After studying this chapter you should be able to:
Knowledge criteria
- •
Describe normal placental development
- •
Describe the structure of the umbilical cord and uteroplacental blood flow
- •
List the placental transfer mechanisms and functions
- •
Describe the sequence of normal fetal development
- •
Understand the formation and clinical significance of amniotic fluid
Early placental development
After fertilization and egg cleavage, the morula is transformed into a blastocyst by the formation of a fluid-filled cavity within the ball of cells.
The outer layer of the blastocyst consists of primitive cytotrophoblast and, by day 7, the blastocyst penetrates the endometrium as a result of trophoblastic invasion ( Fig. 4.1 ). The outer layer of trophoblast becomes a syncytium. In response to contact with the syncytiotrophoblast, the endometrial stromal cells become large and pale, a process known as the decidual reaction . Some endometrial cells are phagocytosed by the trophoblastic cells.

The nature and function of the decidual reaction remain uncertain, but it seems likely that the decidual cells both limit the invasion of trophoblastic cells and serve an initial nutritional function for the developing placenta.
During development of the placenta, cords of cytotrophoblast or Langhans cells grow down to the basal layers of decidua and penetrate some of the endometrial venules and capillaries. The formation of lacunae filled with maternal blood presages the development of the intervillous space.
The invading cords of trophoblast form the primary villi, which later branch to form secondary villi and, subsequently, free-floating tertiary villi.
The central core of these villi is penetrated by a column of mesoblastic cells that become the capillary network of the villi. The body stalk attaching the developing fetus to the placenta forms the umbilical vessels, which advance into the villi to join the villous capillaries and establish the placental circulation.
Although trophoblastic cells surround the original blastocyst, the area that develops into the placenta becomes thickened and extensively branched and is known as the chorion frondosum . However, in the area that subsequently expands to form the outer layer of the fetal membranes or chorion laeve , the villi become atrophic and the surface becomes smooth ( Fig. 4.2 ). The decidua underlying the placenta is known as the decidua basalis and the decidua between the membranes and the myometrium as the decidua capsularis .

Further placental development
By 6 weeks after ovulation, the trophoblast has invaded some 40–60 spiral arterioles. Blood from the maternal vasculature pushes the free-floating secondary and tertiary capillaries into a tent-shaped maternal cotyledon . The tents are held down to the basal plate of the decidua by anchoring villi, and the blood from arterioles spurts towards the chorionic plate and then returns to drain through maternal veins in the basal plate. There are eventually about 12 large maternal cotyledons and 40–50 smaller ones ( Fig. 4.3 ).

The villus
Despite the arrangement of villi into maternal cotyledons, the functional unit of the placenta remains the stem villus or fetal cotyledon . The end unit of the stem villus, sometimes known as the terminal or chorionic villus is shown in Figure 4.4 . There are initially about 200 stem villi arising from the chorion frondosum. About 150 of these structures are compressed at the periphery of the maternal cotyledons and become relatively functionless, leaving a dozen or so large cotyledons and 40–50 smaller ones as the active units of placental function.

The estimated total surface area of the chorionic villi in the mature placenta is approximately 11 m 2 . The surface area of the fetal side of the placenta and of the villi is enlarged by the presence of numerous microvilli. The core of the villus consists of a stroma of closely packed spindle-shaped fibroblasts and branching capillaries. The stroma also contains phagocytic cells known as Hofbauer cells . In early pregnancy, the villi are covered by an outer layer of syncytiotrophoblast and an inner layer of cytotrophoblast. As pregnancy advances, the cytotrophoblast disappears until only a thin layer of syncytiotrophoblast remains. The formation of clusters of syncytial cells, known as syncytial knots , and the reappearance of cytotrophoblast in late pregnancy are probably the result of hypoxia. There is evidence that the rate of apoptosis of syncytial cells accelerates towards term and is particularly increased where there is evidence of fetal growth impairment.
Structure of the umbilical cord
The umbilical cord contains two arteries and one vein ( Fig. 4.5 ). The two arteries carry deoxygenated blood from the fetus to the placenta and the oxygenated blood returns to the fetus via the umbilical vein. Absence of one artery occurs in about 1 in 200 deliveries and is associated with a 10–15% incidence of cardiovascular anomalies. The vessels are surrounded by a hydrophilic mucopolysaccharide known as Wharton’s jelly and the outer layer covering the cord consists of amniotic epithelium. The cord length varies between 30 and 90 cm.

