Objective
The purpose of this study was to investigate the expression and subcellular localization of laeverin, a placenta-specific membrane-bound aminopeptidase, in preeclamptic placentas and its role in trophoblast cell migration and invasion.
Study Design
Expression of laeverin was investigated in 6 normal and 6 preeclamptic placentas with the use of immunofluorescence, sodium dodecylsulfate-polyacrylamide gel electrophoresis with Western blot analysis and immunoelectron microscopy. The role of laeverin in trophoblast migration and invasion was studied with the use of the xCelligence system and Boyden chambers with Matrigel in HTR-8/SVneo cells. The effect of laeverin gene-silencing on selected genes that are involved in cell transformation and tumorigenesis was evaluated by polymerase chain reaction array. The Student t test, Mann-Whitney U test, χ 2 test, or F-test was used to compare groups as appropriate.
Results
Laeverin was expressed in the cell membrane of villous trophoblasts in third-trimester healthy placentas; in preeclamptic placentas, it was expressed ectopically in the cytoplasm, especially in microvesicles. Immunoelectron microscopy showed laeverin leakage into the fetal capillaries and abundant expression in microvesicles in preeclamptic placentas. Migration and invasion of HTR-8/SVneo cells were reduced by 11.5% ( P = .023) and 56.7% ( P = .001), respectively, by laeverin gene–silencing. Analysis of downstream pathways affected by laeverin-silencing demonstrated significant down-regulation of integrin A2 (39-fold), integrin B3 (5-fold), and matrix metalloprotease 1 (36-fold).
Conclusion
Expression of laeverin protein is altered in preeclamptic placentas. Its ectopic expression in the cytoplasm and microvesicles, rather than the cell membrane and leakage into the fetal capillaries, may have a role in the pathophysiologic condition of preeclampsia. Laeverin gene appears to be involved in trophoblast cell migration and invasion through interaction with integrins and matrix metalloprotease 1.
Preeclampsia complicates 5-10% of pregnancies and is a major cause of maternal mortality worldwide. Although it is clearly a placenta-specific disorder, its pathogenesis is not understood fully. Therefore, its prediction, timely diagnosis, and appropriate management remain challenging.
Laeverin, a membrane-bound aminopeptidase, was first reported to be expressed by human trophoblast cells in 2004 by Fujiwara et al and has been suggested to cooperate with the chemokine system in the regulation of human placentation. The same group recently presented some molecular evidence suggesting that laeverin is important for extravillous trophoblast invasion. Laeverin is a trophoblast-specific protein; however, it has been reported to be expressed in other tissues in some inflammatory diseases, such as rheumatoid arthritis. In a previous study, comparing global placental gene expression profile between preeclamptic and healthy pregnancies, we found 16 genes that were able to predict preeclampsia phenotype in our study population. Laeverin was among those genes, and it was up-regulated significantly in the preeclamptic placentas. Therefore, we hypothesized that the deregulation of laeverin protein may lead to abnormal trophoblast function and have a role in the pathophysiologic condition of preeclampsia.
In the present study, we investigated the expression of laeverin protein and its subcellular localization in healthy and preeclamptic placentas. Additionally, we investigated the role of laeverin in trophoblast cell migration and invasion.
Materials and Methods
The study was approved by the Regional Committee for Medical and Health Research Ethics-North Norway (REK Nord reference no. 2010/2058-4), and informed written consent was obtained from all the participants.
Placental samples from a total of 12 pregnant white European women (6 healthy and 6 with severe preeclampsia) were collected. They were matched for maternal age, parity, onset of labor (spontaneous or induced), and the mode of delivery (vaginal or cesarean), and the results were compared. Severe preeclampsia was defined as blood pressure ≥160/110 mm Hg and proteinuria ≥300 mg/24-hour urine or ≥2+ in spot urine after 20 weeks of gestation in previously normotensive women. Women with preexisting medical conditions that may have affected the course and outcome of pregnancy were excluded. Doppler ultrasonography was performed at ≤48 hours before delivery in each case to assess uteroplacental and umbilical circulation.
Collection of maternal blood and placental samples
Maternal venous blood samples were taken at ≤48 hours before delivery for the analysis of hemoglobin, hematocrit, liver function, and renal function. None of the women were in labor when blood samples were taken. Placental tissue samples were obtained immediately after delivery, as described previously.
Cell line
The immortalized HTR-8/SVneo trophoblast cell line, which was obtained from primary cultures of human trophoblast cells, was used for migration, invasion, and polymerase chain reaction (PCR) array studies. Cells were maintained in RPMI Medium 1640 supplemented with 5% fetal bovine serum (FBS; GIBCO, Invitrogen, Carlsbad, CA) in a 37°C water-jacketed incubator (Forma Scientific, Marietta, OH) with 5% CO 2 . Trypsin-ethylenediamine tetraacetic acid (Sigma Chemical Co, St. Louis, MO) was used for harvesting and for the subculturing of cells.
Laeverin antibodies
Polyclonal antibodies against laeverin were raised (Eurogentec, Seraing, Belgium). Rabbits were immunized with synthetic oligo-peptides that contained 2 predicted epitopes (EP073418:CRVHANLQTIKNENLK and EP073419:CERAEVRGPLSPGTG). Peptide sequences for these epitopes were chosen from the amino acid sequence of laeverin (Q0P5U8; http://www.ncbi.nlm.nih.gov/protein/121946569 ). Immunogenic epitopes of the exposed amino acids of the laeverin 3-dimensional structure were chosen for peptide synthesis with software that was provided by Sigma Chemical Co.
A commercially available goat polyclonal antibody of laeverin was used as control (Santa Cruz Biotechnology Inc, Santa Cruz, CA).
Immunofluorescence
Tissue samples from 3 preeclamptic placentas and 3 normal healthy controls were fixed in formalin, embedded in paraffin blocks, cut (4-6 μ sections), and mounted on glass slides. Immunofluorescence cell staining was performed with our laeverin antibody (2.1 μg/mL) and secondary goat anti-rabbit immunoglobulin G- fluorescein isothiocyanate (2.5 μg/mL; Santa Cruz Biotechnology Inc). Slides were counterstained with DAPI (4′,6-diamidino-2-phenylindole) II (Vysis; Abbott Diagnostics, Lake Forest, IL). Images were obtained with CytoVision digital system (Applied Imaging, Grand Rapids, MI) that was equipped with a charge-coupled device camera (Cohu Inc, Poway, CA). A total of ≥200 cells were inspected on each slide. Experiments were run in triplicate.
