Objective
To circumvent the complex protein milieu of plasma and discover robust predictive biomarkers for preeclampsia (PE), we investigate if phospholipid-binding ligands can reduce the milieu complexity by extracting plasma extracellular vesicles for biomarker discovery.
Study Design
Cholera toxin B chain (CTB) and annexin V (AV) which respectively binds GM1 ganglioside and phosphatidylserine were used to isolate extracellular vesicles from plasma of PE patients and healthy pregnant women. The proteins in the vesicles were identified using enzyme-linked immunosorbent assay, antibody array, and mass spectrometry.
Results
CTB and AV were found to bind 2 distinct groups of extracellular vesicles. Antibody array and enzyme-linked immunosorbent assay revealed that PE patients had elevated levels of CD105, interleukin-6, placental growth factor, tissue inhibitor of metallopeptidase 1, and atrial natriuretic peptide in cholera toxin B- but not AV-vesicles, and elevated levels of plasminogen activator inhibitor-1, pro-calcitonin, S100b, tumor growth factor β, vascular endothelial growth factor receptor 1, brain natriuretic peptide, and placental growth factor in both cholera toxin B- and AV-vesicles. CD9 level was elevated in cholera toxin B-vesicles but reduced in AV vesicles of PE patients. Proteome analysis revealed that in cholera toxin B-vesicles, 87 and 222 proteins were present only in PE patients and healthy pregnant women respectively while in AV-vesicles, 104 and 157 proteins were present only in PE and healthy pregnant women, respectively.
Conclusion
This study demonstrated for the first time that CTB and AV bind unique extracellular vesicles, and their protein cargo reflects the disease state of the patient. The successful use of these 2 ligands to isolate circulating plasma extracellular vesicles for biomarker discovery in PE represents a novel technology for biomarker discovery that can be applied to other specialties.
Preeclampsia (PE) remains one of the most common causes of adverse pregnancy outcome in developed and developing countries. The incidence of PE is substantial, about 3% to 8%. PE places the obstetric patient and her infant at substantial risk of preterm birth and perinatal mortality, and severe maternal hypertension and multisystemic organ dysfunction and damage, including eclampsia and abruption placentae. Predictive tests for preeclampsia early in the course of pregnancy would provide sufficient time to intervene and mitigate the risks of PE. There has been an intense interest in biomarkers for the identification of patients at risk for preeclampsia. Although clinical risk factors for preeclampsia are well known, these factors either singly or in combination have limited predictive values and this has led to intense search for predictive biomarkers for PE, particularly in plasma. However, plasma-derived predictive biomarkers like the generic disease biomarkers are generally low abundance proteins and their discovery is confounded by the dominance of several high abundance proteins such as albumin and immunoglobulins. Despite much effort to eliminate or reduce these abundant proteins, circumventing these high abundance plasma proteins remains a challenge. However, the recent extraction of membrane vesicles from bodily fluids such as plasma or urine for biomarker discovery inadvertently resolved this challenge as removal of the high abundance plasma proteins is inherent in the extraction of membrane vesicles.
The cell sources of these circulating vesicles are likely to be diverse as many cell types are known to secrete membrane vesicles. Because these vesicles are essentially fragments of the secreting cells, they and their cargo are microcosms of their cell sources and would reflect the physiologic or diseased state of the cells, making them potential sources of biomarkers for disease diagnosis or prognosis. Indeed, pregnancy-associated exosomes were reported as early as 2006. Circulating plasma vesicles are highly heterogeneous and several distinct classes of membrane vesicles have been described. They include microvesicles, ectosomes, membrane particles, exosome-like vesicles, apoptotic bodies, prostasomes, oncosomes, or exosomes, and are differentiated based on their biogenesis pathway, size, flotation density on a sucrose gradient, lipid composition, sedimentation force, and cargo content. Presently, these vesicles are isolated by differential and/or density gradient centrifugation that rely primarily on the size or density of the vesicles. Because size and density distribution are not discretely unique to each class of membrane vesicles, the present isolation techniques cannot differentiate between the different classes. Although immunoisolation techniques using antibodies against specific membrane proteins could enhance the specificity of membrane vesicle isolation, no membrane protein has been reported to be unique to a class of membrane vesicles or to a particular cell type. For example, although tetraspanins such as CD9, CD81 have often been used as exosome-associated markers, their ubiquitous distribution over the surface membrane of many cell types suggests a generic association with membrane vesicles. Also, such immunoisolation techniques cannot distinguish between membrane vesicles, protein complexes, or soluble receptors. The lack of specific isolation technique for each class of these membrane vesicles is further exacerbated by a lack of nomenclature standard to unambiguously define each class of membrane vesicle. It is also not clear if the present classification of vesicles describe unique entities.
