Pre-implantation genetic diagnosis provides prenatal genetic diagnosis before implantation, thus allowing detection of chromosomal abnormalities and their exclusion from embryo transfer in assisted reproductive technologies. Polar body, blastomere or trophectoderm can each be used to obtain requisite genetic or embryonic DNA. Pre-implantation genetic diagnosis for excluding unbalanced translocations is well accepted, and pre-implantation genetic diagnosis aneuploidy testing to avoid repeated pregnancy losses in couples having recurrent aneuploidy is efficacious in reducing miscarriages. Controversy remains about whether pre-implantation genetic diagnosis aneuploidy testing improves take home pregnancy rates, for which reason adherence to specific indications is recommended while the issue is being adjudicated. Current recommendations are for obligatory 24 chromosome testing, most readily using array comparative genome hybridisation.
Preimplantation genetic diagnosis: indications and practicalities
Now over 20 years old, pre-implantation genetic diagnosis (PGD) is well established as an integral component of the prenatal genetic diagnosis armamentarium, a prime example of the translational fruits of the genome era. Complexities and safeguards, however, are necessary to assure accuracy. Although costs are higher than with traditional prenatal genetic diagnosis, PGD is sometimes the only practical way to achieve a goal. This applies, in particular, to improving pregnancy rates in assisted reproductive technologies (ART). Most pregnancy loses are caused by chromosomal abnormalities; thus, detecting and excluding these disorders by PGD becomes applicable to couples of known infertility or subfertility. The latter may be experiencing unrecognised embryonic losses, and be labelled clinically as infertile.
In this chapter, I first review approaches and pitfalls for obtaining gamete or embryonic DNA necessary for PGD. I then consider well-accepted and still arguable indications for applying PGD for subfertility, their diagnostic accuracy, and recent trends in diagnostic approaches for aneuploidy detection.
Obtaining cells for pre-implantation genetic diagnosis
Pre-implantation genetic diagnosis requires access to DNA from gametes or embryos before 6 days of conception, when implantation occurs. The three approaches are (1) polar-body biopsy assessing female gametes; (2) blastomere biopsy (aspiration), assessing the 3-day, six-to eight-cell cleaving embryo; and (3) trophectoderm biopsy, assessing the 5- to 6-day blastocyst.
Blastomere biopsy in the cleavage stage embryo (six to eight cells)
In blastomere biopsy, the glycoprotein layer surrounding the embryo, zona pellucida is traversed by mechanical, laser, or chemical means in order to extract a cell(s). The first two methods are most commonly used. Almost all centres remove only one cell because even one cell less is believed to reduce embryo survival as manifested by a 10% reduction in pregnancy rate; removal of two cells reduces the pregnancy rate considerably more. These figures are derived from data correlating pregnancy rates with numbers of blastomeres remaining after the thaw of cryopreserved embryos. Extrapolation of these pregnancy rates to those of biopsied embryos not subjected to cryopreservation may or may not be completely applicable. The 40–50% reduction in pregnancy rates associated with loss of two blastomeres, however, cast aspersions on protocols involving removal of two blastomeres. An experienced centre in Brussels, which once routinely removed two cells, recently reached similar conclusions; their live birth rates were 37.4% and 22.4% after removal of one versus two cells, respectively. The ‘take home’ baby rates are comparable with non-biopsied embryos. A total of 2005 cycles were monitored in the European Society of Human Reproduction and Embryology (ESHRE) PGD Consortium data collection; delivery rates were 16% per oocyte retrieval and 22% per embryo transfer; clinical pregnancy rates were 19 and 26%, respectively.
Polar-body biopsy
Oocyte genotype can be deduced readily by analysis of the first and second polar biopsy. The underlying principle is that the first polar body from a heterozygous individual showing a mutant maternal allele should be complemented by a primary oocyte having the normal allele. Oocytes deduced to be genetically normal can be allowed to fertilise in vitro and be transferred for potential implantation. Conversely, a normal polar body indicates an abnormal oocyte; thus, fertilisation would not proceed. If the assessment is for aneuploidy, a polar body with 24 or 22 chromosomes would indicate the oocyte scheduled for fertilisation would have 22 or 24 chromosomes, respectively; thus, the embryo would be monosomic or trisomic and not suitable for fertilisation. The same principle would apply with analysis of the second polar body.
