fibula, small fingers and toes. Joint dislocations. Gradient of severity: proximal to distal. Normal craniofacial and axial skeleton. Allelic to Grebe syndrome, brachydactyly type C, fibula hypoplasia and complex brachydactyly |
Amelia, autosomal recessive | 601360 | AR | Amelia of the upper extremities, severe underdevelopment of the legs; may also have intestinal defects, some renal and genital defects, oral clefts, defects of cardiac septa, and anencephaly |
Anonychia-onchodystrophy with hypoplasia or absence of distal phalanges (Cooks syndrome) | 106995 | AD | Brachydactyly of the 5th finger, triphalangeal thumb, hypoplasia/aplasia of distal phalanges, nail dysplasia, or anonychia; may also have high arched palate, high nasal bridge, and prominent nose |
Aprosencephaly | 207770 | AR | Aprosencephaly/anencephaly; fusion of the humerus and radius, oligodactyly |
Brachial amelia, cleft lip, holoprosencephaly | 601357 | Sporadic | Association of holoprosencephaly, facial clefting and bilateral amelia of the upper limbs. CNS anomalies may also be smooth brain surface, absence of corpus callosum and/or septum pellucidum, and encephalocele. Additional anomalies include femoral and fibular hypoplasia, oligodactyly, omphalocele, and CHD. |
Chondrodysplasia, Grebe type | 200700 | AR | Severe limb shortening progressing in a proximal-distal gradient. Hands and feet most severely affected. Invariably absent proximal and middle phalanges of fingers and toes; distal phalanges present given fingers a “globular” appearance. |
CHARGE syndrome | 214800 | AD | Coloboma, heart defects, atresia of the choanae, retarded growth and development, genital anomalies, ear anomalies; may also have microphthalmos, orofacial clefts, tracheoesophageal fistula, and, in some cases, a variety of limb deficiencies, including monodactyly, ulnar hypoplasia, tibial aplasia, bifid femur, and radial aplasia |
Faciocardiomelic dysplasia | 227270 | AR | Radial, ulnar, tibial, fibular, digital hypoplasia; micrognathia/retrognathia; microglossia; microstomia; congenital heart disease |
Hypoglossia-hypodactylia (Hanhart syndrome) | 103300 | Sporadic | Hypoplastic mandible, absence of lower incisors, hypoglossia, and variable degree of absence of digits and limbs |
Laryngeal atresia, encephalocele, limb deformities | 601732 | AR | Laryngeal atresia, anterior encephalocele, and limb deformities, including radial, ulnar, tibial hypoplasia, and oligodactyly; may also have renal and CNS anomalies (agyria) |
Mesomelic dysplasia, Kantaputra type | 156232 | AD | Symmetric marked shortening of the upper and lower limbs, very short ulnae and bowed radii; dumbbell shaped humeri; relatively normal hand with progressive flexion contractures of the proximal interphalangeal joints |
Mesomelic dysplasia, Langer type | 249700 | AR | Severe limb aplasia or severe hypoplasia of ulna and fibula. Thckened and curved radius and tibia. May have micrognathia. |
Microgastria-limb reduction defects association | 156810 | Sporadic | Radial and ulnar hypoplasia, upper limb phocomelia, terminal transverse defect of humerus, absent thumbs, oligodactyly, microgastria, hypoplastic lungs, genitourinary anomalies. May also have CNS anomalies, anophthalmia, CHD, abnormal lung lobation, absent gallbladder, asplenia, hyposplenia, splenogonadal fusion, intestinal malformation. |
Nievergelt syndrome | 163400 | AD | Hypoplasia of the radius, ulna, tibia, fibula, radioulnar synostosis |
Orofacial digital syndrome X | 165590 | AD | Facial (telecanthus, flat nasal bridge, retrognathia), oral (cleft palate), and digital (oligodactyly, preaxial polydactyly), radial shortening, fibular agenesis, coalescent tarsal bones |
Roberts syndrome | 268300 | AR | Growth restriction, symmetric mesomelic shortening of the the limbs with upper limbs more commonly and severely affected than lower limbs. May have tetraphocomelia and oligosyndactyly. Microcephaly, cleft lip/palate, CHD. |
Skeletal defects, genital hypoplasia, mental retardation | 612447 | AR | Hypoplasia of all segments, hypoplastic clavicle and scapula, oligodactyly, genital hypoplasia, microcephaly, dysmorphic facies, intellectual disability |
Tetramelic monodactyly | 187510 | AD | Only fifth digit present on each limb |
Tukel syndrome | 609428 | AR | Postaxial oligosyndactyly, ophthalmoplegia |
Radial deficiencies are often associated with hypoplasia or aplasia of the thumb, absence of preaxial carpal bones (scaphoid and trapezium) and bowing of the ulna, leading to radial club hand deformity (Figure 23-21). A list of conditions associated with radial deficiency are listed on Table 23-9).47,49 The most commonly encountered conditions in clinical practice are trisomy 18, Cornelia De Lange syndrome, thrombocytopenia–radial aplasia (TAR) syndrome, Fanconi anemia, Holt-Oram syndrome, and VATER/VACTERL association.47
Figure 23-21.