To circumvent this conundrum and develop alternative techniques for isolating membrane vesicles, we focus on membrane lipid as the target for isolation. A defining feature of circulating membrane vesicles is the derivation of their bilipid membrane from the plasma membrane. The plasma membrane is a highly compartmentalized cellular structure with an ordered distribution of proteins and lipids that are highly restricted in their rotational and lateral diffusion within the plane of the membrane. This ordered distribution generates structurally and functionally unique microdomains, and such microdomains have been shown to be critical for membrane activities such as cell signalling, cell adhesion, and membrane trafficking. The best characterized microdomain to date is the lipid raft that is enriched in cholesterol and saturated lipids such as sphingolipids. Another microdomain is the caveolae that are specialized uncoated cell surface invaginations. Caveolae are generally viewed as a specialized subtype of lipid rafts. These lipid raft microdomains are organized by the lipid constituents, namely, cholesterol and sphingolipids. Nonlipid raft microdomains have been reported and these appeared to be organized by proteins eg, the actin cytoskeleton, galectin-1, K- and H-ras.
The compartmentalization of the plasma membrane into microdomains with specialized structures and functions suggest that the biogenesis of each class of membrane vesicles from the plasma membrane is microdomain-specific. Therefore, the membrane lipids of circulating vesicles could reflect the microdomain from which they were derived and may determine their composition and functions. Indeed, membrane of exosomes that originated from endosomes is reportedly enriched in cholesterol and GM1 gangliosides, and this enrichment appears to distinguish exosomes from other membrane vesicles. Cholesterol- and GM1 ganglioside-rich membranes are reflective of lipid rafts that represent the major sites of endocytosis. Exposed phosphatidylserine has been reported to be present on membrane of several extracellular vesicles including exosomes. Although monocytes and macrophages endothelial cells are known to secrete vesicles with exposed phosphatidylserines during inflammation, circulating vesicles with exposed phosphatidylserine in a healthy individual is thought to originate primarily from platelets. Together, the studies on membrane lipids of circulating vesicles suggest that circulating vesicles could be differentiated by their membrane phospholipid composition, specifically GM1 gangliosides and phosphatidylserines. As these 2 phospholipids are known to bind cholera toxin B chain (CTB) and annexin V (AV), respectively, CTB and AV are potentially ligands for extracting different populations of circulating vesicles.
In this study, we tested if circulating plasma membrane vesicles could be fractionated according to their affinity for CTB and AV, and if these fractionated vesicles could be used for discovery of PE biomarkers.
Materials and Methods
Plasma collection
The recruitment and enrollment of third trimester PE and matched healthy pregnant women by KK Women’s & Children’s Hospital were approved by the Singhealth Centralized Institutional Review Board (ref no: CIRB 2011/476/D). PE was defined as maternal systolic blood pressure of >140 mm Hg and/or diastolic blood pressure of >90 mm Hg on 2 occasions separated by 6 hours and proteinuria of 300 mg in a 24-hour period after 20 weeks of gestation as per guidelines of the American College of Obstetricians and Gynecologists. Peripheral blood was collected into ethylenediaminetetraacetic acid vacutainer tubes, centrifuged, and the plasma samples were stored at −80°C until analysis. The plasma samples were analyzed within 3 months and were not freeze-thaw more than twice.
