Poisoning is a morbid state produced by the exposure to a toxic agent (poison) that causes a functional disturbance and/or structural damage. Although the majority of poisonings during pregnancy are accidental, up to one-fifth can be intentional as part of a suicide gesture or attempt.
Two general principles should be kept in mind when treating pregnant women who are poisoned:
More harm and damage may result from withholding or delaying needed therapy to the mother.
We treat the fetus by treating the mother.
Excluding drugs of abuse, the three most common intentional poisonings during pregnancy are those by acetaminophen (also known as paracetamol or acetyl-para-aminophenol [APAP]), nonsteroidal anti-inflammatory medications (NSAIDs; we will review salicylate poisoning), selective serotonin reuptake inhibitors (SSRIs) and opioids (typically in the form of analgesics).
This chapter specifically addresses the perinatal concerns and management of these three poisonings and that of iron, which because of its availability to pregnant women can also be implicated in an acute poisoning.
Pregnancy represents a special population due its physiologic changes affecting drug and toxin absorption, distribution, metabolism, excretion, and transplacental passage with potential fetal effects. General toxicologic and decontamination management principles are the same as for the nonpregnant population. Maternal stabilization is priority, with immediate evaluation of ventilatory, hemodynamic, and mental status. In patient with mental status changes, suspect hypoglycemia or narcotic overdose first and treat accordingly (dextrose and/or naloxone).
Excluding alcohol and drugs of abuse, APAP is the most common drug taken in overdose during pregnancy.
Most APAP is metabolized in the liver by being conjugated with sulfate or glucuronide to form nontoxic metabolites that are excreted in the urine (Fig. 23-1). Approximately 7% of APAP, however, is metabolized in the liver and kidneys by cytochrome P450 to form a toxic metabolite, N-acetyl-p-benzoquinoneimine (NAPQI). NAPQI is an extremely reactive molecule that covalently binds to macromolecules, leading to cell injury and death. NAPQI normally undergoes detoxification by combining with glutathione to form a nontoxic mercapturic acid metabolite that is excreted in the urine. With APAP overdose, however, so much NAPQI is formed that glutathione stores become depleted resulting in NAPQI-induced cytotoxicity. Acetaminophen poisoning principally affects the liver and, to a lesser extent, the kidneys.
Patients acutely ingesting more than 140 mg/kg of acetaminophen are at risk for hepatotoxicity. One can predict the risk for developing hepatotoxicity after an acute, single ingestion by obtaining a serum APAP concentration at least 4 hours after ingestion. Plotting the resulting level on a standard nomogram estimates the risk for hepatotoxicity (Fig. 23-2). If an antidote is not given within 8 hours and hepatotoxicity develops, it is associated with prolonged prothrombin time (PT) and elevation of transaminases (commonly into the thousands). Enzyme values usually peak between 72 and 96 hours after ingestion. Jaundice is uncommon. Seriously poisoned patients occasionally suffer from pancreatitis, oliguria, hypotension, myocardial ischemia, and, possibly, necrosis. Persons who take massive overdoses may present in the first few hours (before the onset of liver failure) with coma and severe metabolic acidosis with elevated lactate concentrations.
FIGURE 23-2
Acetaminophen nomogram. For use only after a single, acute ingestion in a patient who has not recently taken acetaminophen prior to ingestion. A level should not be plotted on the nomogram unless it was obtained at least 4 hours after ingestion. If a level falls above the lower line, N-acetylcysteine should be continued (if already started) or administered immediately.

While nausea and vomiting commonly develop early in APAP poisoning, patients who ingest fatal doses may suffer no symptoms until the onset of symptomatic liver failure, which can occur 1 to 4 days later. Therefore, a serum APAP value must be obtained from both symptomatic and asymptomatic patients in order to properly assess the severity of the overdose.
Patients who habitually take excessive doses of acetaminophen (usually well in excess of 4 g per day) are more likely to develop renal failure along with hepatotoxicity. Serum APAP concentrations in these patients cannot be correlated with severity of illness or risk of hepatotoxicity.