Radial club hand caused by radial deficiency in a fetus with hemifacial microsomia (Goldenhar syndrome). A: Three-dimensional rendered image shows mesomelic shortening of the right forearm and radial clubhand. B: Corresponding postnatal image. C: A view from the dorsal aspect of the hand shows absent thumb and syndactyly between the second and third fingers. (Reproduced with permission from the Perinatology Research Branch of the Eunice Kenney Shriver National Institute of Child Health and Human Development, NIH, DHHS.)



Ulnar deficiency leads to ulnar clubhand. Ulnar deficiency is less common than radial deficiency, and severity ranges from mild deviation of the hand to complete absence of the ulna. Table 23-1047,49 provides a list of disorders associated with ulnar deficiency.
The terms split-hand and split-foot refer to a group of disorders characterized by splitting of the hand and/or foot into 2 parts (Figures 23-21 and 23-22). Other terms include lobster claw deformity, ectrodactyly, and aborted fingers.47 The conditions are classified into typical and atypical.64 The typical form consists of absence of both finger and metacarpal bones, resulting in a deep V-shaped central defect that clearly divides the hand into an ulnar and a radial part. This form occurs in 1 per 90,000 live births and has a familial tendency (usually inherited with an autosomal dominant pattern).65 In contrast, the atypical variety is characterized by a much wider cleft formed by a defect of the metacarpals and the middle fingers. As a consequence, the cleft is U-shaped and wide, with only thumb and small finger remaining. It occurs in 1 per 150,000 live births.65 In its most severe form, monodactyly, the hand or foot have only a single digit.47
Split-hand-and-foot deformities can occur in isolation or as part of more complex syndromes. The most common of these is ectrodactyly, ectodermal dysplasia, and cleft lip/palate (or EEC) syndrome, an autosomal dominant condition generally involving the 4 extremities, with more severe deformities seen in the hands.66,67 The spectrum of ectodermal defects is wide and includes hypopigmentation, dry skin, sparse hair, and dental defects.68-71 Tear duct anomalies and decreased lacrimal secretions may lead to chronic keratoconjunctivitis and severe loss of visual acuity.72,73 Cleft lip is generally bilateral,67 obstructive uropathy is frequent,74 and intelligence is generally normal.75 Table 23-11 provides a comprehensive list of syndromes, inheritance pattern, and main phenotypic features of syndromes associated with split hand/split foot malformations.47,49
In phocomelia, the extremities resemble those of a seal (Figure 23-24). Typically, the hands and feet are present, but the intervening arms and legs are absent. Hands and feet may be normal or abnormal. Three syndromes should be primarily considered in the differential diagnosis of phocomelia: Roberts syndrome,76 some varieties of the thrombocytopenia with absent radius (TAR) syndrome,77 and Grebe dysplasia.78 Phocomelia may also be caused by exposure to thalidomide, but this is only of historical interest.77,79
Figure 23-23.
Gross photograph of same neonate as in Figure 23-22 at birth, confirming ectrodactyly of the hand.

