Objective
The objective of the study was to investigate whether precipitated egg white could be used as a sealant for iatrogenic injury to fetal membranes.
Study Design
Membranes were collected, washed, and affixed to the bottom of glass cylinders filled with second-trimester amniotic fluid. An iatrogenic defect with an 8-gauge needle was created in the test column, and precipitated egg white was added. Fluid levels in test and control columns were compared over a 14 day period. The amount of fluid added to columns was compared using the Wilcoxon rank sum test; P < .05 was considered significant.
Results
Test columns (20/21) maintained seal integrity for 14 days and required less amniotic fluid to be added as compared with controls: median: 55.1 mL (range, 20–137 mL) vs 61.6 mL (range, 22–159 mL), respectively ( P < .01).
Conclusion
Precipitated egg white has the potential to seal iatrogenic membrane defects for up to 14 days’ duration.
Fetal membranes are composed of 2 layers, the amnion and the chorion. The tensile strength of these membranes has been attributed primarily to the amnion. Preterm premature rupture of membranes (PPROM) has been classified as iatrogenic or spontaneous. Both subtypes have their own characteristics. Iatrogenic PPROM (iPPROM) usually consists of a defect with sharply delineated borders; the defect is located at the site of the procedure and it may be associated with spontaneous healing. In contrast, spontaneous rupture consists of a defect with poorly delineated margins, is usually located near the cervix, and spontaneous resealing is infrequently seen.
Rupture of the amniotic membranes is a complication encountered in 1-2% of all diagnostic amniocenteses. This relative low incidence is proposed to be due to the use of small diameter needles (22-gauge). Recently a fetal intervention using a 10- or 12-F trocar for fetoscopy in the treatment of severe twin-twin transfusion has come into widespread use. Unfortunately, the use of a large caliber trocar can lead to the complication of iPPROM in as many as 30% of the cases. Thus, iPPROM has been labeled as the Achilles heel of invasive fetal therapy. A solution to this problem will be necessary if fetoscopic therapy is to be applied to other fetal conditions for their treatment.
Multiple strategies have been studied for the prevention of iPPROM after invasive fetal procedures. Most have had disappointing results. They include amniopatch, fibrin glue, collagen-based graft, Synthaseal, Gelfoam, laser welding, Tisseel, suture, collagen plug, and bioactive amniotic membrane scaffolds.
Previous in vitro studies at our institution have demonstrated the capacity of precipitated egg white in resealing artificially produced defects in balloons and latex condoms containing water.
The purpose of the current study was to examine the ability of egg white to create a stable seal in a model for iatrogenic puncture of human fetal membranes.
Materials and Methods
Second- and third-trimester membranes from patients with negative testing for human immunodeficiency virus or hepatitis B and C and with no clinical evidence of chorioamnionitis, abruption, or prolonged rupture of membranes were collected. Membranes were harvested from elective cesarean sections and elective second-trimester terminations performed by dilation and evacuation at an outpatient clinic.
These procedures and consent forms were approved by the institutional review board under protocol H-21579. All samples were deidentified.
Once amniotic membranes were collected, they were washed with normal saline solution to remove all blood and debris. Membranes were then transported to our laboratory and stored in phosphate-buffered saline solution (PBS). PBS was chosen as storage media in an effort to maintain viability in 70% of amniotic cells without allowing collagen degradation. Membranes were then stored at 4°C. Storage never exceeded 12 hours in an effort to maintain cell viability.
Second-trimester amniotic fluid was obtained from therapeutic amnioreductions in patients undergoing placental laser ablation for twin-to-twin transfusion syndrome (Baylor College of Medicine institutional review board protocol H-20840). Fluid was stored at –70°C until it was thawed for use.
