Objective
To determine placental gene expression of endothelial and inducible nitric oxide synthases and measure nitric oxide levels in patients with hemolysis, elevated liver enzyme levels, and low platelet count syndrome.
Study Design
Preterm placentas were obtained from 15 patients with hemolysis, elevated liver enzyme levels, and low platelet count syndrome and 30 controls matched for age, parity, and gestational age. mRNA levels were evaluated by real-time polymerase chain reaction, whereas nitric oxide and peroxynitrite production was measured by a commercially available kit.
Results
Placental gene expression of inducible nitric oxide and endothelial nitric oxide synthases were significantly lower in the hemolysis, elevated liver enzyme levels, and low platelet count syndrome group than in controls, whereas nitric oxide and peroxynitrite production were significantly higher in hemolysis, elevated liver enzyme levels, and low platelet count syndrome compared with controls.
Conclusion
The reduced endothelial nitric oxide and inducible nitric oxide synthases gene expression in women with hemolysis, elevated liver enzyme levels, and low platelet count syndrome may indicate extreme placental dysfunction that is unable to compensate the endothelial derangement and the related hypertension. The higher nitric oxide formation found in hemolysis, elevated liver enzyme levels, and low platelet count syndrome placentas could be explained as a counteraction to the impaired fetoplacental perfusion, typical of the syndrome.
Nitric oxide (NO) is a free radical and an important modulator of cellular responses in a variety of tissues, including those involved in human reproduction. NO is generated from L-arginine by the catalytic action of the enzyme nitric oxide synthase (NOS). So far, 3 different isoforms of NOS have been identified, cloned, and characterized. Although 2 of the 3 (type I or neuronal or nNOS and type III or endothelial or eNOS) are constitutively expressed in a variety of tissues, the expression of the third isoform (type II or inducible or iNOS) can be induced by cytokines and some other agents. NO is known to have a powerful vasodilating effect and inhibits vasospasm, platelet activation, and leukocyte activation. These are the features of endothelial cell damage that are considered pivotal in the pathogenesis of all pregnancy disorders. The interaction between NO and the superoxide anion (O2−.) produces the relatively long-lived potent prooxidant peroxynitrite (ONOO−), which is highly toxic, induces lipid peroxidation, and nitrates tyrosine residues on proteins, thus inhibiting signal transduction. HELLP syndrome (hemolysis, elevated liver enzyme levels, and low platelet count), is a dangerously severe complication of pregnancy characterized by a multisystemic thrombotic microangiopathy.
HELLP syndrome occurs in about 0.5% to 0.9% of all pregnancies and in 10% to 20% of cases with severe preeclampsia (PE). The complications of HELLP are long-recognized outcomes of severe preeclampsia-eclampsia but were first termed HELLP syndrome by Weinstein. HELLP is currently regarded as a variant of severe preeclampsia or a complication. In literature, the reported maternal mortality from HELLP is 1%, and the perinatal mortality rate ranges from 7.4%–34% and may be due to premature detachment of placenta, intrauterine asphyxia, and prematurity. Endothelial cell damage is considered to play a key role in the pathogenesis of both PE and HELLP even if the mechanism is not fully understood. Different studies highlighted that the oxidative stress in placenta leads to thrombosis, inflammation, and endothelial cell dysfunction. Although the cause is unclear, there exists accumulated evidence for a pathogenic model of PE and hypertensive disorders of pregnancy, whereby a deficiency in trophoblastic invasion of the placental bed leads to a poorly perfused fetoplacental unit. The clinical features, caused by a combination of endothelial damage, generalized vasoconstriction, and activation of coagulation, have been well described. The reduction in uteroplacental perfusion is the cause of the commonly observed fetal growth restriction, which together with prematurity in those needing early delivery, is a major risk factor for neonatal morbidity and mortality. There have been several studies on the role of endothelium-derived relaxing factor, such as NO in the control of placental vascular tone and in the establishment and maintenance of the fetoplacental circulation. Moreover, it has been suggested that impairment in the L-arginine-NO system is involved in the pathophysiology of hypertensive disorders of pregnancy.
Previously, our group demonstrated lower levels of iNOS in the placental bed from pregnancies complicated by HELLP syndrome than in normal pregnancies. However, in the same study, there was no significant difference in eNOS expression between normal and HELLP syndrome pregnancies.
The aim of our study was to evaluate placental expression levels of eNOS and iNOS in patients with HELLP syndrome using real-time quantitative PCR. Moreover, in the same patients NO and ONOO− tissue levels were evaluated.