Syndromes with multiple limb deficiencies are listed on Table 23-12.47,49 Syndromes with bilateral, symmetric upper and lower limb involvement are usually classified with the mesomelic dysplasias. Roberts phocomelia (also known as SC phocomelia or pseudothalidomide syndrome) is a lethal autosomal recessive skeletal dysplasia caused by mutations in the gene ESCO2 (establishment of cohesion 1 homolog 2). The phenotype is characterized by symmetric mesomelic tetraphocomelia with the upper limbs more often and more severely affected than the lower limbs. The radius is always affected, and the thumbs are more often involved compared to the other digits. Craniofacial dysmorphism includes microcephaly (or macrocephaly in case of hydrocephalus), brachycephaly, exophthalmos, hypertelorism, hypoplastic nasal alae, malar hypoplasia, micrognathia, cleft lip and palate, and ear anomalies. Additional abnormalities include horseshoe kidney, ventriculomegaly, cephalocele, and spina bifida. Roberts phocomelia can be diagnosed by standard cytogenetic preparations stained with Giemsa or C-banding techniques showing premature centromere separation (PCS) and heterochromatin repulsion (HR) and/or by sequence analysis for the ESCO2 gene.76
Vertebral anomalies can occur in isolation or as part of association of anomalies or syndromes. They result from failure of formation of the vertebrae, abnormal segmentation, or both. Specific types of vertebral anomalies are described in Table 23-13.80 Examples of butterfly vertebra, hemivertebra and vertebral fusion are shown in Figure 23-25.
Agenesis | Occurs despite the presence of posterior elements Ossification centers of the body fail to appear |
Hemivertebra | Lack of ossification of one-half of the body leads to a unilateral wedge vertebra Lateral hemivertebrae result in scoliosis, frequently present at birth Dorsal hemivertebrae leads to kyphotic deformity Ventral hemivertebra are extremely rare |
Butterfly vertebra | Also known as sagittal cleft vertebrae Result from failure of fusion of the lateral halves of the vertebral body Persistent notochordal tissue between the two halves Anterior spina bifida may be associated with a butterfly vertebra |
Block vertebra | Failure of segmentation leads to congenital vertebral fusion Fusion may be complete or partial Usually occurs in lumbar and cervical segments |
Coronal cleft | Failure of fusion of the anterior and posterior ossification centers Unfused elements are separated by a cartilage plate Most clefts disappear spontaneously by six months after birth More common in lower thoracic and lumbar spine |
Hypoplasia | May result from vascular insufficiency during fetal life One or more vertebrae may be affected |
Platyspondyly | Flattened vertebral bodies with normal disk heights |
Figure 23-25.
Coronal CT reformat displayed with bone window showing multiple segmentation abnormalities of the thoracic spine leading to a dextroconvex scoliosis. Arrows: butterfly vertebrae. Arrowheads: lateral hemivertebrae. Stars: hemivertebra associated with persistence of the right and left halves of the vertebral bodies, leading to the hemivertebrae being separated from each other in the sagittal plane; the right hemivertebra fuses with the contralateral body of the vertebral segment below.

Whenever segmentation abnormalities of the spine are encountered, the examiner should focus on identifying specific anomalies that may point to associations and syndromes. The most common association seen with spinal abnormalities is VACTERL, an acronym that stands for the nonrandom occurrence of Vertebral (60%-80%, including hemivertebra, congenital scoliosis, caudal regression or spina bifida), Anal (55%-90%, including imperforate anus/anal atresia), Cardiac (40%-80%), Tracheo-Esophageal (50%-80%), Renal (50%-80%), and Limb abnormalities (40%-50%, including radial ray anomalies, polydactyly and oligodactyly). VACTERL is a highly heterogeneous disorder diagnosed when a cluster of at least three components of the association are present. It is considered by most as a sporadic condition, with no known underlying genetic defect, and with an incidence of approximately 1 per 10,000 to 1 per 40,000 births.81 Vertebral segmentation anomalies are a component of several other syndromic and nonsyndromic conditions, including but not limited to Aicardi syndrome (Figure 23-26), atelosteogenesis type 3, campomelic dysplasia, caudal regression syndrome, CHARGE syndrome (coloboma of the eye, Heart defects, Atresia of the choanae, Retardation of growth and/or development, Genital and/or urinary abnormalities, and Ear abnormalities and deafness), femoral hypoplasia-unusual facies syndrome, hemifacial microsomia (Goldenhar syndrome), Klippel Feil syndrome, OEIS syndrome (omphalocele, exstrophy of the cloaca, imperforate anus, and spina abnormalities), and spondylocostal dysostosis. Maternal diabetes, exposure to antiepileptic drugs, and maternal exposure to toxins (eg, carbon monoxide) may also cause congenital vertebral anomalies.82 Caudal regression syndrome is frequently seen in cases of poorly controlled maternal diabetes during embryogenesis and is characterized by agenesis of varying portions of the lumbar and sacral spine.83
Figure 23-26.