Egg white was precipitated using the method of serial acidification, precipitation, centrifugation, and alkali resuspension described by Berry. Normal jumbo chicken eggs from the common domestic chicken ( Gallus domesticus ) were cracked on a sterile white surface with special care in leaving the yolk intact. Thin and thick egg white components were identified; and using a 10 mL syringe, the thick egg white was aspirated and placed in a glass container. The remaining egg white and the yolk were discarded. The thick egg white was then acidified using 25 mL of 5% acetic acid (white vinegar) and centrifuged for 1 minute. Acetic acid was then decanted.
The pellet was then resuspended with 25 mL of 5% acetic acid and again centrifuged for 1 minute. Again the acetic acid was decanted. The resulting pellet was then suspended in 25 mL of normal saline solution and centrifuged for 1 minute. The normal saline was then decanted and the pellet was suspended in dilute sodium bicarbonate. Once an optimal pH of 7.4 was achieved, the precipitate was refrigerated and stored at 4°C. One jumbo egg produced approximately 5-7 mL of precipitate.
To study human membrane integrity, we utilized an in vitro model previously reported by Reddy et al and Louis-Sylvestre et al. All materials that were used in the final phase of the experiment were autoclaved using a liquid-based mode at 90°C to eliminate all potential biological contaminants. The membranes were then placed on a sterile glass surface and a 7 × 7 cm square was trimmed. They were then stretched over the bottom of a sterile 3 cm diameter graduated cylinder that had been modified to be open at both ends. Membranes were affixed to the lower end of the cylinder using 2 sterile rubber bands with the amnion facing the inside of the glass column and the chorion facing out ( Figure 1 , A).

Two glass cylinders were prepared using the membrane from each patient. One was randomly assigned to be the test column and the other served as the control. Both columns were then filled with second-trimester amniotic fluid that had been previously thawed and warmed to 37°C in a water bath. A predetermined height of 8 cm of fluid was used when a second-trimester membrane was being studied; a 12 cm height was used for third-trimester membranes. These conditions were chosen to mimic ambient intraamniotic fluid pressures encountered in normal pregnancy.
After confirmation of lack of leakage from both cylinders, the fetal membrane attached to the test column was punctured at a 90° angle with a sterile 8-gauge needle creating approximately a defect 3.3 mm in diameter. This gauge needle was chosen to simulate the defect created by the 3.3 mm (10F) trocar typically used for fetoscopic laser surgery. The column was then placed in a vertical position and fluid was allowed to drain for 30 seconds. Five milliliters of precipitated egg white was then added to the top of the test column to form a seal in the membrane defect.
Additional amniotic fluid was then added to the column to achieve the predetermined fluid height of the column. If leakage persisted, another 5 mL of precipitated egg white were added to the column, and it was again filled with amniotic fluid to the prescribed height. If the leakage persisted, the experiment was labeled a failure.
The fixed columns were then suspended by clamps and submerged in sterile glass beakers filled with sterile PBS, which were filled to a level that would allow the bottom 2 cm of the column to be submerged in fluid. Two additional centimeters of fluid column height were added to counteract the loss of hydrostatic pressure from the buoyancy. The glass beakers were then placed in a circulating water bath preset to maintain amniotic fluid in the column at 37°C ( Figure 1 , B). An antibiotic was added to both the amniotic fluid in the column and the PBS in the beaker to prevent bacterial overgrowth (see Results section in the following text for description of the specific antimicrobials). Both water bath and glass columns were covered to avoid contamination from the environment.
Levels of the amniotic fluid in the control and test columns were evaluated every 24 hours, and amniotic fluid was added as needed to maintain the prespecified fluid level to simulate physiological hydrostatic pressures throughout the experimental period, and the data were recorded. Membrane rupture was defined as a total loss of fluid level in the column. The experiment was continued for 14 days.
The test and control columns were compared for the duration of the seal and the total amount of fluid added daily. The Wilcoxon rank sum test was used to compare the median amount of fluid added to both paired columns. Statistical analysis was completed using SAS statistical software (version 9.2; SAS Institute, Inc, Cary, NC). P < .05 was considered statistically significant.