Materials and Methods
Patient recruitment and tissue collection
Fifteen patients with HELLP syndrome and 30 healthy pregnant controls comparable for maternal and gestational age were enrolled for the study. All women had singleton pregnancy and were white. The study was performed in accordance with the principles of the Declaration of Helsinki as revised in 2001. The study was approved by the local institutional review board, and informed consent was obtained.
HELLP syndrome was defined as new onset of severe hypertension (systolic blood pressure ≥160 mm Hg and/or diastolic blood pressure ≥110 mm Hg) with platelet count of <100,000 cells/mm 3 , aspartate aminotransferase activity ≥70 IU/L, and lactate dehydrogenase activity >600 IU/L.
All women recruited, cases and controls, received a single course of bethamesone for fetal lung maturity (12 mg/d for 2 days) within 3 days before delivery, and no patient received magnesium sulphate before delivery. Specific exclusion criteria for the control group included a history of hypertension, renal disease, cardiac disease, diabetes mellitus, thyroid and immunologic diseases, congenital or acquired thrombophilic disorders, and the presence of chromosomal and other fetal anomalies. Gestational age was determined, by our internal obstetric protocol, by reference to the last menstrual period and crown rump length measurements between 8 and 12 weeks of gestation, confirmed by an early second trimester ultrasonographic examination. To rule out any impact of labor on placenta, only the women who delivered by elective cesarean section preceding labor were recruited. In the HELLP group, the reason for cesarean section was based on deterioration of maternal or fetal condition. A group of 30 patients matched for gestational age who underwent preterm cesarean section for medical problems unrelated to placental development were recruited as controls. Namely, the indications for cesarean section in this control group were fetal anomalies not associated to genetic causes, such as gastroschisis or congenital heart disease, acute maternal cerebral trauma, maternal congenital heart defect unrelated to hypertension and to cyanogenic cardiopathies, breast cancer, and cervical cancer. Intrauterine fetal growth restriction and preterm labor were not included in the controls. Placental tissue was taken immediately after delivery. Two tissue blocks of whole placenta (1 × 1 × 1 cm) were cut from the lower third of the cotyledons of each placenta and placed immediately into liquid nitrogen. Once frozen, the placental specimens were stored at −80°C until analysis. The total processing time length was less than 10 minutes.
RNA extraction
A piece of the frozen tissue (20-40 mg) was homogenized in lysis buffer, and the total RNA was extracted with an RNA isolation kit (Promega, Madison, WI). RNA samples were tested by ultraviolet absorption at 260 nm to determine RNA concentration. The quality and concentration of the RNA samples were further confirmed by electrophoresis on denaturated 1% agarose gels. Two micrograms of RNA were reverse transcribed in a total volume of 25 μL for 60 minutes at 37° C with M-MLV Reverse Transcriptase (Promega) using random nonamers to obtain complementary DNA (cDNA).
Real-time quantitative PCR
cDNA was used for real-time quantitative PCR. To avoid false-positive results attributable to the amplification of contaminating genomic DNA in the cDNA preparation, the primers were selected to flank an intron, and PCR efficiencies were tested and found to be close to 1. The following primers were used: 5′-GTGGCTGTCTGCATGGACCT-3′ (forward) and 5′-CCACGATGGTGACTTTGGCT-3′ (reverse) for eNOS, 5′-TGCAGACACGTGCGTACTCC-3′ (forward) and 5′-GGTAGCCAGCATAGCGGATG-3′ (reverse) for iNOS, 5′-TCCACTGGCGTCTTCACC-3′ (forward) and 5′- GGCAGAGATGATGACCCTTTT-3′ (reverse) for GAPDH. The genes were run in duplicate using SYBR Green chemistry. All samples, taken from each pregnancy, were tested in triplicate using as reference the GAPDH gene for data normalization to correct for variations in RNA quality and quantity.