Protein isolation
Placental tissue was cut in small pieces, and proteins were isolated with the use of T-PER (Pierce Chemical Co, Rockford, IL) with Complete Mini ethylenediamine tetraacetic acid–free protease inhibitor cocktail in combination MagNA Lyser Green Beads for homogenizing on MagNA Lyser (Roche, Indianapolis, IN). Protein concentration was measured with the use of the DC Protein Assay kit (Bio-Rad Laboratories, Hercules, CA) in a ThermoMax Microplate Reader (Molecular Devices, Downington, PA).
Sodium dodecylsulfate-polyacrylamide gel electrophoresis and Western blot analysis
Reduced and denatured proteins (5 μg) that had been isolated from 8 placentas (4 preeclamptic and 4 normal) were separated by sodium dodecyl sulfate-polyacrylamide gel electrophoresis (SDS-PAGE) on 4-12% NuPAGE (Invitrogen). Electrophoresis and blotting (polyvinylidene difluoride nylon membrane, pore size 0.45 μm; Invitrogen) were run on Novex Mini Cell XCell Sure Lock (Invitrogen). Blots were cut under a 49-kDa protein band to provide 2 blots; 1 for laeverin and another for the housekeeping protein actin. Labeling was done with primary antibodies against laeverin (our antibody [0.42μg/ml] and commercial antibody [1 μg/mL; Santa Cruz Biotechnology Inc] or actin [1 μg/mL; Santa Cruz Biotechnology Inc]). Detection was performed with goat anti-mouse immunoglobulin G-alkaline phosphatase–conjugated antibody (0.2 μg/mL; Santa Cruz Biotechnology Inc) and CDP-Star (Roche). Pictures were taken on ImageQuant LAS 4000 (GE Healthcare Bio-Sciences AB, Uppsala, Sweden). Experiments were run in triplicate.
Immunoelectron microscopy
Immunoelectron microscopy was performed on ultrathin tissue sections of 2 healthy placentas and 2 placentas that were obtained from women with severe preeclampsia. All experiments were run in triplicates.
Fresh placental tissue samples were dissected, mounted in membrane carriers, and frozen at high pressure (EMPACT 2 HPF; Leica Microsystems, Vienna, Austria). Frozen samples freeze substituted (EM AFS2, Leica Microsystems) and infiltrated in Lowicryl HM20 (Electron Microscopy Sciences, Hatfield, UK). Ultrathin sections of 70 nm were cut on a Leica EM UC6 ultramicrotome (Leica Microsystems) and mounted on copper grids (Agar Scientific, Stansted, UK) with Formwar and carbon.
Immunolabeling was performed with the optimal dilution of primary antibodies. Single and double labeling experiments were performed with both locally designed (26.25 μg/mL) and commercially purchased laeverin (5 μg/mL) antibodies. For double labeling, anti-endoplasmatic reticulum (ER) mouse monoclonal antibody (RL90) to protein disulphide isomerase (ab2792; 0.1 μg/mL) and anti-Golgi apparatus (GA) mouse monoclonal antibody (AE-6) to MG160 protein (MG160; ab58826; 0.05 μg/mL; Abcam, Cambridge, UK) were used as the specific markers of ER and GA, respectively.
Microscopy was done with a JEM-1010 transmission electron microscope (JEOL, Tokyo, Japan) at 4000, 10,000, 20,000, 30,000, and 70,000 magnifications. Images were taken and processed in Morada Soft Imaging Camera system with iTEM software (Olympus, Hamburg, Germany). A total of 200 images from each experiment were processed. Image montage was done in Adobe Photoshop and Adobe Illustrator (Adobe Systems Inc, San Jose, CA).
Immunoglobulin G conjugated gold particles were used as controls in similar experiments. Possible secondary antibody cross-reactivity was excluded by the omission of primary antibodies in separate experiments.
xCelligence migration assay
HTR-8/SVneo trophoblast cells (2 × 10 5 cells/well) were seeded the day before small interfering RNA (siRNA) transfection with FuGENE transfection reagent (Promega Corp, Madison, WI). SiRNA (10 pmol) against laeverin or scrambled siRNA A or D (control; Santa Cruz Biotechnology Inc) were used. Plates were incubated at 37°C with 5% CO 2 for 5 hours; transfection solution was replaced with fresh RPMI Medium 1640 with 5% FBS, and cells were further incubated for 24 hours; 2 × 10 5 cells/well were added to each well of the CIM-Plate 16 (ACEA Biosciences Inc, San Diego, CA). Migration assays were performed (for 72 hours, with sweeps of 30 minutes each) in the xCelligence system (ACEA Biosciences Inc). Three different CIM-Plates 16 were used. Experiments were run in quadruplicate. Coefficients of variation for siRNA A, D, and laeverin were 3.5%, 1.2%, and 3.5%, respectively. Untransfected cells were used as controls and were run in duplicates on each plate. Analysis was performed in the RTCA software (version 1.2.1; ACEA Biosciences Inc).
Reverse transcription–PCR of the cell line that was used in migration assays
Total RNA was isolated from cultured cells (untransfected, siRNA silenced laeverin siRNA A and D silenced) with TRIzol Reagent (Invitrogen) 55 hours after transfection at the migration optimum. Total RNA was extracted with RNeasy Mini Kit (Qiagen, Venio, The Netherlands); the concentration of RNA was measured with NanoDrop (Saveen Werner, Malmo, Sweden), and reverse transcription was performed with the High Capacity RNA-to-cDNA Kit (Applied Biosystems, Foster City, CA). Complementary DNA samples were profiled for the relative expression of the genes of laeverin, glyceraldehyde-phosphate dehydrogenase, and actin, beta with the Taq Man Gene Expression Assays on 7900HT Fast Real-Time PCR system (Applied Biosystems).
Matrigel invasion studies in Boyden chambers
HTR-8/SVneo trophoblast cells (5 × 10 5 cells) were grown in RPMI Medium 1640 with 10% FBS and incubated at 37°C, with 5% CO 2 overnight. Medium was replaced by RPMI Medium 1640 with 5% FBS the next day. On day 3, transfection with 50 pmol laeverin siRNA or 50 pmol of siRNA A control (Santa Cruz Biotechnology Inc) with Lipofectamine 2000 (Invitrogen) was performed in separate flasks. Cultures were incubated at 37°C with 5% CO 2 for 4 hours and washed with RPMI Medium 1640 without serum before incubation overnight. Invasion studies (1 × 10 5 cells/well; 5% FBS used as chemoattractant) were performed in 48 hours in BD BioCoat–BD Matrigel Invasion Chambers (24-well plate 8 μ with control inserts; BD Biosciences, San Jose, CA) and incubated at 37°C with 5% CO 2 . The noninvading cells were removed from the upper part of the insert’s membrane by scrubbing with cotton-tipped swabs that had been moistened with medium. Cell invasion was performed by methylthiazolyldiphenyl-tetrazolium bromide (MTT)-assay. Results were monitored in Thermo Multiscan Ex (ThermoFisher Scientific Inc, Waltham, MA). Experiments were run in triplicate.