Polar-body biopsy has advantages and disadvantages. One advantage is that first polar body is present before fertilisation; thus, its analysis offers the unique possibility of preconceptional diagnosis. The second polar body is not extruded until the mature oocyte is exposed to sperm. This becomes the only useful option if one must limit the number of oocytes that can be fertilised or embryos transferred; biopsy of the first polar body allows, in the absence of recombination, identification of a normal oocyte. Even without examining the second polar body, however, most euploid oocytes may be fertilised and reasonable pregnancy rates maintained, despite restrictive legislation. Another advantage of polar-body biopsy is that no reduction occurs in cell number. The obvious disadvantage is inability to assess paternal genotype, obviously precluding application if the father has an autosomal dominant disorder and making analysis less efficient in managing couples at risk for autosomal traits. On the other hand, 95% of aneuploidies arise in maternal meiosis, so there is little loss in efficiency in PGD aneuploidy testing.
If one is carrying out PGD for detecting single nucleotide polymorphisms (SNPs) on mutant alleles causing single-gene disorders, one must take into account recombination, a phenomenon that obligatorily occurs between homologous chromosomes. If recombination of chromatids were not to occur, the two chromatids of a single chromosome in the first polar body would be identical in genotype and complementary to the oocyte; the second polar body (chromatid) would be identical to the oocyte. If crossing over were, however, to involve the region containing the gene in question, the single chromosome in the first polar body would show different alleles on its two chromatids (heterozygosity). Genotype of the oocyte could thus not be predicted without either biopsy of the second polar body or biopsy of the embryo per se (blastomere). In practice, both first and second polar bodies are biopsied in almost all centres.
Polar-body biopsy has recently gained popularity for an unexpected reason: greater validity in correlating results of a single-cell analysis. It is paradoxical but true that chromosomal status of the oocyte, only deduced from its complementary first and second polar bodies, is a more reliable indicator of embryo status than a blastomere from the embryo itself. Sequential testing of blastomere or blastocyst would still be necessary to exclude the rare 5% of paternally derived aneuploidies. That analysis of a blastomere is less accurate than polar-body biopsy reflects the occurrence in blastomeres of mitotic non-disjunctional events that are unrepresentative of the status of the embryo’s remaining cells. A randomised-controlled trial (RCT) planned by ESHRE to assess whether PGD aneuploidy testing increases ART success rates will use polar-body biopsy.
Centres technically experienced in polar-body biopsy seem to have pregnancy rates comparable to those achieved using blastomere biopsy. No attempts have been made to compare relative safety of blastomere versus polar-body biopsy, reflecting, until recently, the reality that few centres carried out polar-body biopsy. In fact, RCTs could be potentially misleading if technical expertise in a given centre is not comparable with both techniques.
Blastocyst biopsy
Biopsy of the trophectoderm in the 5- to 6 day, 120-cell blastocyst is the third approach. More cells can be removed at this stage, potentially facilitating diagnosis. Because trophectoderm forms the placenta, embryonic cells per se could theoretically not be removed. In fact, long before the development of PGD, human blastocysts were recovered by uterine lavage. Lavage to recover blastocysts was envisioned to be the approach by which cells removed by trophectoderm biopsy could allow genetic diagnosis. After the development of the polymerase chain reaction, lavage for PGD was no longer pursued. Blastocyst biopsy received little attention, as ART centres routinely generated salutary pregnancy rates by transferring 3- to 4-day morulae.
Practitioners of ART have now returned to the blastocyst as a preferred stage of transfer. The additional 2–3 days in culture, beyond that required for an eight-cell embryo, allows some self-selection against non-thriving and aneuploid embryos. About one-third of embryos with chromosomal abnormalities are selected against between 3 and 5. Pre-implantation genetic diagnosis is still necessary to exclude remaining aneuploidies, whose frequency depends upon maternal age. Australian and other investigators have demonstrated the feasibility of blastocyst biopsy for PGD. One problem is increased rate of monozygotic twinning and concern that some morphologically good 4–5 days embryos may not thrive on in-vitro media constructed for use up to 3 days. Another potential problem is that culture media suitable for day 1-3 embryos may not always be optimal for day 4–5 embryos.