Acetaminophen can cross the placenta and has the potential for fetal hepatotoxicity and in acute intoxications of spontaneous abortion and stillbirth.
Supportive therapy including IV fluids, oxygen, cardiac monitoring, and electronic fetal monitoring (if appropriate for the gestational age of the pregnancy).
Decontamination procedures: Induced emesis may be indicated for home treatment of a large dose (>100 mg/kg). Gastric lavage and activated charcoal (AC) (1 g/kg in water) can prevent further absorption of acetaminophen. Oral AC avidly adsorbs acetaminophen and should be administered if the patient presents within 1 hour of ingestion. The network of pores present in AC adsorbs 100 to 1000 mg of drug per gram of charcoal.
In pregnancy, oral AC may be of benefit greater than 1 hour after the ingestion as the gastric emptying is delayed. Oral AC administered with NAC greater than 4 hours after ingestion has been shown in one case series to be effective in reducing the incidence of liver toxicity after toxic acetaminophen ingestion.
Antidote: The antidote for APAP poisoning is N-acetylcysteine (NAC, Mucomyst®) (Fig. 23-3). NAC undergoes conversion to cysteine, which, in turn, is metabolized to glutathione. NAC’s main effect is to maintain glutathione stores so that NAPQI can be detoxified. Started within 8 hours of ingestion, NAC prevents serious maternal hepatic and renal toxicity from APAP. To ensure that NAC is started promptly after an acute ingestion, it is generally most prudent to begin NAC therapy immediately and discontinue it only if the serum APAP concentration is found to be in the nontoxic range when plotted on the nomogram. Therapy with NAC provides benefit by lessening the severity of hepatic necrosis and increasing chances for survival, even when begun more than 24 hours after ingestion. Therefore, it is never too late to begin NAC therapy; though efficacy begins to decrease if therapy is delayed beyond 16 hours.
A serum APAP concentration (measured at least 4 hours after ingestion) resulting in a value above the lower line of the nomogram necessitates a full course of NAC therapy (eg, standard oral loading dose of 140 mg/kg of NAC followed by 70 mg/kg every 4 hours for 17 oral doses) be delivered. NAC therapy should not be stopped prematurely simply because a repeat serum APAP concentration falls to zero or plots below the lower line on the nomogram.
Patients commonly experience nausea and vomiting following APAP ingestion, sometimes making it difficult to administer oral NAC therapy. In these cases, NAC has been given intravenously. An intravenous formulation is prepared in the pharmacy by aseptically passing sterile inhalable or oral NAC solution through a 0.2-μm filter and diluting it in 5% dextrose in water, to create an intravenous solution. An intravenous protocol commonly used in the United States is described in Fig. 23-3.
Occasionally, patients suffer symptomatic histamine release from intravenous NAC and require treatment with antihistamines. On rare occasions, patients suffer life-threatening anaphylactoid reactions requiring fluids, epinephrine, antihistamines, and corticosteroids. If the pharmacy cannot timely prepare intravenous NAC, treatment should not be delayed and oral NAC therapy should be started immediately. Treatment for adjunctive complications (eg, liver failure, renal failure) is entirely supportive and identical to that for other pregnant patients.
Fetal death from APAP overdose has been reported in all trimesters. Maternal NAPQI does not cross the placenta. Maternal APAP, however, does cross and has the potential to produce toxic fetal concentrations. The fetus’s ability to produce NAPQI from APAP begins as early as 14 weeks intrauterine life and increases until term. The fetus’s ability to detoxify APAP by conjugation with sulfate and glucuronide remains impaired until after birth, possibly shunting more APAP through cytochrome P450. The third-trimester fetus, therefore, appears to be at greatest risk for direct toxicity from APAP. Nevertheless, fetal loss appears to be most common in the first trimester—not because the fetus is necessarily poisoned, but because maternal illness is more likely to lead to fetal loss at that time.