There was a total of 11 PE patients and 11 healthy pregnant patients (controls) enrolled. The mean gestation age of PE presentation for the 11 PE patients was 30.5 weeks (range, 24.0–35.0 wks). The mean systolic and diastolic blood pressure of the 11 PE patients were 166 mm Hg (range, 148–182 mm Hg) and 97 mm Hg (range, 71–114 mm Hg), respectively.
The mean gestation of the 11 control and 11 PE patients at the time of collection were 31.9 weeks (range, 27.9–36.0 weeks) and 32.4 weeks (range, 28.4–38.0 weeks), respectively. The mean age of the control and PE patients were 27.7 years (range, 20–38 years) and 32.2 years (range, 21–38 years), respectively. The mean gravidity of the control and PE patients were 2.0 (range, 1–5) and 1.9 (range, 1–3), respectively. The mean parity of the control and PE patients were 0.7 (range, 0–3) and 0.2 (range, 0–1), respectively. The mean BMI of the control and PE patients were 24.8 kg/m 2 (range, 18.3–33.2 kg/m 2 ) and 30.8 kg/m 2 (range, 22.3–43.2 kg/m 2 ), respectively.
None of control patients had comorbidity. Nine of the 11 PE patients had severe preeclampsia (> BP 160/110). One PE patient subsequently developed eclampsia. One PE patient was severely obese (BMI 43.2 kg/m 2 ), whereas another had developed gestational diabetes.
Of the 11 control and 11 PE patients, 6 control and 6 PE patients were processed for analyses using both mass spectrometry and a commercially available array of antibodies. The remainder 5 control and 5 PE patients were processed for analysis using enzyme-linked immunosorbent assay (ELISA) for candidate biomarkers that were not covered in the standard commercial antibody array.
Isolation of GM1 ganglioside- and phosphatidylserine-rich membrane vesicles
CTB (SBL Vaccin AB, Stockholm, Sweden) and AV (Biovision, San Francisco, CA) was biotinylated using Sulfo-N-hydroxysulfosuccinimide Biotin (Thermo Scientific, Waltham, MA) as per manufacturer’s instruction. Ten microliters of plasma from each healthy and preeclampsia patients were incubated with 0.5 ηg biotinylated CTB or 0.5 ηg biotinylated AV in 100 μL binding buffer (2.5 mM calcium chloride, 0.01 M Hepes [Life Technologies, Grand Island, NY], and 0.14 M sodium chloride) for 30 minutes at 37°C in a rotating tube. At the same time, 100 μL of Dynabeads MyOne Streptavidin T1 (Life Technologies) was washed thrice with 100 μL wash buffer (0.1% bovine serum albumin in phosphate buffer saline) by vortex mixing the beads, immobilizing the beads with a magnet, and removing the supernatant for each wash. After removing the last wash buffer, the beads were resuspended in 100 μL binding buffer. Five microliters of the washed beads were then added to the plasma-CTB or plasma-AV reaction mix and incubated with rotation for 30 minutes. The beads were immobilized with a magnet and the supernatant was removed. The beads were then washed thrice with 200 μL AV binding buffer as described above. The bead-captured membrane vesicles were then analyzed by sodium dodecyl sulfate polyacrylamide gel electrophoresis (SDS-PAGE), ELISA, antibody array, and mass spectrometry.
SDS-PAGE
The beads were boiled in 28 μL of a standard denaturing/reducing SDS-PAGE loading buffer and resolved on 4-12% SDS–polyacrylamide gels.