Three-dimensional US of the fetal brain and spine. A-C: Axial (A), coronal (B), and sagittal (C) reformats of the brain in a 20-week fetus show a pointed and splayed appearance of the anterior horns of the lateral ventricles, absence of the cavum septi pellucidi, elevated third ventricle (arrowhead), tear-drop lateral ventricles, and an interhemispheric cyst (*). Note that these findings are mainly appreciated on (A) and that the reconstructed sagittal view (C) does not show features that would confirm the diagnosis of corpus callosum dysgenesis because of the image degradation on the reformatted elevation plane. D: Three-dimensional rendered US image of the fetal spine shows scoliosis associated with multiple segmentation anomalies. E: Axial reformat of the fetal face at the level of the orbits shows right microphthalmia (arrowhead). The constellation of findings led to prenatal diagnosis of Aicardi syndrome. (Reproduced with permission from Gonçalves LF. Three-dimensional ultrasound of the fetus: how does it help? Pediatr Radiol. 2016 Feb;46(2):177-189.)





Cranial abnormalities may present as decreased mineralization of the calvarium, as well as with widening or premature fusion of cranial sutures. In addition, small intrasutural bones typically found around the lambdoid sutures and known as wormian bones may be associated with several abnormal conditions.
As mentioned previously, decreased mineralization of the calvarium is usually suspected by observing unusually clear images of the brain hemisphere closest to the transducer on axial images of the fetal head (see Figures 23-7A and 23-8A) and further confirmed by noting easy compressibility of the parietal bone upon application of gentle transducer pressure (see Figure 23-8B). Decreased mineralization of the calvarium is characteristic of osteogenesis imperfecta type 2, but may also be seen in other disorders such as achondrogenesis type 1A, lethal hypophosphatasia, Astley-Kendall Dysplasia, and Greenberg dysplasia.22
Suture widening is typical of cleidocranial dysplasia, but may also occur in Crane-Heise syndrome, mandibuloacral dysplasia, pyknodysostosis, and hypophosphatasia.22 Similar to decreased mineralization, suture widening may manifest by prenatal ultrasound as unusually well seen brain in the hemisphere presenting closest to the transducer (see Figures 23-9A to C).84
Wormian bones may occur in normal individuals as well as in several syndromic and nonsyndromic disorders, the most common of which osteogenesis imperfecta and cleidocranial dysplasia. Other conditions include chondrodysplasia punctata, copper deficiency (Menkes syndrome), Down syndrome, hypophosphatasia, otopalatodigital syndrome, progeria, pyknodysostosis, rickets, and Zellweger syndrome.85 Prenatal diagnosis of wormian bones has been reported in fetuses with cleidocranial dysplasia,86 osteogenesis imperfecta,87 in a fetus with multiple anomalies associated with craniosynostosis,88 and in normal fetuses with no other associated anomalies.89