Embryo biopsy and cryopreservation
Embryo survival after polar body or blastomere biopsy was once considered almost impossible. It was thus believed that biopsied embryos must be transferred by day 6, with all required diagnostic results available by then. Cryopreservation of biopsied embryos is now feasible. As a result, one promising approach can be envisioned: blastocyst biopsy, vitrification, unhurried diagnostic analysis, thawing 1 month or more later and transfer in synchrony with a natural cycle. The intervening month allows diagnostic application, like array comparative genome hybridisation (CGH) or multiplex genome analysis using polymorphisms (SNPs) or copy number variants (CNVs). Although all these can be carried out in ‘real time’, allowing fresh embryo transfer, this is a technical tour de force and now unnecessary.
Obtaining cells for pre-implantation genetic diagnosis
Pre-implantation genetic diagnosis requires access to DNA from gametes or embryos before 6 days of conception, when implantation occurs. The three approaches are (1) polar-body biopsy assessing female gametes; (2) blastomere biopsy (aspiration), assessing the 3-day, six-to eight-cell cleaving embryo; and (3) trophectoderm biopsy, assessing the 5- to 6-day blastocyst.
Blastomere biopsy in the cleavage stage embryo (six to eight cells)
In blastomere biopsy, the glycoprotein layer surrounding the embryo, zona pellucida is traversed by mechanical, laser, or chemical means in order to extract a cell(s). The first two methods are most commonly used. Almost all centres remove only one cell because even one cell less is believed to reduce embryo survival as manifested by a 10% reduction in pregnancy rate; removal of two cells reduces the pregnancy rate considerably more. These figures are derived from data correlating pregnancy rates with numbers of blastomeres remaining after the thaw of cryopreserved embryos. Extrapolation of these pregnancy rates to those of biopsied embryos not subjected to cryopreservation may or may not be completely applicable. The 40–50% reduction in pregnancy rates associated with loss of two blastomeres, however, cast aspersions on protocols involving removal of two blastomeres. An experienced centre in Brussels, which once routinely removed two cells, recently reached similar conclusions; their live birth rates were 37.4% and 22.4% after removal of one versus two cells, respectively. The ‘take home’ baby rates are comparable with non-biopsied embryos. A total of 2005 cycles were monitored in the European Society of Human Reproduction and Embryology (ESHRE) PGD Consortium data collection; delivery rates were 16% per oocyte retrieval and 22% per embryo transfer; clinical pregnancy rates were 19 and 26%, respectively.
Polar-body biopsy
Oocyte genotype can be deduced readily by analysis of the first and second polar biopsy. The underlying principle is that the first polar body from a heterozygous individual showing a mutant maternal allele should be complemented by a primary oocyte having the normal allele. Oocytes deduced to be genetically normal can be allowed to fertilise in vitro and be transferred for potential implantation. Conversely, a normal polar body indicates an abnormal oocyte; thus, fertilisation would not proceed. If the assessment is for aneuploidy, a polar body with 24 or 22 chromosomes would indicate the oocyte scheduled for fertilisation would have 22 or 24 chromosomes, respectively; thus, the embryo would be monosomic or trisomic and not suitable for fertilisation. The same principle would apply with analysis of the second polar body.
Polar-body biopsy has advantages and disadvantages. One advantage is that first polar body is present before fertilisation; thus, its analysis offers the unique possibility of preconceptional diagnosis. The second polar body is not extruded until the mature oocyte is exposed to sperm. This becomes the only useful option if one must limit the number of oocytes that can be fertilised or embryos transferred; biopsy of the first polar body allows, in the absence of recombination, identification of a normal oocyte. Even without examining the second polar body, however, most euploid oocytes may be fertilised and reasonable pregnancy rates maintained, despite restrictive legislation. Another advantage of polar-body biopsy is that no reduction occurs in cell number. The obvious disadvantage is inability to assess paternal genotype, obviously precluding application if the father has an autosomal dominant disorder and making analysis less efficient in managing couples at risk for autosomal traits. On the other hand, 95% of aneuploidies arise in maternal meiosis, so there is little loss in efficiency in PGD aneuploidy testing.
If one is carrying out PGD for detecting single nucleotide polymorphisms (SNPs) on mutant alleles causing single-gene disorders, one must take into account recombination, a phenomenon that obligatorily occurs between homologous chromosomes. If recombination of chromatids were not to occur, the two chromatids of a single chromosome in the first polar body would be identical in genotype and complementary to the oocyte; the second polar body (chromatid) would be identical to the oocyte. If crossing over were, however, to involve the region containing the gene in question, the single chromosome in the first polar body would show different alleles on its two chromatids (heterozygosity). Genotype of the oocyte could thus not be predicted without either biopsy of the second polar body or biopsy of the embryo per se (blastomere). In practice, both first and second polar bodies are biopsied in almost all centres.