Gene expression profiling
To investigate the downstream effect of laeverin-gene silencing in HTR-8/SVneo trophoblast cells, we performed a PCR array to explore 6 biologic pathways that are involved in cell transformation and tumorigenesis ( Appendix ; Supplemental Table 1 ).
HTR-8/SVneo trophoblast cells (4-5,7 × 10 5 cells) were transfected with Lipofectamine 2000 and 120 pmol siRNA laeverin or siRNA A (control). Cells were mixed with TRIzol Reagent and RNA isolated by RNeasy Mini kit. Complementary DNA synthesis and quantitative reverse transcription–PCR were performed with the use of RT Profiler PCR Array Human Cancer Pathway Finder (PAHS-033A; SABiosciences Corporation, Frederick, MD). Actin, beta was used as housekeeping gene. Analysis of fold changes was done by the comparative Ct (ΔΔCt) method with the integrated web-based software package for the PCR array system.
Statistical analysis
Data were analyzed with IBM SPSS Statistics 21 software (SPSS Inc, Chicago, IL). Continuous variables are presented as mean ± SE or median (range); categoric variables are presented as number (%). Assessment of normality was performed with the Shapiro-Wilk test. Differences between groups were tested with the Student t test for parametric variables and the Mann-Whitney U test, χ 2 test, or F-test for nonparametric variables. A probability value of < .05 was considered significant.
Results
Phenotype of the study population
The baseline demographic and clinical characteristics of the study population that included birth outcomes are shown in the Table . The mean proteinuria level was 5.9 g/L (range, 3.9–9.0 g/L) in women with preeclampsia. None of the women who were included in the study had HELLP (hemolysis, elevated liver enzymes and low-platelets) syndrome. Two women in the preeclampsia group were delivered by cesarean section because of worsening condition. Two women in the normal group also had cesarean deliveries; one because of breech presentation and another because of placenta previa. None of them were in labor. Four women in each group had vaginal delivery; 3 women in each group had induced labor.
Variable | Preeclampsia (n = 6) | Health control subjects (n = 6) | P value |
---|---|---|---|
Maternal age, y a | 28 ± 2.35 | 32 ± 1.58 | .261 |
Body mass index before delivery, kg/m 2 a | 28.9 ± 0.85 | 29.6 ± 1.85 | .873 |
Primiparous, n (%) | 4 (66.7) | 2 (33.3) | .567 |
Mean arterial pressure, mm Hg a | 131 ± 3.82 | 85 ± 4.15 | < .0001 |
24-hour proteinuria, g/L a | 5.92 ± 5.10 | N/A | |
Uterine artery pulsatility index (mean of the left and right side) a | 1.23 ± 0.29 | 0.69 ± 0.9 | .157 |
Middle cerebral artery pulsatility index a | 1.20 ± 0.14 | 1.38 ± 0.12 | .142 |
Umbilical artery pulsatility index a | 1.19 ± 0.15 | 0.81 ± 0.13 | .049 |
Gestational age at delivery, wk a | 34 ± 1.4 | 39 ± 0.48 | .005 |
Cesarean delivery, n (%) | 2 (33.3) | 2 (33.3) | 1 |
Neonatal birthweight, g a | 2390 ± 430 | 3328 ± 207 | .055 |
Placental weight, g a | 437 ± 72 | 623 ± 69 | .065 |
5-minute Apgar score b | 8 (6–9) | 10 (10–10) | .002 |
Arterial cord blood pH a | 7.27 ± 0.02 | 7.25 ± 0.03 | 1.0 |
Arterial cord blood base excess, mmol/L a | 1.97 ± 2.06 | 7.67 ± 0.58 | .069 |
Venous cord blood pH a | 7.33 ± 0.02 | 7.35 ± 0.02 | .343 |
Venous cord blood base excess, mmol/L a | −2.12 ± 1.66 | −4.50 ± 0.84 | .343 |
Laeverin ectopically expressed in the trophoblastic cytoplasm in preeclampsia
Immunofluorescence analysis demonstrated that laeverin is expressed by the villous trophoblasts ( Figure 1 ). In normal placenta, it was membrane-bound and mainly expressed in plasma membrane ( Figure 1 , A). Laeverin was expressed more abundantly in the preeclamptic placenta and was localized in the cytoplasm of the villous trophoblast cells ( Figure 1 , B). In control placentas in which laeverin was replaced by phosphate-buffered saline solution, no specific labeling was detected in the villous trophoblasts ( Figure 1 , C).

Molecular mass of laeverin
We estimated the molecular mass of laeverin to be approximately 60 kDa by performing denaturing and reducing SDS-PAGE and Western blot analysis of healthy and preeclamptic placentas ( Figure 2 ). Experiments with our locally designed antibody that was raised against the N- and C-terminal part of laeverin and commercially available antibody that was raised against a peptide mapping within an internal region of laeverin gave the same results.

Laeverin in preeclamptic placentas
Immunoelectron microscopy demonstrated that laeverin was expressed in the plasma membrane of trophoblast cells of healthy placentas. It was hardly detectable in the cytosol and was not detectable in the fetal capillaries ( Figure 3 , B). However, in preeclamptic placentas, laeverin was expressed strongly in the cytoplasm, especially in the microvesicles and in the fetal capillaries ( Figure 3 , A, C, E, and G). Laeverin was expressed abundantly in microvesicles within the cytoplasm, in the extracellular space, and in areas of focal aggregation of syncytiotrophoblasts (syncytial knots). Laeverin was not expressed in mitochondria but was expressed in ER and GA. Experiments with the use of the commercially available laeverin antibody gave same results ( Figure 4 ).


Laeverin silencing affects trophoblast cell migration
Transfection with siRNA against laeverin showed an 11.5% ( P = .023) reduction in the migration of HTR-8/SVneo trophoblast cells compared with cells that were transfected with scrambled siRNA (control) at the peak of migration, approximately 30 hours after transfection ( Figure 5 , A; Supplemental Table 5 ). Laeverin messenger RNA (mRNA) was 36% reduced in silenced cells ( Figure 5 , B).