Premature fusion of one or more cranial sutures is known as craniosynostosis.90 The major cranial sutures are the sagittal, coronal, lambdoid, and metopic (Figure 23-27A). These sutures do not fuse until adulthood, with the exception of the metopic suture, which fuses between 3 and 9 months of age.91 Therefore, all major sutures should be patent in utero. Small or major segments of one or more sutures may be close prematurely in utero or after birth, resulting in alterations in head shape that vary according to the affected suture(s).92 When a suture closes prematurely, the skull cannot grow along the axis perpendicular to the suture and, instead, grows parallel to it. For example, in the setting of premature closure of the sagittal suture, skull growth in the longitudinal axis leads to an elongated head shape known as dolichocephaly or scaphocephaly (Figure 23-27B). Dolichocephaly can be objectively confirmed by calculating the cephalic index (CI), which consists of dividing the biparietal diameter (BPD) by the occipitofrontal diameter (OFD). A cephalic index less than 0.75 indicates dolichocephaly.93 Premature closure of both coronal sutures leads to brachycephaly (Figure 23-27C), which is seen as a round head shape given the wide BPD, with a cephalic index more than 0.85. Premature closure of the metopic suture leads to trigonocephaly, with the head shape characterized by a pointed and narrow forehead, a smaller frontal cranial fossa and a dense frontal ridge (Figure 23-28). In trigonocephaly, the medial orbital roofs are deviate superiorly and medially toward the prematurely closed suture, an imaging feature known as the “quizzical eye.” When all sutures close prematurely, the head shape is severely deformed and known as Kleeblattschädel or “cloverleaf” skull. Such severe deformity can be seen in several syndromes, including Pfeiffer and thanatophoric dysplasia type 2 (Figure 23-29). Unilateral closure of the coronal suture leads to anterior plagiocephaly, characterized by ipsilateral flattening of the forehead and elevation of the ipsilateral sphenoid wing and orbital roof (“Harlequin” eye deformity). Unilateral closure of the lambdoid suture leads to posterior plagiocephaly, characterized by ipsilateral occipital flattening and posterior displacement of the ipsilateral ear.
Figure 23-28.
A: Three-dimensional US rendered image of the fetal face shows premature closure of the metopic suture. B: Postnatal CT viewed with bone window shows abnormal head shape characterized by a pointed and narrow forehead and small anterior cranial fossa. C: Postnatal 3D CT reconstruction shows the metopic craniosynostosis and anterior osseous ridge to greater advantage.