Polar-body biopsy has recently gained popularity for an unexpected reason: greater validity in correlating results of a single-cell analysis. It is paradoxical but true that chromosomal status of the oocyte, only deduced from its complementary first and second polar bodies, is a more reliable indicator of embryo status than a blastomere from the embryo itself. Sequential testing of blastomere or blastocyst would still be necessary to exclude the rare 5% of paternally derived aneuploidies. That analysis of a blastomere is less accurate than polar-body biopsy reflects the occurrence in blastomeres of mitotic non-disjunctional events that are unrepresentative of the status of the embryo’s remaining cells. A randomised-controlled trial (RCT) planned by ESHRE to assess whether PGD aneuploidy testing increases ART success rates will use polar-body biopsy.
Centres technically experienced in polar-body biopsy seem to have pregnancy rates comparable to those achieved using blastomere biopsy. No attempts have been made to compare relative safety of blastomere versus polar-body biopsy, reflecting, until recently, the reality that few centres carried out polar-body biopsy. In fact, RCTs could be potentially misleading if technical expertise in a given centre is not comparable with both techniques.
Blastocyst biopsy
Biopsy of the trophectoderm in the 5- to 6 day, 120-cell blastocyst is the third approach. More cells can be removed at this stage, potentially facilitating diagnosis. Because trophectoderm forms the placenta, embryonic cells per se could theoretically not be removed. In fact, long before the development of PGD, human blastocysts were recovered by uterine lavage. Lavage to recover blastocysts was envisioned to be the approach by which cells removed by trophectoderm biopsy could allow genetic diagnosis. After the development of the polymerase chain reaction, lavage for PGD was no longer pursued. Blastocyst biopsy received little attention, as ART centres routinely generated salutary pregnancy rates by transferring 3- to 4-day morulae.
Practitioners of ART have now returned to the blastocyst as a preferred stage of transfer. The additional 2–3 days in culture, beyond that required for an eight-cell embryo, allows some self-selection against non-thriving and aneuploid embryos. About one-third of embryos with chromosomal abnormalities are selected against between 3 and 5. Pre-implantation genetic diagnosis is still necessary to exclude remaining aneuploidies, whose frequency depends upon maternal age. Australian and other investigators have demonstrated the feasibility of blastocyst biopsy for PGD. One problem is increased rate of monozygotic twinning and concern that some morphologically good 4–5 days embryos may not thrive on in-vitro media constructed for use up to 3 days. Another potential problem is that culture media suitable for day 1-3 embryos may not always be optimal for day 4–5 embryos.
Embryo biopsy and cryopreservation
Embryo survival after polar body or blastomere biopsy was once considered almost impossible. It was thus believed that biopsied embryos must be transferred by day 6, with all required diagnostic results available by then. Cryopreservation of biopsied embryos is now feasible. As a result, one promising approach can be envisioned: blastocyst biopsy, vitrification, unhurried diagnostic analysis, thawing 1 month or more later and transfer in synchrony with a natural cycle. The intervening month allows diagnostic application, like array comparative genome hybridisation (CGH) or multiplex genome analysis using polymorphisms (SNPs) or copy number variants (CNVs). Although all these can be carried out in ‘real time’, allowing fresh embryo transfer, this is a technical tour de force and now unnecessary.
Diagnostic methods for selecting chromosomal abnormalities
Because a karyotype cannot be obtained reliably from a single cell, chromosome-specific fluorescent in-situ hybridisation (FISH) probes have traditionally been used. Pivotal to obtaining accurate results is an intact nucleus. A high percentage of informative cases can be achieved only if removal of a blastomere or polar body can be accomplished in 3–5 mins, preferably less. Otherwise, the embryo (and blastomere or polar body) may be damaged as result of desiccation, temperature changes or alterations in osmolarity. The high error rates and high numbers of non-informative embryos in certain RCTs may reflect embryo damage.
Diagnostic sensitivity with chromosome-specific probes logically should increase as the number of chromosomes tested increases. About 70–80% of aneuploidy embryos can be detected using eight to12 chromosomes (e.g. X, Y, 13, 15, 16, 17, 18, 21, 22). These chromosomes must be interrogated over two to three hybridisation cycles, given that no more than five probes per hybridisation are recommended. Munné et al. observed that actually 90% of aneuploidy can be detected by interrogating only 10 to 12 chromosomes. The reason is that rarer trisomies (double trisomy) often accompany more traditional aneuploidies (e.g. numbers 16 or 21). Thus, screening with only 10 chromosome-specific probes identifies 89% (382 out of 427) of embryos that are either normal or abnormal; screening with 12 probes identifies 91% (389 out of 427).