ELISA
To assay for membrane proteins such as CD9, the beads were incubated with 1:500 dilution of mouse antihuman CD9 antibody (Santa Cruz Biotechnology, Santa Cruz, CA) with rotation for 30 minutes. The beads were then immobilized and supernatant was removed, washed thrice with 200 μL wash buffer, and then incubated with 1: 5000 HRP conjugated donkey antimouse IgG antibody (Santa Cruz Biotechnology) for 30 minutes with rotation at room temperature. After washing, the beads were incubated with 100 μL Amplex Red Substrate (Life Technologies) for 30 minutes and fluorescent intensity was measured at 530/590 ƞm (excitation/emission). To assay for luminal proteins, the bound vesicles are lysed with 100 μL of cell lysis buffer (Biovision). The lysed vesicles were then biotinylated by adding 10 μL 1:4000 diluted 10 mM Sulfo-NHS Biotin (Thermo Scientific, #21217). To assay for CD9, soluble fms-like tyrosine kinase-1, brain natriuretic peptide (BNP), atrial natriuretic peptide (ANP), placenta growth factor (PlGF), magnetic bead conjugated antibody specific for the protein of interest was then added. The antibody-bound protein was then immobilized by magnet and washed thrice as described above. The target protein was assayed using Amplex Ultra Red Substrate as described earlier.
Antibody array
For antibody array, CTB- and AV- vesicles were isolated from each of 6 PE patients and 6 healthy controls by incubating 30 μL of plasma with 1.5 ƞg biotinylated CTB or AV, respectively. The isolated vesicles were lysed as described previously and analyzed for proteins using RayBio Custom Quantibody Array (cat. QAH-CUST) according to manufacturer’s instructions (RayBiotech, Norcross, GA).
Mass spectrometry
For mass spectrometry, 300 μL of pooled plasma from either 6 PE patients or 6 healthy controls was incubated with 15 ng CTB or AV to isolate CTB- and AV- vesicles. The 60 μL of the washed beads prepared as described above were then added to the plasma-CTB or plasma-AV reaction mix and incubated with rotation for 30 minutes. The beads were immobilized with a magnet and the supernatant was removed. The beads were then washed thrice with 200 μL AV binding buffer as described above. The isolated vesicles were lysed and resolved on a protein gel. Each gel lane was sliced separately into 8 pieces. The gel pieces were destained; proteins in the gel were reduced by 10 M dithiothreitol at 56°C for 1 hour and alkylated by 55 mM iodoacetamide for 45 minutes in the dark at room temperature. Tryptic digestion was performed by using porcine trypsin (Sequencing Grade Modified, Promega, WI) overnight. The tryptic peptides were extracted by 5% formic acid in 50% acetonitrile and vacuum dried by speedvac. Each dried fraction was reconstituted in 100 μL of 0.1% formic acid and analyzed using a linear ion trap–Fourier transform (LTQ–FT) Ultra mass spectrometer (Thermo Electron, Bremen, Germany) coupled with a ProminenceTM HPLC unit (Shimadzu, Kyoto, Japan). For each analysis, samples was injected from an autosampler (Shimadzu) and concentrated in a Zorbax peptide trap (Agilent, Palo Alto, CA). The peptide separation was performed in a capillary column (75 μm inner diameter × 15 cm) packed with C18 AQ (5 μm particles, 300 Å pore size; Michrom Bioresources, Auburn, CA). Mobile phase A (0.1% formic acid in H 2 O) and mobile phase B (0.1% formic acid in acetonitrile) were used to establish the 90 minute gradient comprising 3 minutes of 0-5% B and then 52 minutes of 5-25% B followed by 19 minutes of 25-80% B, maintenance at 80% B for 8 minutes, and finally reequilibration at 5% B for 8 minutes. The HPLC system was operated at a constant flow rate of 30 μL minute −1 , and a splitter was used to create an effective flow rate of approximately 300 nL minute −1 at the electrospray emitter. The sample was injected into an LTQ-FT through an Advance CaptiveSpray source (Michrom Bioresources) with an electrospray potential of 1.5 kV. The gas flow was set at 2, ion transfer tube temperature was 180°C, and collision gas pressure was 0.85 millitorr. The LTQ-FT was set to perform data acquisition in the positive ion mode as described previously. Briefly, a full mass spectrometry (MS) scan (350–1600 m/z range) was acquired in the FT-ICR cell at a resolution of 100,000. The linear ion trap was used to collect peptides and to measure peptide fragments generated by CID. The 10 most intense ions above a 500-count threshold were selected for fragmentation in CID (MS2). For each experiment, MS/MS (dta) spectra of the 8 gel fractions were combined into a single mascot generic file by a home-written program. Protein identification was achieved by searching the combined data against the international protein index human protein database (version 3.34; 69,164 sequences, 29,064,825 residues) via an inhouse Mascot server (version 2.3.02; Matrix Science, London, UK). The search parameters were: a maximum of 2 missed cleavages using trypsin; fixed modification was carbaminomethylation of cysteine, and variable modifications was oxidation of methionine. The mass tolerances were set to 10 ppm and 0.8 Da for peptide precursor and fragment ions respectively. Protein identification was accepted as true positive if 2 different peptides were found to have scores greater than the homology or identity scores.