Trophoblast invasion reduced in laeverin-silenced HTR-8/SVneo cells
We found that the absorbance (optical density 540) of laeverin-silenced cells was 0.16 ± 0.07 and that absorbance at 540 nm of control cells was 0.3 ± 0.11 ( P = .001). Thus, laeverin-silenced cells had a 56.7% reduced ability to invade through Matrigel, compared with control cells ( Figure 6 ). Laeverin mRNA was 80% reduced in silenced cells (data not shown).

Integrin alpha-2, matrix metalloproteinase 1, and integrin beta-3 genes down-regulated in laeverin-silenced HTR-8/SVneo cells
Three genes that are involved in cell transformation and tumorigenesis were shown to be down-regulated by laeverin-silencing at a significant level (>4-fold) with the PCR array. Integrin alpha-2, matrix metalloproteinase 1 (MMP1), and integrin beta-3 were down-regulated 39-fold, 36-fold, and 5-fold, respectively ( Figure 7 ). The complete list of genes on the array together with reverse transcription–PCR results (average threshold cycle [Ct], average difference in cycle number [ΔCt], and fold-regulation) are given in Supplemental Tables 2-4 .

Results
Phenotype of the study population
The baseline demographic and clinical characteristics of the study population that included birth outcomes are shown in the Table . The mean proteinuria level was 5.9 g/L (range, 3.9–9.0 g/L) in women with preeclampsia. None of the women who were included in the study had HELLP (hemolysis, elevated liver enzymes and low-platelets) syndrome. Two women in the preeclampsia group were delivered by cesarean section because of worsening condition. Two women in the normal group also had cesarean deliveries; one because of breech presentation and another because of placenta previa. None of them were in labor. Four women in each group had vaginal delivery; 3 women in each group had induced labor.
Variable | Preeclampsia (n = 6) | Health control subjects (n = 6) | P value |
---|---|---|---|
Maternal age, y a | 28 ± 2.35 | 32 ± 1.58 | .261 |
Body mass index before delivery, kg/m 2 a | 28.9 ± 0.85 | 29.6 ± 1.85 | .873 |
Primiparous, n (%) | 4 (66.7) | 2 (33.3) | .567 |
Mean arterial pressure, mm Hg a | 131 ± 3.82 | 85 ± 4.15 | < .0001 |
24-hour proteinuria, g/L a | 5.92 ± 5.10 | N/A | |
Uterine artery pulsatility index (mean of the left and right side) a | 1.23 ± 0.29 | 0.69 ± 0.9 | .157 |
Middle cerebral artery pulsatility index a | 1.20 ± 0.14 | 1.38 ± 0.12 | .142 |
Umbilical artery pulsatility index a | 1.19 ± 0.15 | 0.81 ± 0.13 | .049 |
Gestational age at delivery, wk a | 34 ± 1.4 | 39 ± 0.48 | .005 |
Cesarean delivery, n (%) | 2 (33.3) | 2 (33.3) | 1 |
Neonatal birthweight, g a | 2390 ± 430 | 3328 ± 207 | .055 |
Placental weight, g a | 437 ± 72 | 623 ± 69 | .065 |
5-minute Apgar score b | 8 (6–9) | 10 (10–10) | .002 |
Arterial cord blood pH a | 7.27 ± 0.02 | 7.25 ± 0.03 | 1.0 |
Arterial cord blood base excess, mmol/L a | 1.97 ± 2.06 | 7.67 ± 0.58 | .069 |
Venous cord blood pH a | 7.33 ± 0.02 | 7.35 ± 0.02 | .343 |
Venous cord blood base excess, mmol/L a | −2.12 ± 1.66 | −4.50 ± 0.84 | .343 |
Laeverin ectopically expressed in the trophoblastic cytoplasm in preeclampsia
Immunofluorescence analysis demonstrated that laeverin is expressed by the villous trophoblasts ( Figure 1 ). In normal placenta, it was membrane-bound and mainly expressed in plasma membrane ( Figure 1 , A). Laeverin was expressed more abundantly in the preeclamptic placenta and was localized in the cytoplasm of the villous trophoblast cells ( Figure 1 , B). In control placentas in which laeverin was replaced by phosphate-buffered saline solution, no specific labeling was detected in the villous trophoblasts ( Figure 1 , C).
Molecular mass of laeverin
We estimated the molecular mass of laeverin to be approximately 60 kDa by performing denaturing and reducing SDS-PAGE and Western blot analysis of healthy and preeclamptic placentas ( Figure 2 ). Experiments with our locally designed antibody that was raised against the N- and C-terminal part of laeverin and commercially available antibody that was raised against a peptide mapping within an internal region of laeverin gave the same results.
Laeverin in preeclamptic placentas
Immunoelectron microscopy demonstrated that laeverin was expressed in the plasma membrane of trophoblast cells of healthy placentas. It was hardly detectable in the cytosol and was not detectable in the fetal capillaries ( Figure 3 , B). However, in preeclamptic placentas, laeverin was expressed strongly in the cytoplasm, especially in the microvesicles and in the fetal capillaries ( Figure 3 , A, C, E, and G). Laeverin was expressed abundantly in microvesicles within the cytoplasm, in the extracellular space, and in areas of focal aggregation of syncytiotrophoblasts (syncytial knots). Laeverin was not expressed in mitochondria but was expressed in ER and GA. Experiments with the use of the commercially available laeverin antibody gave same results ( Figure 4 ).
Laeverin silencing affects trophoblast cell migration
Transfection with siRNA against laeverin showed an 11.5% ( P = .023) reduction in the migration of HTR-8/SVneo trophoblast cells compared with cells that were transfected with scrambled siRNA (control) at the peak of migration, approximately 30 hours after transfection ( Figure 5 , A; Supplemental Table 5 ). Laeverin messenger RNA (mRNA) was 36% reduced in silenced cells ( Figure 5 , B).
Trophoblast invasion reduced in laeverin-silenced HTR-8/SVneo cells
We found that the absorbance (optical density 540) of laeverin-silenced cells was 0.16 ± 0.07 and that absorbance at 540 nm of control cells was 0.3 ± 0.11 ( P = .001). Thus, laeverin-silenced cells had a 56.7% reduced ability to invade through Matrigel, compared with control cells ( Figure 6 ). Laeverin mRNA was 80% reduced in silenced cells (data not shown).