Results
Initially, 5 experiments using term fetal membranes were undertaken to establish the optimal conditions for the experiment. Initially, we proposed that a difference of 20% or more in fluid height between the test columns as compared with the control columns would be indicative of a membrane leak. We noted that the fluid levels in both columns decreased at variable rates because of evaporation and transmembranous passage of fluid. This prompted our decision to add additional amniotic fluid to the columns to maintain a prescribed hydrostatic pressure and to measure the difference between the 2 columns.
Our second experiment was complicated by early rupture. Microbiologic culture of the amniotic fluid in the column revealed bacterial overgrowth with Stenotrophomonas sp. Because this is a bacterium that is commonly found in water supplies, we undertook 2 modifications to the protocol. All distilled water and glassware used in the study were autoclaved. In addition, the antimicrobial combination initially used in the protocol (penicillin, 100 units/mL and streptomycin, 100 μg/mL) was altered to a new regimen (chloramphenicol, 30 μg/mL; tetracycline, 60 μg/mL; gentamicin, 30 μg/mL; and amphotericin, 30 μg/mL) to prevent the growth of the previously cultured pathogen.
The 3 remaining experiments required minor changes predominantly related to methods to seal the tops of the columns and the water bath. Our final proof-of-concept experiment resulted in membrane integrity for a total of 6 weeks without evidence of infection.