The vessels grow in a helical shape. This configuration has the functional advantage of protecting the patency of the vessels by absorbing torsion without the risk of kinking or snarling of the vessels.
The few measurements that have been made in situ of blood pressures in the cord vessels indicate that the arterial pressure in late pregnancy is around 70 mmHg systolic and 60 mmHg diastolic, with a relatively low pulse pressure and a venous pressure that is exceptionally high, at approximately 25 mmHg. This high venous pressure tends to preserve the integrity of the venous flow and indicates that the pressure within the villus capillaries must be in excess of the cord venous pressures.
The high capillary pressures imply that, at the point of proximity, the fetal pressures exceed the pressures in the choriodecidual space, so that any disruption of the villus surface means that fetal blood cells enter the maternal circulation and only rarely do maternal cells enter the fetal vascular space.
The cord vessels often contain a false knot consisting of a refolding of the arteries; occasionally, blood flow is threatened by a true knot, although such formations are often seen without any apparent detrimental effects on the fetus.
In the full-term fetus, the blood flow in the cord is approximately 350 mL/min.
Uteroplacental blood flow
Trophoblastic cells invade the spiral arterioles within the first 10 weeks of pregnancy and destroy some of the smooth muscle in the wall of the vessels which then become flaccid dilated vessels. Maternal blood enters the intervillous space and, during maternal systole, blood spurts from the arteries towards the chorionic plate of the placenta and returns to the venous openings in the placental bed. The intervillous space is characterized by low pressures, with a mean pressure estimated at 10 mmHg and high flow. Assessments of uterine blood flow at term indicate values of 500–750 mL/min ( Fig. 4.6 ).

Factors that regulate fetoplacental and uterine blood flow
The fetoplacental circulation is effected by the fetal heart and aorta, the umbilical vessels and the vessels of the chorionic villi, so factors that affect these structures may affect the fetal circulation. Such factors as oedema of the cord, intramural thrombosis and calcification within the large fetal vessels or acute events such as acute cord compression or obstruction of the umbilical cord may have immediate and lethal consequences for the fetus. However, the more common factors that influence the welfare of the fetus arise in the uteroplacental circulation. Access to these factors by the use of Doppler ultrasound has greatly improved our understanding of the control mechanisms of uterine blood flow.
The regulation of uterine blood flow is of critical importance to the welfare of the fetus. The uteroplacental blood flow includes the uterine arteries and their branches down to the spiral arterioles, the intervillous blood flow and the related venous return.
Impairment of uterine blood flow leads to fetal growth impairment and, under severe circumstances, to fetal death. Factors that influence uteroplacental blood flow acutely include maternal haemorrhage, tonic or abnormally powerful and prolonged uterine contractions and substances such as noradrenaline (norepinephrine) and adrenaline (epinephrine). Angiotensin II increases uterine blood flow at physiological levels, as it has a direct effect on the placental release of vasodilator prostaglandins, but in high concentrations it produces vasoconstriction.
At the simplest level, acute fetal asphyxia can be produced by the effect of the mother lying in the supine position in late pregnancy, causing compression of the maternal inferior vena cava and hence a sudden reduction in blood flow through the uteroplacental bed.
In terms of chronic pathology, the main causes of impaired uteroplacental circulation are inadequate trophoblast invasion and acute atherosis affecting the spiral arterioles; resulting in placental ischaemia, advanced maturation and placental infarction.
Placental transfer
The placenta plays an essential role in growth and development of the fetus and in regulating maternal adaptation to pregnancy. The placenta is an organ of fetal nutrition, excretion, respiration, and of hormone synthesis.
Transfer of materials across the placental membrane is governed by molecular mass, solubility and the ionic charge of the substrate involved. Actual transfer is achieved by simple diffusion, facilitated diffusion, active transport and pinocytosis ( Fig. 4.7 ).