Integrin alpha-2, matrix metalloproteinase 1, and integrin beta-3 genes down-regulated in laeverin-silenced HTR-8/SVneo cells
Three genes that are involved in cell transformation and tumorigenesis were shown to be down-regulated by laeverin-silencing at a significant level (>4-fold) with the PCR array. Integrin alpha-2, matrix metalloproteinase 1 (MMP1), and integrin beta-3 were down-regulated 39-fold, 36-fold, and 5-fold, respectively ( Figure 7 ). The complete list of genes on the array together with reverse transcription–PCR results (average threshold cycle [Ct], average difference in cycle number [ΔCt], and fold-regulation) are given in Supplemental Tables 2-4 .
Comment
Laeverin was first reported to be expressed in the cell surface of extravillous trophoblasts obtained from human third-trimester chorion laeve. Northern blot analysis showed that laeverin is a placenta-specific protein. It contains a transmembrane domain at the N-terminus and has an amino acid sequence that is homologous with membrane-bound aminopeptidase-N. However, the function of laeverin still is not understood completely. Our immunofluorescence studies on healthy placental tissues demonstrated that laeverin is expressed in the plasma membrane of trophoblast cells, which confirms previous findings. However, in preeclamptic placentas, it was localized mainly in the cytoplasm, especially the microvesicles. To our knowledge, this has not been reported previously.
Protein modifications or cleavage of laeverin could be responsible for its altered placental expression in preeclampsia. However, results of SDS-PAGE and Western blot analysis clearly indicate that laeverin has a molecular mass of 60 kDa ( Figure 2 ) both in preeclamptic and normal placenta. The predicted molecular mass from the amino acid sequence is 113 kDa. However, this variance can be explained. Native laeverin might have cleaved during the purification that resulted in 2 identical proteins of 60 kDa, with a total mass of 120 kDa. Furthermore, alternate splicing of the laeverin gene may produce 4 protein isoforms with different molecular masses. Horie et al detected 3 different bands of 200-270 kDa, 160 kDa, and 130 kDa in normal placenta instead of 1. This discrepancy could be due to the differences in antibodies and methods that were used for protein purification and analysis.
Immunoelectron microscopy demonstrated the expression of laeverin in the fetal capillaries and in the microvesicles within the cytosol of trophoblast cells in preeclamptic placentas. Microvesicles in the extracellular space and syncytial knots also expressed laeverin abundantly. Microvesicles and exosomes have been found previously in preeclamptic placentas, and syncytiotrophoblast vesicles have been shown to play a role in the pathophysiologic condition of preeclampsia. Trophoblastic microvesicles can also be found in the maternal circulation and release cytokines that provoke maternal inflammatory response. Microvesicles contain fetal DNA, RNA, and proteins and play an important role in cell communication. They facilitate intracellular transport of proteins and their attachment to the plasma membrane at specific sites. Colocalization of laeverin together with ER and GA markers indicated aberrant processing of laeverin in preeclamptic placentas that may have resulted in massive production of microvesicles. Because the ER and GA in normal placentas did not show accumulation of laeverin, conventional exocytosis might be impaired in preeclamptic placenta.
The laeverin enzyme appears to have a broad spectrum of substrates that can affect cell migration and angiogenesis. We found that laeverin silencing reduces migration and invasion of HTR-8/SVneo trophoblast cells. In line with this, Horie et al have also demonstrated reduced cell invasion in laeverin-silenced human chorionic villous explants cultures.
It has been hypothesized that laeverin plays a role in extravillous trophoblast invasion in cooperation with the chemokine system in the fetomaternal interface. Kisspeptin, angiotensin III, edokinin C, and dynorphin A1-8 are the only known proteins that can be cleaved by laeverin. We looked for other possible interaction partners by performing PCR array on 84 selected genes that are representative of the 6 biologic pathways that are involved in cell transformation and tumorigenesis. Silencing laeverin had downstream effects on the regulation of the cell adhesion system that is mediated by MMP1 and integrins. MMP1 is expressed by invasive trophoblasts in the first-, second-, and third-trimester placentas. MMPs are involved in changing cell phenotype from adhesive to a migratory by degrading the extracellular matrix (ECM). They affect cell migration during physiologic processes (such as embryonic development, reproduction and tissue remodeling) and in pathologic conditions (such as cancer metastasis). Integrins are the main receptors for the ECM and are involved in regulating cell adhesion and locomotion. Indeed, trophoblast interaction with the ECM has been shown to be mediated by integrins and MMPs. Therefore, it is plausible that laeverin-silenced trophoblasts lose their invasiveness by interacting with the cell’s integrin and MMP repertoire.
The molecular link between reduced trophoblast invasion of maternal decidua in the first trimester and the development of preeclampsia later in pregnancy is still missing. We have shown previously that laeverin mRNA is increased in preeclamptic placentas. Our present study shows that laeverin is also increased at protein level and that laeverin silencing reduces trophoblast cell migration and invasion in vitro. Moreover, it has been shown that endogenous laeverin on the surface of isolated extravillous trophoblastic cells acts to promote their invasion capacity. We found that, in preeclampsia, laeverin is not bound to the trophoblast cell membrane, which indicates a possible deregulation of its physiologic function. Consequently, one can hypothesize that the production of a deregulated, malfunctioning protein in the preeclamptic placenta might lead to a compensatory increase of laeverin at the mRNA level.
A limitation of our study is that the preeclamptic placentas were delivered earlier compared with the control placentas. However, because term placentas express higher levels of laeverin compared with early (first-trimester) placentas, one would expect to see lower levels of laeverin in preeclamptic placentas that were delivered preterm. Therefore, the observed differences in laeverin expression are likely to be real and suggest that the overexpression of laeverin in preeclampsia is associated with the disease process rather than the differences in gestational age. Another limitation of the study is the small number of placental samples that were used. However, experiments were run in triplicate, and the results were reproducible.
In summary, laeverin, a placenta-specific protein, appears to be deregulated in preeclampsia that leads to its overexpression and altered subcellular localization in the villous trophoblast. Whether it could be used potentially as a biomarker of abnormal placentation for prediction and diagnosis of preeclampsia needs further investigation. We are studying longitudinal changes in laeverin levels in maternal circulation during normal pregnancy and assessing whether first- and second-trimester serum laeverin concentration can be used to improve the prediction of preeclampsia in an unselected population.