Statistical analysis
Statistical analysis was performed using Mann–Whitney U test. Differences were considered to be statistically significant when the P values were less than .05.
Results
CTB and AV extracted protein containing vesicles from plasma
Plasma was incubated with biotinylated CTB or AV followed by streptavidin-conjugated magnetic beads. After extensive washing, the beads were boiled in a protein reducing and denaturing buffer, and analyzed by SDS-PAGE for protein ( Figure 1 ). The amount of protein extracted from 5 μL plasma by CTB or AV was less than that in 0.01 μL plasma or less than 0.1% of the starting protein concentration. Despite the relatively low resolution of a 2D-gel, there were distinct differences in the protein profile in the CTB- and AV-lipid vesicles ( Figure 1 ).

Presence of distinct CTB- and AV-vesicle populations in the plasma
Plasma was first extracted for either CTB- or AV-vesicles followed by extraction for AV- and CTB-vesicles, respectively. The extracted vesicles were then assayed for CD9, a ubiquitous membrane protein which was used here as a surrogate marker for plasma membrane. The level of CD9 in CTB-vesicles was similar before and after depletion with AV ( Figure 2 ). Likewise, the level of CD9 in AV-vesicles was similar before and after depletion with CTB. Because neither of the vesicles was depleted by extraction of the other vesicle, the 2 vesicles did not share an affinity for either ligands and were distinct populations.

Candidate biomarkers for PE segregated differently in circulating CTB- and AV-vesicles of preeclampsia and healthy pregnant women
Vesicles were isolated from plasma of preeclampsia and matched healthy pregnant women. They were then assayed for the presence of previously reported preeclampsia biomarkers using either ELISA or a commercially available antibody array. Plasma from 2 different sets of preeclampsia patients and matched healthy controls were used; 1 for each assay.
Using a commercially available array of antibodies, CTB- and AV-vesicles from 6 PE patients and 6 matched healthy controls were assayed for angiotensin-converting enzyme 2, angiopoietin 1, C reactive protein, E-selectin, endoglin (CD105), growth hormone, interleukin-6, P-selectin, plasminogen activator inhibitor-1 (PAI-1), PlGF, procalcitonin, S100b, tumor growth factor β, tissue inhibitor of metallopeptidase 1, and tumor necrosis factor α ( Figures 3 and 4 ). Four proteins, namely CD105, interleukin-6, PlGF, and tissue inhibitor of metallopeptidase 1 were significantly elevated in only CTB- but not AV-vesicles of preeclampsia patients. Another 4 PAI-1, procalcitonin, S100b, tumor growth factor β were elevated in both CTB- and AV-vesicles of PE patients.