Twenty-eight potential test subjects were approached and asked to participate; 21 gave their written consent. Table 1 shows the demographic characteristics of the 21 participating patients.
Age, y | Gestational age, wks | Parity | Mode of Delivery | Notes |
---|---|---|---|---|
25 | 28.3 | G2P1102 | Cesarean section | Maternal schwannoma, dexamethasone for ↑ ICP |
27 | 17.5 | — | Elective abortion | Fetal anencephaly |
29 | 19.6 | — | Elective abortion | Fetal anencephaly |
28 | 38.6 | G3P3003 | Cesarean section | Maternal VSD |
32 | 37.4 | G4P3013 | Cesarean section | Elective |
31 | 38.4 | G2P2002 | Cesarean section | Elective |
28 | 38.6 | G3P2012 | Cesarean section | Elective |
26 | 38.4 | G3P3003 | Cesarean section | Elective |
35 | 39.1 | G6P4024 | Cesarean section | Elective |
37 | 36.4 | G2P1102 | Cesarean section | Elective |
21 | 34.2 | G1P1001 | Cesarean section | Elective |
26 | 28.3 | G4P2113 | Cesarean section | TTTS, s/p laser at 20 weeks’ gestation |
33 | 37 | G3P2012 | Cesarean section | Elective |
20 | 24.5 | G1P1001 | Cesarean section | Elective |
39 | 24.2 | G9P0272 | Cesarean section | CHF, chronic renal disease |
24 | 38.6 | G2P1102 | Cesarean section | Elective |
28 | 36.3 | G1P1001 | Cesarean section | Elective |
38 | 39.2 | G2P1012 | Cesarean section | Elective |
28 | 37.4 | G3P3003 | Cesarean section | Elective |
35 | 38.2 | G2P2002 | Cesarean section | Elective |
26 | 37.4 | G3P2012 | Cesarean section | Elective |
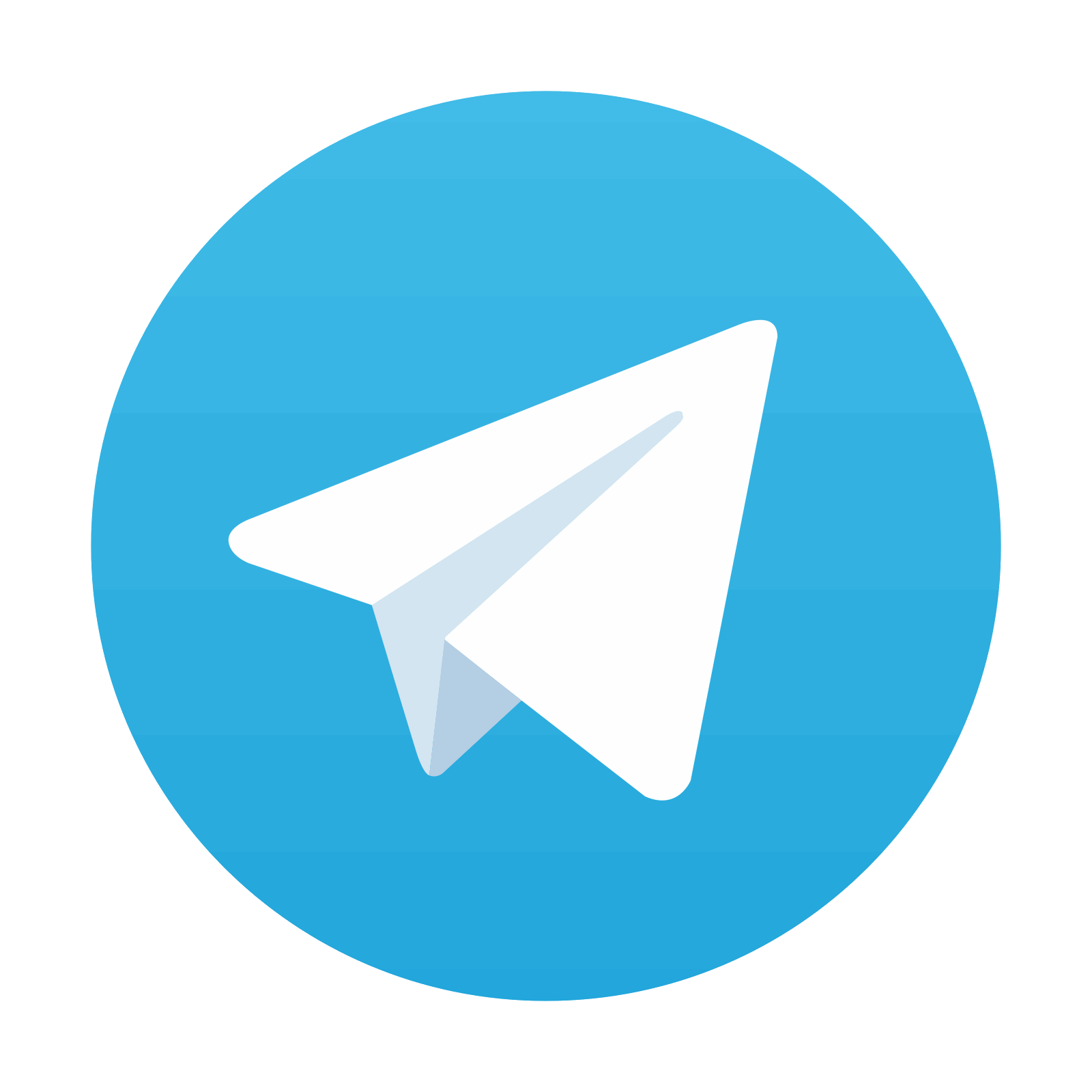
Stay updated, free articles. Join our Telegram channel

Full access? Get Clinical Tree
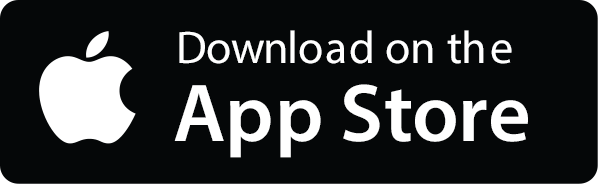
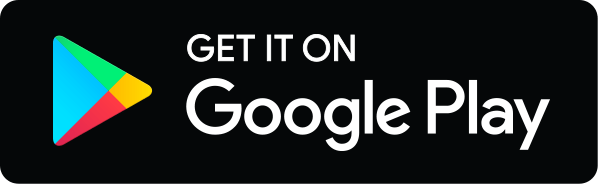