Appendix
PCR array catalog #: | Unigene | PAHS-033 | Symbol | Description | Gname | RT2 Catalog |
---|---|---|---|---|---|---|
Position | Refseq | |||||
A01 | Hs.525622 | NM_005163 | AKT1 | V-akt murine thymoma viral oncogene homolog 1 | AKT/PKB | PPH00088A |
A02 | Hs.369675 | NM_001146 | ANGPT1 | Angiopoietin 1 | AGP1/AGPT | PPH00374A |
A03 | Hs.583870 | NM_001147 | ANGPT2 | Angiopoietin 2 | AGPT2/ANG2 | PPH00377E |
A04 | Hs.552567 | NM_001160 | APAF1 | Apoptotic peptidase activating factor 1 | APAF-1/CED4 | PPH00752A |
A05 | Hs.367437 | NM_000051 | ATM | Ataxia telangiectasia mutated | AT1/ATA | PPH00325B |
A06 | Hs.370254 | NM_004322 | BAD | BCL2-associated agonist of cell death | BBC2/BCL2L8 | PPH00075B |
A07 | Hs.624291 | NM_004324 | BAX | BCL2-associated X protein | BCL2L4 | PPH00078B |
A08 | Hs.150749 | NM_000633 | BCL2 | B-cell CLL/lymphoma 2 | Bcl-2 | PPH00079B |
A09 | Hs.516966 | NM_138578 | BCL2L1 | BCL2-like 1 | BCL-XL/S | PPH00082B |
A10 | Hs.194143 | NM_007294 | BRCA1 | Breast cancer 1, early onset | BRCAI/BRCC1 | PPH00322E |
A11 | Hs.599762 | NM_001228 | CASP8 | Caspase 8, apoptosis-related cysteine peptidase | ALPS2B/CAP4 | PPH00359E |
A12 | Hs.244723 | NM_001238 | CCNE1 | Cyclin E1 | CCNE | PPH00131A |
B01 | Hs.437705 | NM_001789 | CDC25A | Cell division cycle 25 homolog A (S. pombe) | CDC25A2 | PPH00930A |
B02 | Hs.19192 | NM_001798 | CDK2 | Cyclin-dependent kinase 2 | p33(CDK2) | PPH00117E |
B03 | Hs.95577 | NM_000075 | CDK4 | Cyclin-dependent kinase 4 | CMM3/PSK-J3 | PPH00118E |
B04 | Hs.370771 | NM_000389 | CDKN1A | Cyclin-dependent kinase inhibitor 1A (p21, Cip1) | CAP20/CDKN1 | PPH00211E |
B05 | Hs.512599 | NM_000077 | CDKN2A | Cyclin-dependent kinase inhibitor 2A (melanoma, p16, inhibits CDK4) | ARF/CDK4I | PPH00207B |
B06 | Hs.390736 | NM_003879 | CFLAR | CASP8 and FADD-like apoptosis regulator | CASH/CASP8AP1 | PPH00333A |
B07 | Hs.291363 | NM_007194 | CHEK2 | CHK2 checkpoint homolog (S. pombe) | CDS1/CHK2 | PPH00921B |
B08 | Hs.517356 | NM_030582 | COL18A1 | Collagen, type XVIII, alpha 1 | KNO/KNO1 | PPH01141E |
B09 | Hs.654393 | NM_005225 | E2F1 | E2F transcription factor 1 | E2F-1/RBAP1 | PPH00136F |
B10 | Hs.446352 | NM_004448 | ERBB2 | V-erb-b2 erythroblastic leukemia viral oncogene homolog 2, neuro/glioblastoma derived oncogene homolog (avian) | CD340/HER-2 | PPH00209B |
B11 | Hs.644231 | NM_005239 | ETS2 | V-Ets erythroblastosis virus E26 oncogene homolog 2 (avian) | ETS2IT1 | PPH00091B |
B12 | Hs.244139 | NM_000043 | FAS | Fas (TNF receptor superfamily, member 6) | ALPS1A/APO-1 | PPH00141B |
C01 | Hs.533683 | NM_000141 | FGFR2 | Fibroblast growth factor receptor 2 | BEK/BFR-1 | PPH00391E |
C02 | Hs.25647 | NM_005252 | FOS | V-fos FBJ murine osteosarcoma viral oncogene homolog | AP-1/C-FOS | PPH00094A |
C03 | Hs.90708 | NM_006144 | GZMA | Granzyme A (granzyme 1, cytotoxic T-lymphocyte-associated serine esterase 3) | CTLA3/HFSP | PPH00314E |
C04 | Hs.90753 | NM_006410 | HTATIP2 | HIV-1 Tat interactive protein 2, 30kDa | CC3/SDR44U1 | PPH06957A |
C05 | Hs.37026 | NM_024013 | IFNA1 | Interferon, alpha 1 | IFL/IFN | PPH01321A |
C06 | Hs.93177 | NM_002176 | IFNB1 | Interferon, beta 1, fibroblast | IFB/IFF | PPH00384E |
C07 | Hs.160562 | NM_000618 | IGF1 | Insulin-like growth factor 1 (somatomedin C) | IGF1A/IGFI | PPH00167B |
C08 | Hs.624 | NM_000584 | IL8 | Interleukin 8 | CXCL8/GCP-1 | PPH00568A |
C09 | Hs.644352 | NM_181501 | ITGA1 | Integrin, alpha 1 | CD49a/VLA1 | PPH00627B |