Notwithstanding excellent results with chromosome-specific FISH probes over the past 10–15 years, the current standard is to obtain information on all 24 chromosomes. In fact, hybridisation can be carried out to cover all chromosomes. For example, using single blood cells enumerated all 24 chromosomes on a single slide, carrying out six successive FISH cycles each with four chromosomes. Griffin et al. developed a 24-colour FISH assay, using four rounds of hybridisation completed within 24 h. The preferred approach, however, is to apply genome-wide molecular approaches (cytogenomics). One approach is to use SNPs, using several informative per chromosome. Given DNA from male and female partners and other family member(s), one can deduce the number of chromosomes in a single cell on the basis of number of SNPs (alleles) on a given chromosome. For example, one normally expects two different SNPs, or a 1:1 allele ratio. If there are three different SNPs or an altered ratio (2:1), trisomy can be deduced. Single-cell aneuploidy testing using genome-wide SNPs can be accomplished reliably on a single blastomere. Results can be provided quickly enough for normal embryos to be transferred in the same cycle. Using SNP analysis, accomplished this in 4 h.
Comparative genomic hybridisation (chromosomal microarrays)
Comparative genomic hybridisation is an exciting molecular cytogenetic technique that allows comprehensive analysis of the entire genome, based on single-stranded DNA annealing (hybridising) with a complementary single-stranded DNA. Hybridisation occurs whether denatured DNA is from the same individual or from different individuals. Typically, normal (control) DNA is labelled with a fluorochrome of one colour (e.g. green), whereas test (patient) DNA is labelled with a fluorochrome of a different colour (e.g. red). Given that both test and control DNA are denatured (single stranded), hybridisation occurs. If equal amounts of control and test DNA are present, the colour of the hybridised mixture should be yellow. If the test DNA is in excess (e.g. trisomy), the entire region for that chromosome would reveal more of the colour used to connote test (patient) DNA. This would be red in the previous example.
In practice, small amounts of single-stranded DNA of known sequence are placed by photolithography onto a platform (array) in ordered fashion. The amount of DNA in each ‘spot’ is small (i.e. micro). The number of sequences is set in advance, but is expected to encompass the entire genome, one sequence overlaying the adjacent one (‘tiling’). The ‘control’ DNA embedded by photolithography is labelled with a fluorochrome of one colour, and is exposed to single-stranded test DNA (e.g. patient), now labelled with a fluorochrome of different colour as reasoned above. Again, if control and test DNA are quantitatively equal for a given sequence, the colour is yellow. If test DNA is in excess (trisomy), one colour predominates; if test DNA is deficient, the other colour predominates.
Various commercial platforms are available, all interrogating sequences of DNA along every chromosome. ‘Coverage’ varies slightly based on sensitivity sought, but in all there is redundancy. That is, a given region is interrogated more than once to ensure replicability before making a diagnosis. Arrays used to interrogate blood from liveborn infants or chorionic villi or fluid cells are designed to be more sensitive than those used for PGD. The latter are designed to detect aneuploidies reliably only from all chromosomes.
Wells et al. used array CGH (Illumina platform) and found the probability of an individual interrogated by array CGH embryo generating a pregnancy was 66.7% compared with 27.9% without PGD. Sher et al. reported a remarkable increase in birth rates transferring blastocysts which, as 3-day embryos, underwent biopsy and CGH followed by vitrification and then thawing for transfer at a later time: 48% birth rate (45 out of 94) per transferred CGH-tested blastocyst compared with only 15% (57 out of 382) in non-CGH tested blastocysts. In 2012, array CGH is the preferred diagnostic approach to assess 24 chromosome aneuploidy. Of note, the platform detects both whole chromosome and chromatid errors, the latter of which now seems to be the more common cytologic explanation for aneuploidy.