Western blot
To confirm the real-time PCR results, we made a Western blot. Tissue extracts were prepared with RIPA lysis buffer containing 1 × phosphate-buffered saline (PBS), 1% Igepal CA-630, 0.5% sodium deoxycholate, 0.1% SDS, 10 mg/mL PMSF, aprotinin, 100 mM sodium orthovanadate, and 4% protease inhibitor cocktails. As a positive control, protein extracts from mouse liver were also loaded in all Western blots. Samples containing 50 μg proteins were subjected to 8% sodium dodecyl sulfate-polyacrylamide gel and electrophoresed for 1 hour at 130 V along with a molecular weight marker (Precision Plus Protein Standards; Bio-Rad Laboratories, Inc, Hercules, CA). The resolved protein bands were then transferred onto PVDF membranes at 100 V for 60 minutes using a transfer buffer of 25 mmol/L Tris base, 192 mmol/L glycine, and 20% methanol. The blots were blocked overnight at 4°C with blocking buffer (containing 1 × PBS, 5% nonfat milk, and 0.05% Tween 20). After regular blocking and washing, the membranes were incubated for 1 hour with mouse monoclonal antibody antiendothelial nitric oxide synthase (eNOS, 1:3000 dilution; Sigma-Aldrich, St Louis, MO) or with rabbit polyclonal antibody anti-inducible NOS (iNOS, 1:200 dilution; Abcam Inc, Cambridge, MA) diluted in blocking buffer. Blots were then washed using washing buffer (1 × PBS and 0.05% Tween 20) and incubated with horseradish peroxidase-conjugated anti-mouse immunoglobulin G (IgG) (1:80000 dilution; Sigma-Aldrich) for eNOS detection or incubated with horseradish peroxidase-conjugated anti-rabbit Ig G (1:2000 dilution; Pierce, Rockford, IL) for iNOS detection. We visualized either eNOS and iNOS proteins using an enhanced chemiluminescent substrate (SuperSignal West Femto Maximum Sensitivity Substrate; Pierce Biotechnology, Rockford, IL). The intensities of protein bands were evaluated from pictures obtained using Kodak 1D Image Analysis Software (Eastman Kodak Company, Rochester, NY).
NO production
The NO released was measured as nitrite/nitrate using a commercially available kit (Total Nitric Oxide Assay Kit, Stressgen; Assay Designs, Inc, Ann Arbor, MI). The kit allows for the total determination of both NO products in the sample by conversion of all the sample nitrate into nitrite, followed by the determination of total concentration of nitrite in the sample. All measurements were carried out in quadruplicate according to the manufacturers’ instructions at 540 nm with a microplate reader (Sunrise; Tecan Group Ltd, Männedorf, Switzerland). The amount of NO in each tissue extract, obtained as reported previously, was determined using a standard curve; protein concentration was determined with the Bradford BioRad protein assay using serum albumin as a standard and NO concentration was expressed as nmol NO/mg protein.
Preparation of DCF-free base
DCFDA free base was prepared daily, by mixing 0.05 mL 10 mM/L DCFDA with 2 mL 0.01 N NaOH, at room temperature for 30 minutes. The mixture was neutralized with 18.0 mL 25 mmol/L PBS pH 7.4. This solution was maintained on ice in the dark until use.
Peroxynitrite determination
NO is able to combine, in a rate limiting reaction catalyzed by iNOS, with superoxide anions to generate peroxynitrite, a nitrogen free radical that produces relevant peroxidative damage. Peroxynitrite levels were evaluated using the fluorescence probe 2,7-dichlorofluorescein diacetate (DCFDA) as previously described. Briefly, tissue extracts were incubated for 15 minutes with 5 μM DCFDA-free base at 37°C. Then the DCFDA treated samples were incubated with or without addition of L-arginine 100 mM and NG-monomethyl-L-arginine (L-NMMA, that is a specific inhibitor of NO production) 100 mM for 15 minutes at 37°C in the dark. After washing in PBS pH 7.4, cells were broken by sonication. The mixture was then centrifuged at 1000 rpm for 5 minutes and the fluorescence was measured in the supernatant in a Perkin-Elmer LS-50B spectrofluorimeter (Perkin-Elmer, Waltham, MA), at an excitation wavelength of 475 nm and emission wavelength of 520 nm. ONOO− production was expressed in arbitrary units/mg protein.
Statistical analysis
All statistical analyses were performed by using the Statistica software (version 6.0; Stat Soft, Tulsa, OK). The Mann-Whitney U test was performed to compare data from control and pathological placentas.
Differences were considered significant at P < .05. The results were obtained as “fold changes” in relative gene expression of the study group with respect to controls.