Placental transfer of NAC (a class B medication) in humans has been documented with concentrations in the umbilical cord blood similar to maternal levels (Horowitz et al.). Because considerations of lack of first-pass effect through the fetal liver (compared to the maternal intake of NAC), most authorities prefer the parenteral use of the antidote during pregnancy. When NAC is given intravenously at currently used therapeutic doses, serum levels are 10 to 100 times higher.
At least one authority has recommended consideration for delivery of the mature fetus by cesarean section so that NAC therapy can be administered directly to the baby at risk (ie, when maternal serum APAP concentrations are toxic). This has to be balanced against the risks of the procedure related to coagulopathy. Advocates of immediate delivery state that the maternal and fetal risk of late third-trimester cesarean section are extremely low in comparison to data from the several case reports describing fetal death from APAP toxicity. The incidence correlates with delays in initiating therapy with NAC. Unfortunately, no animal or human studies have examined the benefits of immediate delivery followed by direct newborn NAC therapy. The published experience does not contain reports of fetal demise following acute single APAP ingestions if NAC therapy is begun promptly. No standard of care to guide clinicians who face this dilemma exists. Given the potential for a nonreassuring fetal condition, fetal monitoring of viable pregnancies is recommended during therapy.
Although it has not been proven, serial assessments of the fetal well-being are recommended upon discharge from a severe exposure to acetaminophen.
Large doses of iron are extremely toxic and may lead to multiorgan system dysfunction and death. Strong evidence indicates that the fetus is protected from elevated maternal iron levels. Iron poisoning is, almost entirely, a situation in which fetal survival depends on maternal survival. Table 23-1 and Fig. 23-4 summarize the pathophysiology and management of iron toxicity, respectively.
1. Iron is corrosive to the gastrointestinal tract, producing nausea, vomiting, diarrhea, abdominal pain, gastrointestinal bleeding, and rarely perforations. |
2. Systemically absorbed iron causes venodilatation and increased capillary permeability with associated third spacing of fluid. |
3. Iron causes cell dysfunction and death by disrupting ATP formation in mitochondria and by catalyzing the formation of oxygen-free radicals that destroy cell membranes. The liver takes the brunt of the injury with potential for fulminant hepatic failure, but in massive iron poisoning, any organ can be affected. |
4. Early after ingestion, high serum iron concentrations directly inhibit serine proteases (thrombin) and lengthen the prothrombin time, even in the absence of hepatic failure. |
To determine how much iron was ingested, the elemental iron content must be calculated. On a milligram basis, ferrous sulfate contains 20% elemental iron; ferrous fumarate 33% elemental iron; and ferrous gluconate 12% elemental iron. Any patient who ingests more than 20 mg/kg of elemental iron, any patient with symptoms, and/or any patient in whom the amount of ingested iron is not known requires an evaluation. During pregnancy, the prepregnancy weight should be used for calculation of the dose ingested. Keep in mind that if the mechanism of the poisoning was intentional, the history may be unreliable as patients may conceal or minimize the magnitude of the exposure.
Traditionally, clinical effects of iron poisoning are considered in four stages, although the distinctions between stages are not always clear.
Stage 1 is characterized by abdominal pain, vomiting, and diarrhea, and results from the corrosive effects of iron on the gut. Stage 1 begins 1 to 6 hours after ingestion. Hematemesis is possible, and hypovolemia may result in hypotension and metabolic acidosis. Serum iron levels may be normal or elevated at this stage.
Stage 2 is not always seen, but, when present, lasts for about 2 to 24 hours or so after Stage 1. Stage 2 is characterized by resolution of gastroenteritis, and patients commonly lie in bed quietly. Pallor, metabolic acidosis, and in the face of uncorrected hypovolemia, tachycardia and hypotension may be noted. Physicians may be falsely reassured by the resolution of gastroenteritis in the face of ensuing systemic iron toxicity as tissue iron stores rise. Tachycardia and hypotension are common if hypovolemia has not been corrected. Metabolic acidosis and hypotension result from uncorrected hypovolemia, venodilation, third-spacing of fluid, and the cytotoxic effects of iron. Serum iron concentration may be elevated and liver enzyme values are normal. PT may be elevated if serum iron concentrations are high from a direct effect of iron on serum proteases such as thrombin. In severe iron poisoning, the patient may rapidly progress from Stage 1 to Stage 3.