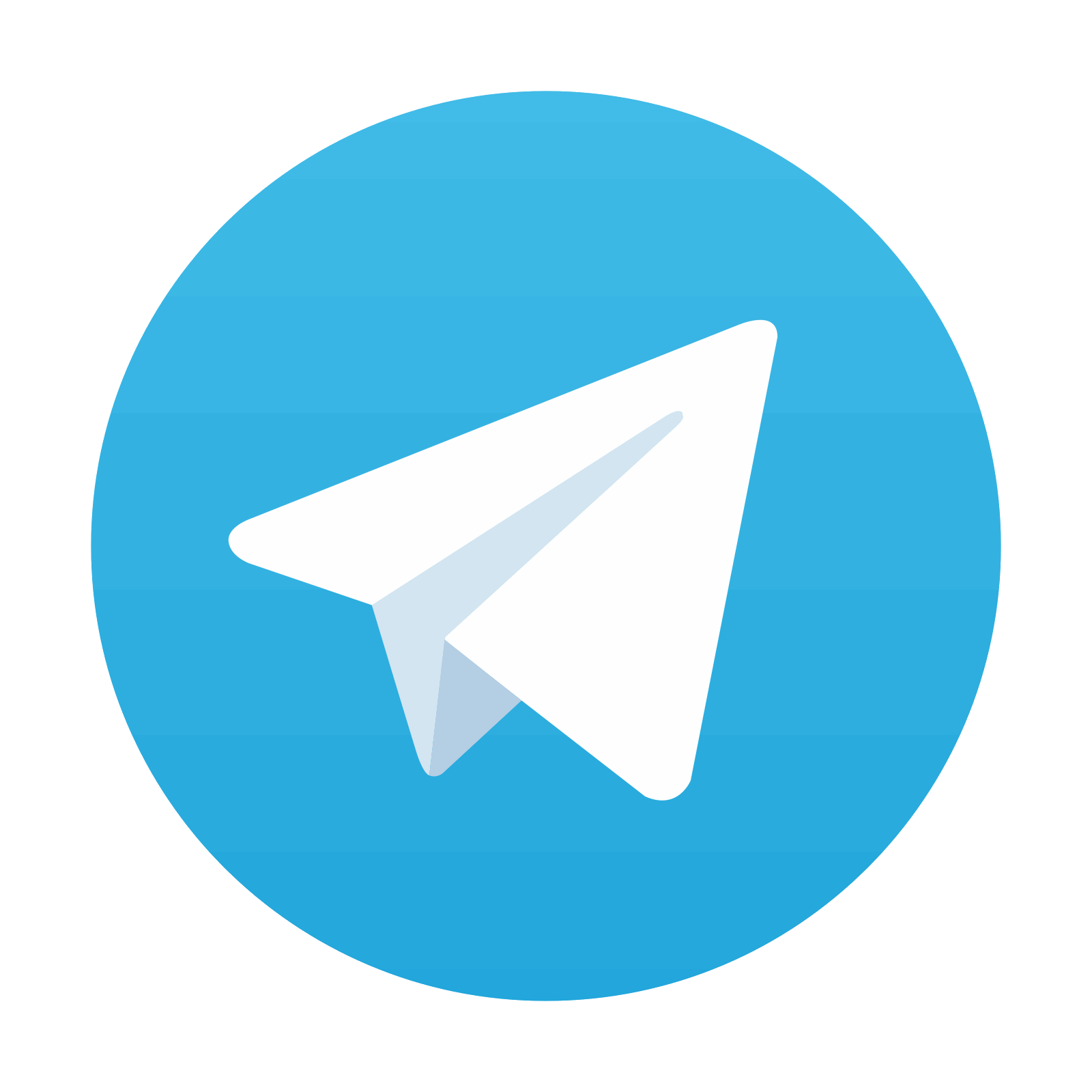
Stay updated, free articles. Join our Telegram channel

Full access? Get Clinical Tree
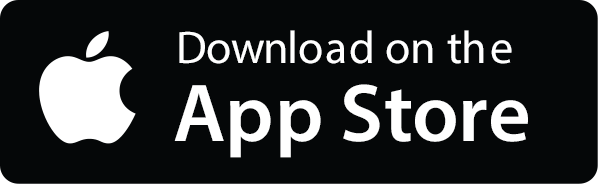
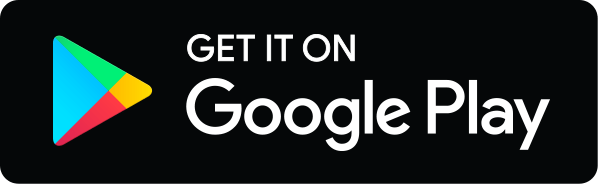