For other candidate biomarkers that were not covered in the antibody array, CTB- and AV-vesicles from 5 PE patients and 5 matched controls were assayed by ELISA. The proteins assayed were CD9, vascular endothelial growth factor receptor 1 (VEGFR1), BNP, ANP, and PlGF. ANP was significantly increased in the CTB- but not AV-vesicles of PE patients although VEGFR1, BNP, and PlGF were significantly increased in both CTB- and AV-vesicles of PE patients ( Figure 5 ). The statistically significant increased PlGF level ( P = .047) in AV-vesicles of PE patients contrasted with its insignificant increase ( P = .055) when assayed using antibody arrays. This discrepancy could be a statistical anomaly as the 2 assays were conducted using small samples of 2 independent sets of patients and controls ( P = .055). CD9 was significantly decreased in the CTB- but significantly increased in AV-vesicles of PE patients.

Together, these 2 studies demonstrated that previously reported candidate biomarkers for PE were present and differentially distributed in CTB- and AV-vesicles of PE patients relative to matched healthy controls.
Proteomic analysis of the CTB- and AV-vesicle populations in the plasma of preeclampsia and healthy pregnant women
For a comprehensive proteomic analysis of the CTB- and AV-vesicles from the pooled plasma of 6 preeclampsia and 6 healthy pregnant women, proteins in these vesicles were identified by mass spectrometry. A total of 285 and 269 proteins were detected in the CTB- and AV-vesicles of PE patients respectively, whereas 420 and 322 proteins were detected in those of healthy controls ( Figure 6 ). Of the 285 and 420 proteins in the CTB-vesicles of PE and healthy pregnant women, 198 proteins were found in the CTB vesicles of both patient groups. Likewise, 165 proteins were found in the AV-vesicles of both patient groups. Therefore, the remaining proteins that were present only in the vesicles of either PE or healthy pregnant women, ie, 87 CTB-proteins of PE patients, 104 AV-proteins of PE patients, 222 CTB-proteins of healthy pregnant women and 157 AV-proteins of healthy pregnant women ( Figure 6 ) represented candidate PE biomarkers ( Tables 1 and 2 ). Twenty-four of the 87 CTB- and 104 AV-proteins were found in both vesicles whereas 67 of the 222 CTB- and 157 AV-proteins in the control group were present in both vesicles ( Table 3 ). Eleven of the 87 CTB-proteins in PE patients were present in AV-vesicles of healthy pregnant women whereas 17 of the 104 AV-proteins in PE patients were present in CTB-vesicles of the matched control group ( Tables 4-10 ). These observations indicated that the candidate biomarkers were distributed in all possible permutations between the 2 vesicle types of PE patients vs healthy pregnant women. Therefore, a single PE biomarker could be differentially expressed in the 2 vesicles of a pregnant woman. This differential expression would potentially increase the robustness of the biomarker and facilitate comparison between patients by determining the ratio of the biomarker in the 2 vesicles.