Stage 3 comprises systemic organ damage or failure from the cytotoxic effects of iron. Its onset is observed at any time from ingestion through 48 hours. Stage 3 is characterized by hepatic failure, lethargy/coma/convulsions, renal failure, and, occasionally, heart failure. Hypoglycemia and coagulopathy reflect hepatic damage. In this setting, metabolic acidosis has numerous causes including hepatic failure, low cardiac output, and impaired oxidative phosphorylation. The liver is the major organ affected by the toxicity of iron and typically is the first organ to fail. Stage 3 toxicity is associated with spontaneous abortion, preterm delivery, and maternal death.
Stage 4 is characterized by recurrence of vomiting (mostly in pediatric population) due to gastric outlet or small bowel obstruction from gastrointestinal scarring of mucosal ulcers several weeks after the poisoning (2-6 wks).
While most patients who suffer iron poisoning of Stage 2 or greater are thought to have peak serum concentrations more than 350 μg/dL, the actual peak level is seldom observed as a consequence of mistiming. Serum iron levels peak sometime between 2 and 6 hours after ingestion. Normal or mildly elevated serum iron concentrations can be misleading, since they do not always reflect the tissue iron burden, which is the one responsible for the systemic toxicity. Therefore, as an isolated finding, a normal serum iron concentration cannot always be used to exclude iron poisoning in the symptomatic patient. Obtaining a normal or low iron level more than 6 hours after ingestion may be misleading as the reason that the level is low is redistribution of iron in the tissues. Toxicity is still possible in these cases and the patient, not the numbers need to be treated. The measurement of total iron binding capacity (TIBC) does not assist in the treatment of acute iron poisoning since it is falsely elevated in the face of high serum iron concentrations by some methods and since serum iron concentrations may be quickly changing in their relationship to TIBC.
Ipecac emesis may be considered in the conscious patient within the first hour of exposure. Prolonged vomiting (>1 hour) should be attributed to iron toxicity rather than to the effects of ipecac. Generally, patients who remain completely asymptomatic for 6 hours after ingestion and have a normal physical examination do not require treatment for iron poisoning (Fig. 23-5). Asymptomatic patients who ingest more than 20 mg/kg of elemental iron but are seen within 6 hours might benefit from gastric lavage with saline. If vomiting has already occurred, it is not thought that gastric lavage would be of further benefit. One percent bicarbonate (200-300 mL) after lavage or induced emesis may promote the conversion of ferrous iron to ferrous carbonate (less soluble). Alternatively a single oral dose of 8% magnesium oxide (Milk of Magnesia; 60 mL/g of elemental iron ingested) significantly reduces iron absorption in healthy volunteers.
Patients are considered symptomatic when they present with more than minimal symptoms (eg, more than one emesis). These patients require treatment with fluids and deferoxamine mesylate, in addition to magnesium oxide (Table 23-2). When a patient has significant clinical symptoms, it is never prudent to wait for results of a serum iron concentration before beginning therapy, including deferoxamine. The traditional dose of deferoxamine is 15 mg/kg/h intravenously, not to exceed a daily dose of 6 grams.