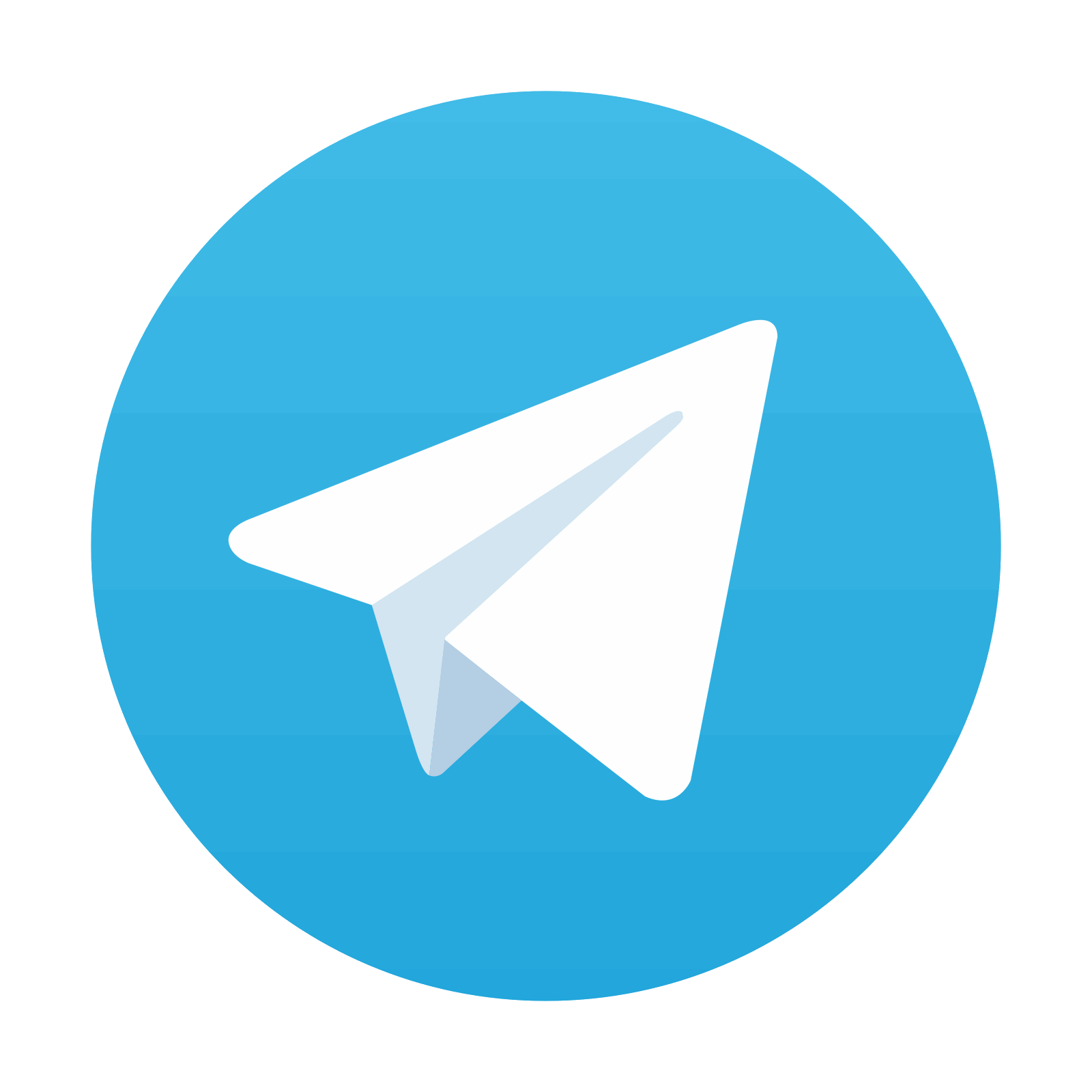
Stay updated, free articles. Join our Telegram channel

Full access? Get Clinical Tree
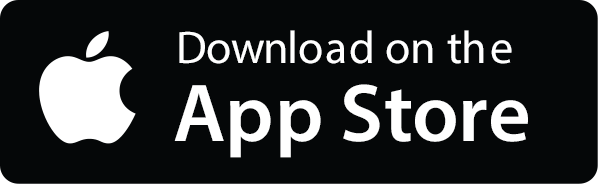
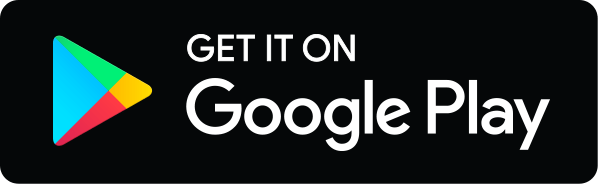