C10 | Hs.482077 | NM_002203 | ITGA2 | Integrin, alpha 2 (CD49B, alpha 2 subunit of VLA-2 receptor) | BR/CD49B | PPH00625E |
C11 | Hs.265829 | NM_002204 | ITGA3 | Integrin, alpha 3 (antigen CD49C, alpha 3 subunit of VLA-3 receptor) | CD49C/GAP-B3 | PPH00175A |
C12 | Hs.694732 | NM_000885 | ITGA4 | Integrin, alpha 4 (antigen CD49D, alpha 4 subunit of VLA-4 receptor) | CD49D/IA4 | PPH00659E |
D01 | Hs.436873 | NM_002210 | ITGAV | Integrin, alpha V (vitronectin receptor, alpha polypeptide, antigen CD51) | CD51/DKFZp686A08142 | PPH00628B |
D02 | Hs.643813 | NM_002211 | ITGB1 | Integrin, beta 1 (fibronectin receptor, beta polypeptide, antigen CD29 includes MDF2, MSK12) | CD29/FNRB | PPH00650B |
D03 | Hs.218040 | NM_000212 | ITGB3 | Integrin, beta 3 (platelet glycoprotein IIIa, antigen CD61) | CD61/GP3A | PPH00178C |
D04 | Hs.536663 | NM_002213 | ITGB5 | Integrin, beta 5 | FLJ26658 | PPH00634E |
D05 | Hs.714791 | NM_002228 | JUN | Jun oncogene | AP-1/AP1 | PPH00095A |
D06 | Hs.145442 | NM_002755 | MAP2K1 | Mitogen-activated protein kinase kinase 1 | MAPKK1/MEK1 | PPH00711B |
D07 | Hs.599039 | NM_006500 | MCAM | Melanoma cell adhesion molecule | CD146/MUC18 | PPH00651A |
D08 | Hs.484551 | NM_002392 | MDM2 | Mdm2 p53 binding protein homolog (mouse) | HDMX/hdm2 | PPH00193E |
D09 | Hs.132966 | NM_000245 | MET | Met proto-oncogene (hepatocyte growth factor receptor) | AUTS9/HGFR | PPH00194A |
D10 | Hs.83169 | NM_002421 | MMP1 | Matrix metallopeptidase 1 (interstitial collagenase) | CLG/CLGN | PPH00120B |
D11 | Hs.513617 | NM_004530 | MMP2 | Matrix metallopeptidase 2 (gelatinase A, 72kDa gelatinase, 72kDa type IV collagenase) | CLG4/CLG4A | PPH00151B |
D12 | Hs.297413 | NM_004994 | MMP9 | Matrix metallopeptidase 9 (gelatinase B, 92kDa gelatinase, 92kDa type IV collagenase) | CLG4B/GELB | PPH00152E |
E01 | Hs.525629 | NM_004689 | MTA1 | Metastasis associated 1 | Mta-1 | PPH01083E |
E02 | Hs.173043 | NM_004739 | MTA2 | Metastasis associated 1 family, member 2 | DKFZp686F2281/MTA1L1 | PPH13564A |
E03 | Hs.700429 | NM_014751 | MTSS1 | Metastasis suppressor 1 | DKFZp781P2223/MIM | PPH10073A |
E04 | Hs.202453 | NM_002467 | MYC | V-myc myelocytomatosis viral oncogene homolog (avian) | MRTL/bHLHe39 | PPH00100A |
E05 | Hs.654408 | NM_003998 | NFKB1 | Nuclear factor of kappa light polypeptide gene enhancer in B-cells 1 | DKFZp686C01211/EBP-1 | PPH00204E |
E06 | Hs.81328 | NM_020529 | NFKBIA | Nuclear factor of kappa light polypeptide gene enhancer in B-cells inhibitor, alpha | IKBA/MAD-3 | PPH00170E |
E07 | Hs.118638 | NM_000269 | NME1 | Non-metastatic cells 1, protein (NM23A) expressed in | AWD/GAAD | PPH01314A |
E08 | Hs.9235 | NM_005009 | NME4 | Non-metastatic cells 4, protein expressed in | NDPK-D/NM23H4 | PPH01086A |
E09 | Hs.535898 | NM_002607 | PDGFA | Platelet-derived growth factor alpha polypeptide | PDGF-A/PDGF1 | PPH00217B |
E10 | Hs.1976 | NM_002608 | PDGFB | Platelet-derived growth factor beta polypeptide (simian sarcoma viral (v-sis) oncogene homolog) | PDGF2/SIS | PPH00488E |
E11 | Hs.132225 | NM_181504 | PIK3R1 | Phosphoinositide-3-kinase, regulatory subunit 1 (alpha) | GRB1/p85 | PPH00713E |
E12 | Hs.77274 | NM_002658 | PLAU | Plasminogen activator, urokinase | ATF/UPA | PPH00796B |
F01 | Hs.466871 | NM_002659 | PLAUR | Plasminogen activator, urokinase receptor | CD87/UPAR | PPH00797B |
F02 | Hs.409965 | NM_002687 | PNN | Pinin, desmosome associated protein | DRS/SDK3 | PPH19485E |