|
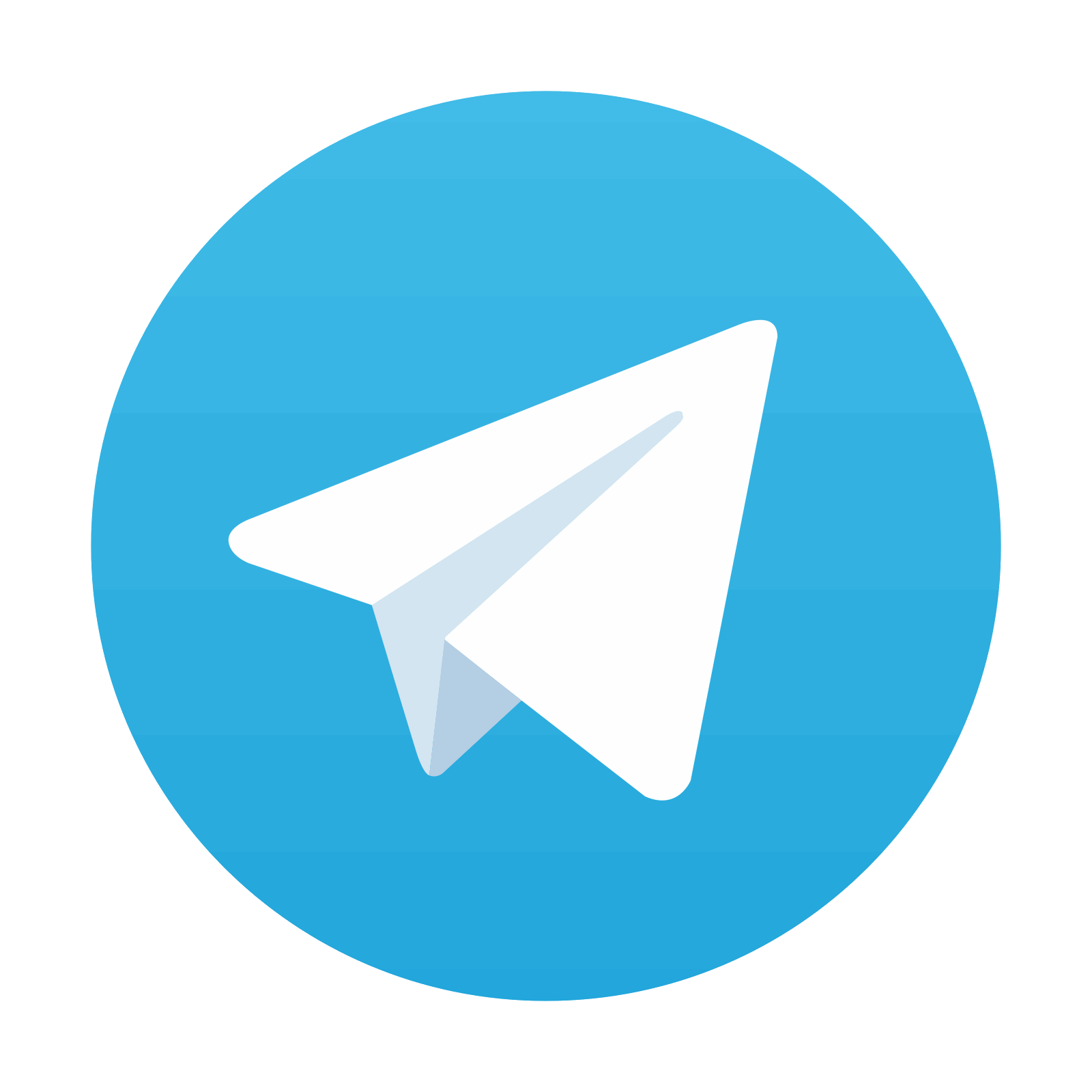
Stay updated, free articles. Join our Telegram channel

Full access? Get Clinical Tree
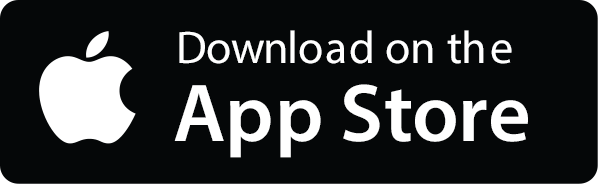
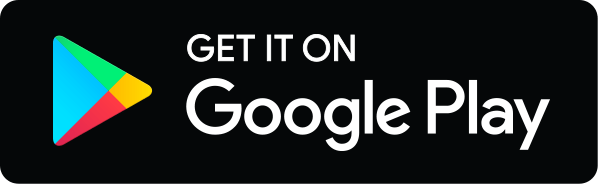