The reader is advised to approach unusual calvarial morphology during fetal life with caution, since abnormal head shape is not synonymous with craniosynostosis.91 Indeed, approximately 14% of trisomy 21 fetuses have brachycephaly; however, the head shape of trisomy 21 is not caused by coronal craniosynostosis.94 Likewise, most fetuses presenting with dolichocephaly are normal, as head molding secondary to breech presentation and/or oligohydramnios frequently leads to dolichocephaly.95
Craniosynostosis has an estimated incidence of 1 per 2000 to 1 per 2500 live births, is associated with advanced paternal age, and females are more often affected than males by a 2:1 ratio.92 Craniosynostosis is often isolated, with syndromic forms accounting for 8% to 15% of the cases.90,91 The recurrence risk for nonsyndromic craniosynostosis is approximately double that of the general population.91 In a series of prenatally diagnosed cases spanning 10 years, Rubio et al92 found only 6 cases of syndromic craniosynostosis (3 cases of Apert syndrome, 2 cases of Pfeiffer syndrome, and 1 case of Carpenter syndrome), highlighting the low incidence of syndromic forms, estimated at 1 per 60,000 births for Crouzon syndrome and 1 per 65,000 to 1 per 160,000 births for Apert syndrome. The incidence of syndromic craniosynostosis is higher when the coronal sutures are prematurely closed (see Figure 23-14). Therefore, a diligent search for associated anomalies, including detailed evaluation of the hands and feet, is mandatory. The prototypical syndromes associated with coronal craniosynostosis are Apert’s, Crouzon, Pfeiffer, Muenke, Saethre-Chotzen, and Antley-Bixler, which are described in greater detail on Table 23-14. Fetuses with syndromic craniosynostosis are also at higher risk for potential airway challenges related to midface hypoplasia and/or complex anomalies of the airways, hearing loss, increased intracranial pressure, impaired feeding, and incomplete eyelid coverage.92 Craniosynostosis is suspected in utero by first noticing an abnormal head shape, and then by meticulous examination of each suture. Three-dimensional US with maximum intensity projection rendering can provide exquisite images of the skull bones and sutures under ideal conditions and is an important tool to diagnose craniosynostosis in utero.96 Fetal MRI complements fetal ultrasonography by revealing concomitant facial and brain anomalies, particularly when ultrasound images are suboptimal due to maternal body habitus, oligohydramnios, and/or unfavorable fetal head position.91
Syndrome | Gene | Inheritance/Incidence | Affected Sutures | Features |
---|---|---|---|---|