F03 | Hs.159130 | NM_002880 | RAF1 | V-raf-1 murine leukemia viral oncogene homolog 1 | CRAF/NS5 | PPH00227E |
F04 | Hs.408528 | NM_000321 | RB1 | Retinoblastoma 1 | OSRC/RB | PPH00228E |
F05 | Hs.654444 | NM_002961 | S100A4 | S100 calcium binding protein A4 | 18A2/42A | PPH01313E |
F06 | Hs.55279 | NM_002639 | SERPINB5 | Serpin peptidase inhibitor, clade B (ovalbumin), member 5 | PI5/maspin | PPH00695E |
F07 | Hs.414795 | NM_000602 | SERPINE1 | Serpin peptidase inhibitor, clade E (nexin, plasminogen activator inhibitor type 1), member 1 | PAI/PAI-1 | PPH00215E |
F08 | Hs.349470 | NM_003087 | SNCG | Synuclein, gamma (breast cancer-specific protein 1) | BCSG1/SR | PPH01051E |
F09 | Hs.371720 | NM_003177 | SYK | Spleen tyrosine kinase | DKFZp313N1010 | PPH01639E |
F10 | Hs.89640 | NM_000459 | TEK | TEK tyrosine kinase, endothelial | CD202B/TIE-2 | PPH00795B |
F11 | Hs.492203 | NM_198253 | TERT | Telomerase reverse transcriptase | EST2/TCS1 | PPH00995E |
F12 | Hs.645227 | NM_000660 | TGFB1 | Transforming growth factor, beta 1 | CED/DPD1 | PPH00508A |
G01 | Hs.494622 | NM_004612 | TGFBR1 | Transforming growth factor, beta receptor 1 | AAT5/ACVRLK4 | PPH00237B |
G02 | Hs.164226 | NM_003246 | THBS1 | Thrombospondin 1 | THBS/THBS-1 | PPH00799E |
G03 | Hs.522632 | NM_003254 | TIMP1 | TIMP metallopeptidase inhibitor 1 | CLGI/EPA | PPH00771B |
G04 | Hs.644633 | NM_000362 | TIMP3 | TIMP metallopeptidase inhibitor 3 | HSMRK222/K222 | PPH00762A |
G05 | Hs.241570 | NM_000594 | TNF | Tumor necrosis factor (TNF superfamily, member 2) | DIF/TNF-alpha | PPH00341E |
G06 | Hs.521456 | NM_003842 | TNFRSF10B | Tumor necrosis factor receptor superfamily, member 10b | CD262/DR5 | PPH00241B |
G07 | Hs.279594 | NM_001065 | TNFRSF1A | Tumor necrosis factor receptor superfamily, member 1A | CD120a/FPF | PPH00346B |
G08 | Hs.462529 | NM_003790 | TNFRSF25 | Tumor necrosis factor receptor superfamily, member 25 | APO-3/DDR3 | PPH00349A |
G09 | Hs.654481 | NM_000546 | TP53 | Tumor protein p53 | LFS1/TRP53 | PPH00213E |
G10 | Hs.66744 | NM_000474 | TWIST1 | Twist homolog 1 (Drosophila) | ACS3/BPES2 | PPH02132A |
G11 | Hs.563491 | NM_017549 | EPDR1 | Ependymin related protein 1 (zebrafish) | EPDR/MERP-1 | PPH09305E |
G12 | Hs.73793 | NM_003376 | VEGFA | Vascular endothelial growth factor A | MVCD1/VEGF | PPH00251B |
H01 | Hs.534255 | NM_004048 | B2M | Beta-2-microglobulin | B2M | PPH01094E |
H02 | Hs.412707 | NM_000194 | HPRT1 | Hypoxanthine phosphoribosyltransferase 1 | HGPRT/HPRT | PPH01018B |
H03 | Hs.523185 | NM_012423 | RPL13A | Ribosomal protein L13a | RPL13A | PPH01020B |
H04 | Hs.592355 | NM_002046 | GAPDH | Glyceraldehyde-3-phosphate dehydrogenase | G3PD/GAPD | PPH00150E |
H05 | Hs.520640 | NM_001101 | ACTB | Actin, beta | PS1TP5BP1 | PPH00073E |
H06 | N/A | SA_00105 | HGDC | Human Genomic DNA Contamination | HIGX1A | |
H07 | N/A | SA_00104 | RTC | Reverse Transcription Control | RTC | |
H08 | N/A | SA_00104 | RTC | Reverse Transcription Control | RTC | |
H09 | N/A | SA_00104 | RTC | Reverse Transcription Control | RTC | |
H10 | N/A | SA_00103 | PPC | Positive PCR Control | PPC | |
H11 | N/A | SA_00103 | PPC | Positive PCR Control | PPC | |
H12 | N/A | SA_00103 | PPC | Positive PCR Control | PPC |
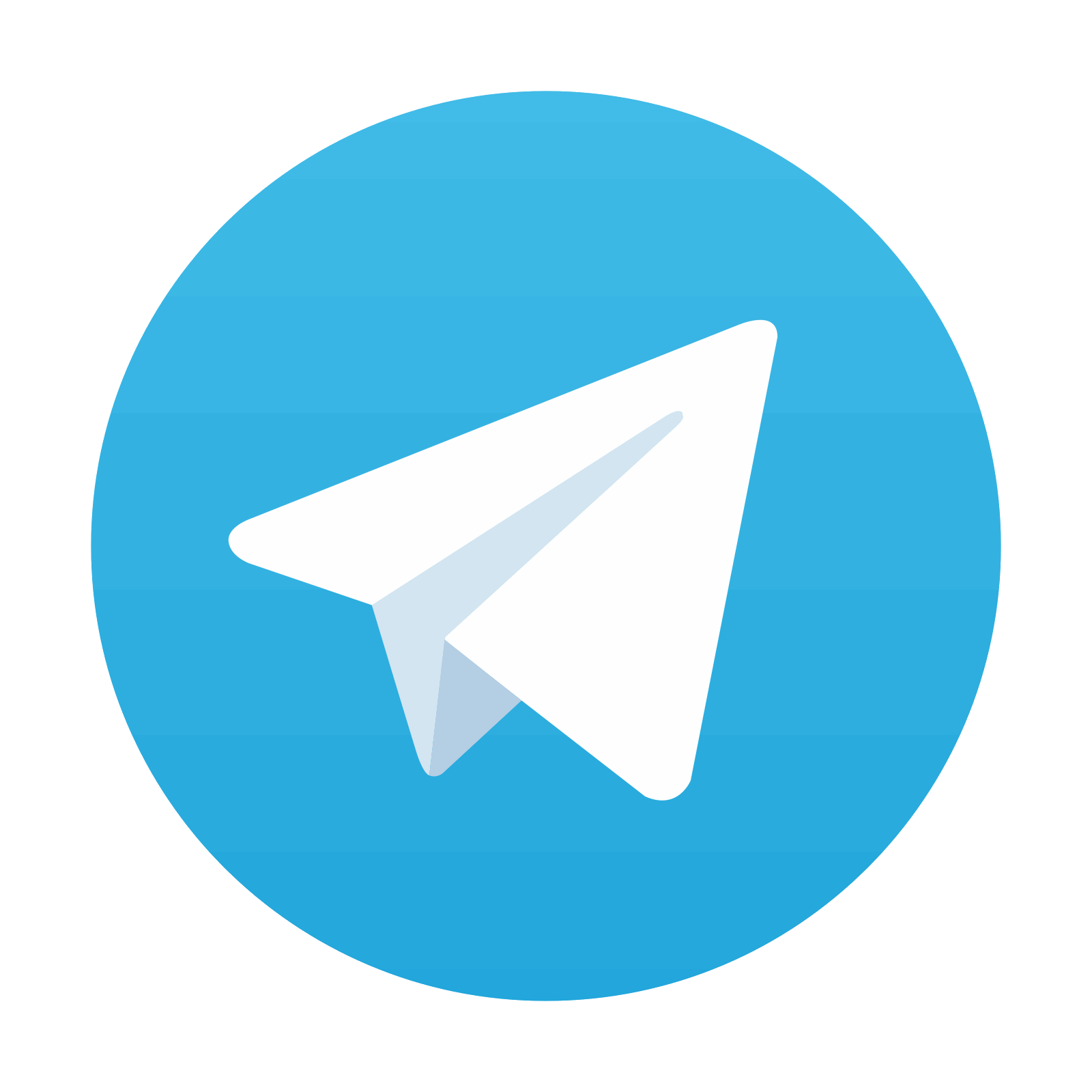
Stay updated, free articles. Join our Telegram channel

Full access? Get Clinical Tree
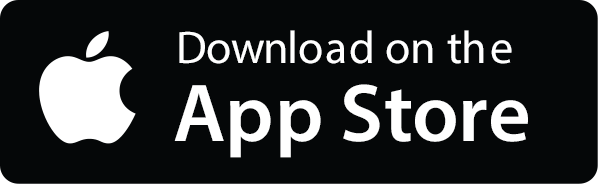
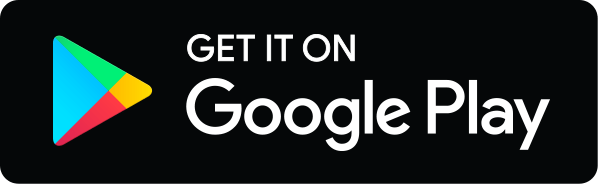