Simple diffusion
Transfer between maternal and fetal blood is regulated by the trophoblast, and it must be remembered that the layer separating fetal from maternal blood in the chorionic villus is not a simple semipermeable membrane but a metabolically active cellular layer. However, with regard to some substances, it does behave like a semipermeable membrane and substances pass by simple diffusion.
Although there are some exceptions, small molecules generally cross the placenta in this way and movement is determined by chemical or electrochemical gradients. The quantity of solute transferred is described by the Fick diffusion equation:
Q t = K A ( C 1 − C 2 ) L
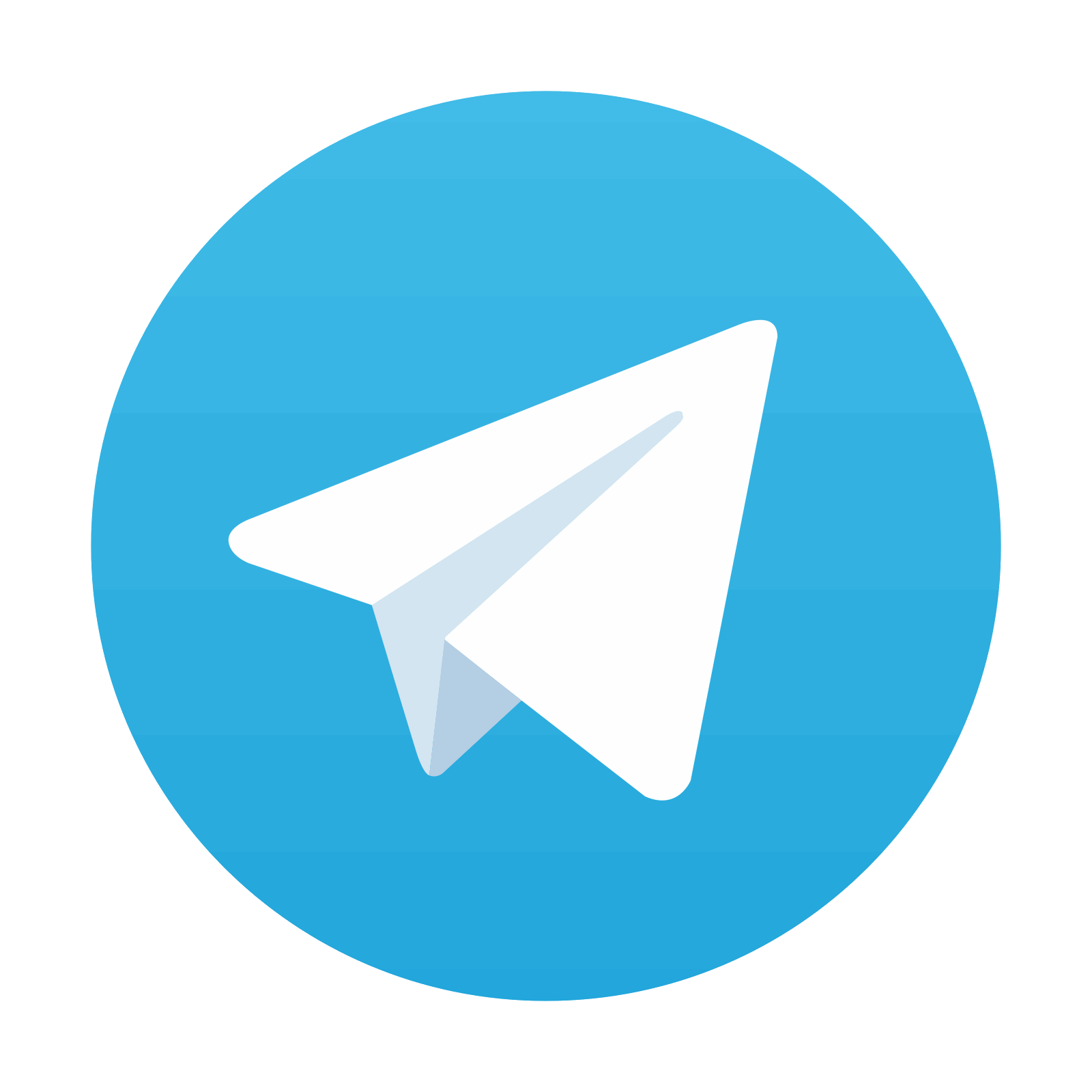
Stay updated, free articles. Join our Telegram channel

Full access? Get Clinical Tree
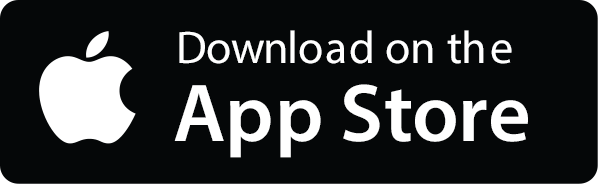
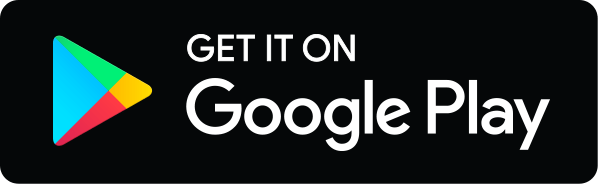