Proteins | ||
---|---|---|
ANTXR1 | GGT1 | LONRF3 |
APC | HSPA5 | MMP7 |
APOA1 | HSPA7 | NF1 |
APOC1 | IGFBP3 | NID1 |
APOC2 | IGKV2-40 | NONO |
APOE | IGLV8-61 | NT5C3L |
ARFIP1 | INHBC | ORM1 |
ARL5B | INHBE | PCF11 |
ATPAF1 | IPI00045460 | PEG3 |
C7 | IPI00385253 | PRL |
C9orf4 | IPI00385555 | PRX |
CD37 | IPI00387025 | RELN |
CDC2L1 | IPI00479426 | RPS6 |
CDC6 | IPI00796011 | SAA1 |
CDKL2 | IPI00827643 | SERPINA6 |
CIB2 | KIF27 | TFAP2E |
CSHL1 | KRT27 | THBS3 |
E2F7 | KRT28 | UBA1 |
EFEMP1 | KSR2 | UNC13B |
F13A1 | LOC100292046 | VPS4A |
GCA | LOC441687 | WDR60 |
Proteins | |||||
---|---|---|---|---|---|
A1BG | C8G | FGA | IPI00384392 | KRT8 | RAB10 |
ACTB | C9 | FGB | IPI00384401 | KRT9 | RAB15 |
ACTBL2 | CAMP | GC | IPI00384407 | LBP | RAB1B |
ADAM12 | CASP12 | GDF15 | IPI00385264 | LCP1 | RAB35 |
AGT | CD14 | GH2 | IPI00385985 | LOC100126583 | RAB8A |
AHSG | CD5L | GPLD1 | IPI00387026 | LOC100290320 | RAN |
AMBP | CDADC1 | GPX3 | IPI00387106 | LOC100291682 | RARRES2 |
APCS | CDC5L | GSN | IPI00387116 | LOC401847 | SAA4 |
APOA2 | CDH1 | HAUS3 | IPI00387118 | LOC55908 | SCFV |
APOA4 | CDH23 | HBB | IPI00783024 | LPA | SEPP1 |
APOA5 | CDH5 | HBD | IPI00791534 | LRRC8B | SERPINA10 |
APOB | CETP | HP | IPI00807428 | LYZ | SERPINA3 |