Apert | FGFR2 | AD/1:65,000 | Bicoronal Diastatic sagittal suture | Midface hypoplasia Hypertelorism Fusion of cervical vertebrae Foreshortened anterior cranial fossa Cleft palate/glossoptosis Agenesis of the corpus callosum Agenesis of the ductus venosus Abnormally expanded lungs Symmetrical syndactyly of the hands and feet |
Crouzon | FGFR2 FGFR3 | AD/1:60,000-64,500 | Coronal, sagittal and/or lambdoid | Midface hypoplasia Hypertelorism Fusion of cervical vertebrae Exophthalmos Beaked nose Mandibular prognathism Anal atresia Normal limbs |
Pfeiffer | FGFR FGFR2 | AD/1:100,000 | Coronal and/or sagittal Kleeblattschädel (cloverleaf skull) | Midface hypoplasia Hypertelorism Fusion of cervical vertebrae Syndactyly Brachydactyly Broad radially deviated thumbs Broad great toes Type 1 (FGFR 1 or 2): milder Type 2 (FGFR 2): cloverleaf skull and elbow fusion Type 3 (FGFR 2): no cloverleaf skull, visceral anomalies present |
Muenke (kinky hair) | FGFR3 | AD/1:10,000-12,000 | Uni- or Bicoronal synostosis | Midface hypoplasia Hypertelorism Macrocephaly Thrimble-shaped middle phalanges Carpal/tarsal fusion Wormian bones |
Saethre-Chotzen | TWIST1 FGFR2 | AD/1:25,000-50,000 | Uni- or Bicoronal synostosis | Parietal foramina Hypertelorism Syndactyly (index and middle fingers) Heart defects |
Antley-Bixler | FGFR2 | AR/Rare | Coronal and/or lambdoid | Midface hypoplasia Choanal atresia Joint contractures Radiohumeral (elbow) synostosis Femoral bowing Genitourinary anomalies |
Crouzonoid with acanthosis nigricans | FGFR-3 | AD/1:1,000,000 | Coronal, sagittal, and/or lambdoid | Crouzon facies Skeletal findings similar to achondroplasia Early widespread acanthosis nigricans (postnatal finding) Morse severe skull base abnormalities (postnatal finding) |
Craniofrontonasal syndrome | EFNB-1 | AD/Rare | Coronal synostosis (variable) | Hypertelorism Bifid nasal tip Longitudinally split nails (postnatal finding) |
Baller-Gerold syndrome | RECQL4 | AR/Rare | Craniosynostosis | Radial ray hypoplasia/aplasia Imperforate anus |
Beare-Stevenson syndrome | FGFR2 | AD/Rare | Craniosynostosis Kleeblattschädel (cloverleaf skull) | Cutis gyrate Anterior anus Genitourinary anomalies Developmental delay/early death |
Carpenter syndrome | RAB23 MEGF8 | AR/Rare | Coronal, sagittal, and/or lambdoid (variable) | Agenesis of the corpus callosum Congenital heart disease Abdominal wall defect Micrognathia Ascites Hydronephrosis Hypogenitalism Polysyndactyly hands and feet Brachydactyly Bowed femora or tibiae |
Jackson-Weiss | FGFR2 | AD/Rare | Craniosynostosis | Midface hypoplasia Broad great toes/normal hand Tarsal/metatarsal coalition |
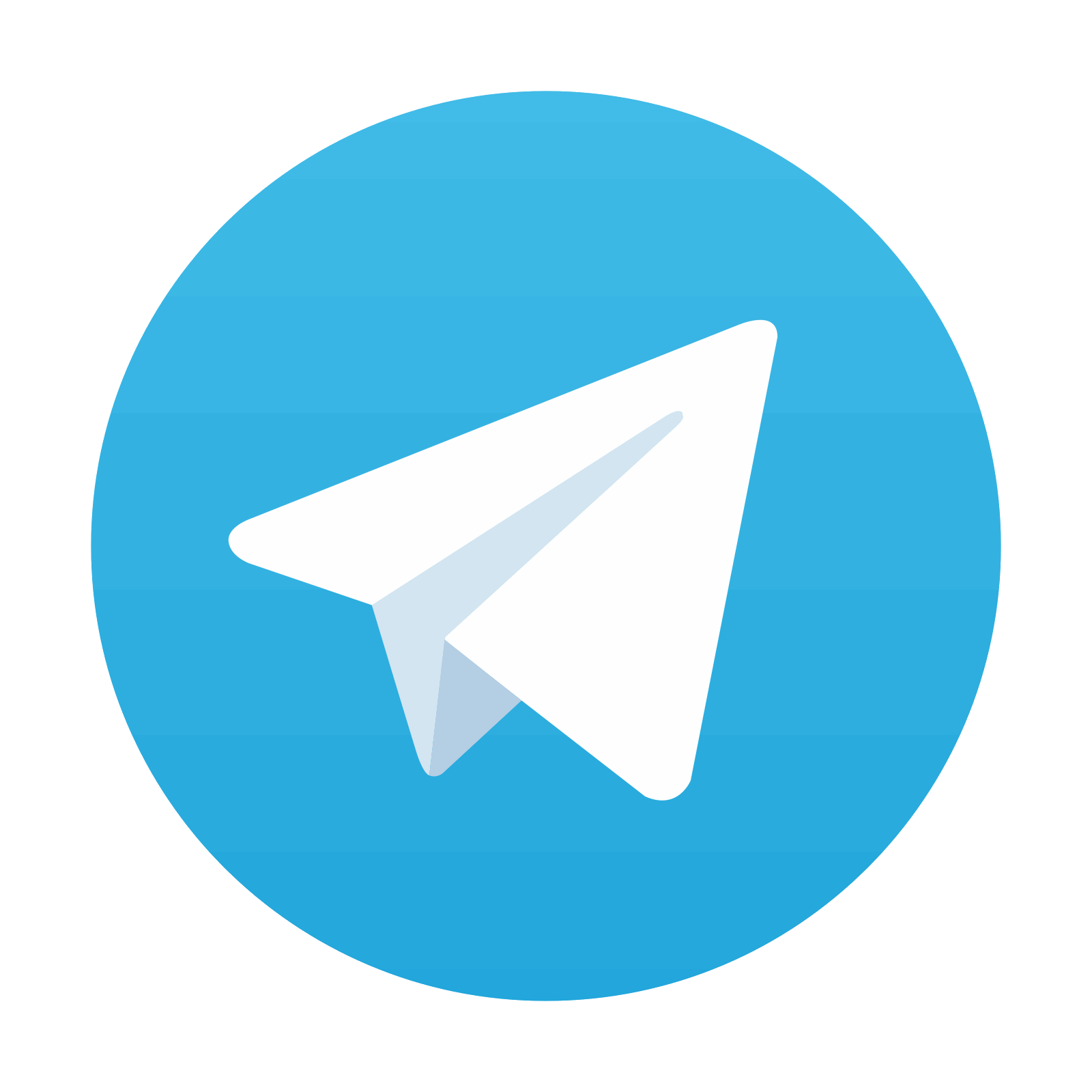
Stay updated, free articles. Join our Telegram channel

Full access? Get Clinical Tree
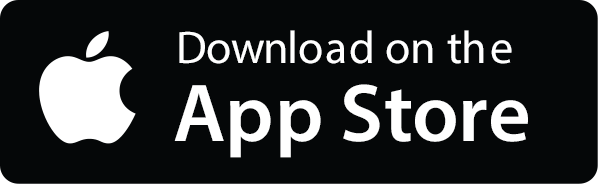
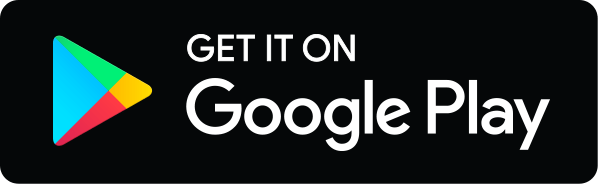