APOC3 | CFB | HSP90AA1 | IPI00816799 | MAGT1 | SERPINA4 |
APOC4 | CFH | HSP90B1 | IPI00829827 | MASP2 | SERPINC1 |
APOD | CLTC | IGFALS | IPI00830088 | MGAT1 | SERPING1 |
APOF | CLU | IGHA1 | IPI00909649 | MGP | SHBG |
APOL1 | COL18A1 | IGHG2 | IPI00922295 | PCMT1 | SIGLEC16 |
APOM | COL6A3 | IGHG4 | IPI00940451 | PF4 | SIL1 |
ASPN | CP | IGHM | IPI00941961 | PGLYRP2 | SPP2 |
ATP6AP2 | CPB2 | IGHV3-48 | IPI00944652 | PLTP | SRI |
B4GALT1 | CRP | IGJ | ITGA2B | PLXND1 | SVEP1 |
BMP1 | CSH1 | IGK@ | ITIH1 | PON1 | TF |
C1QC | DAK | IGKV3-20 | ITIH2 | PON3 | THBS1 |
C1R | DEFA1 | IGLC1 | ITIH3 | PPBP | THBS4 |
C1RL | DNAH6 | IGLV7-43 | ITIH4 | PRDX6 | THSD1 |
C1S | EBI3 | IPI00003469 | KRT1 | PRG4 | TLL2 |
C20orf3 | ENO1 | IPI00003470 | KRT10 | PRKDC | TRAP1 |
C3 | F2 | IPI00007899 | KRT14 | PROS1 | TSKU |
C4A | F5 | IPI00186448 | KRT2 | PRSS3 | TTR |
C4B | F7 | IPI00382420 | KRT4 | PSG1 | UBE2N |
C4BPA | FBLN1 | IPI00382436 | KRT5 | PSG4 | VCL |
C5 | FBLN5 | IPI00382440 | KRT6B | PZP | VTN |
C8B | FETUB | IPI00382481 | KRT77 | QSOX1 | VWF |
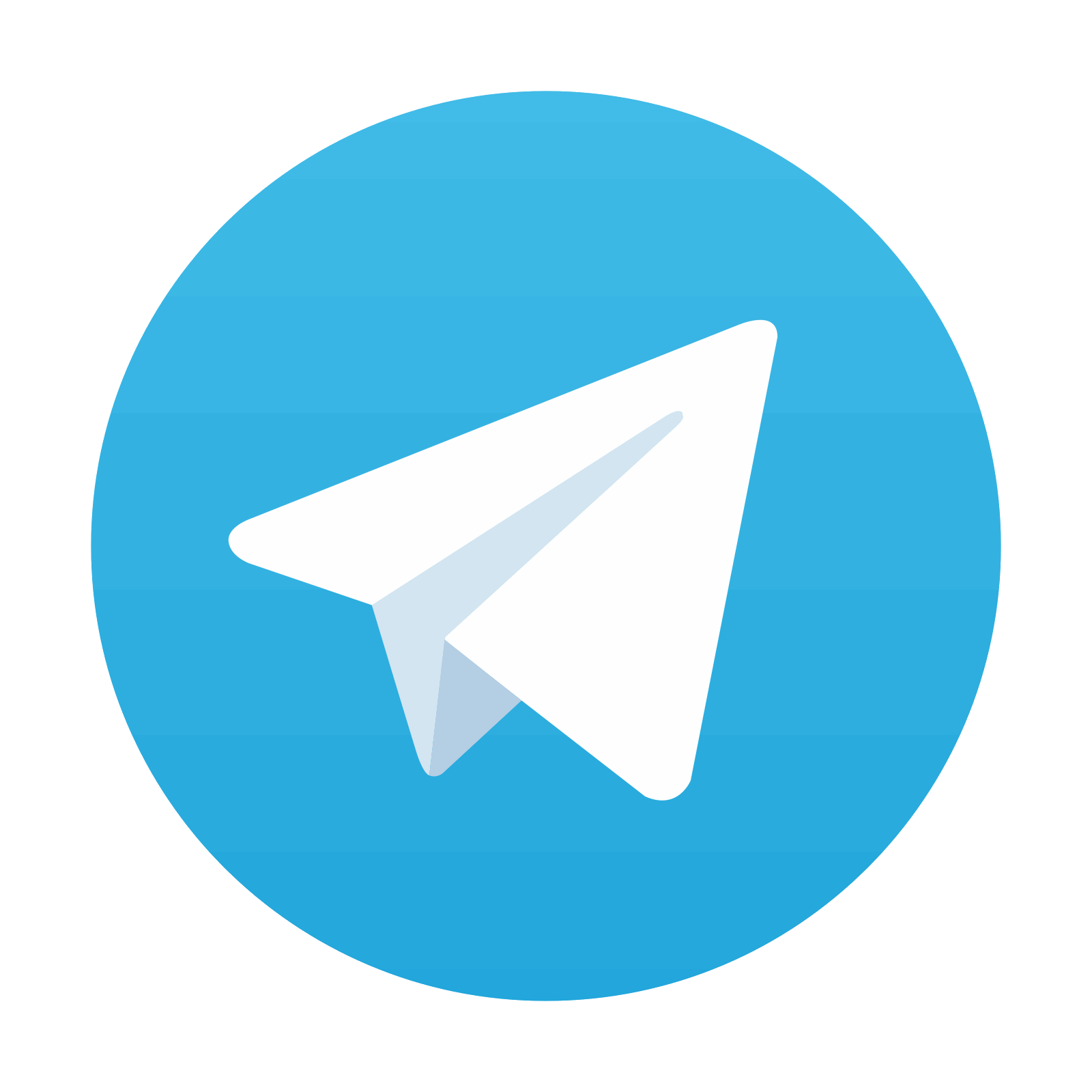
Stay updated, free articles. Join our Telegram channel

Full access? Get Clinical Tree
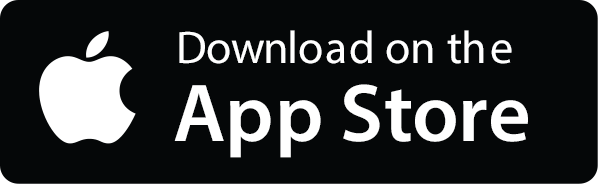
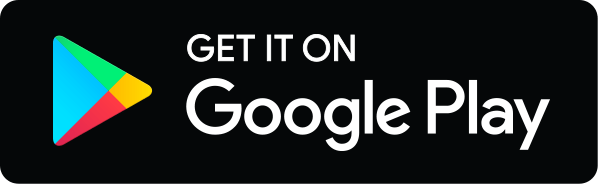