Bilirubin is formed in the organism by oxidation–reduction reactions. It is the end product of heme catabolism (Figure 5-1). The heme comes mainly from aging red blood cells (RBCs), but muscle myoglobin and some liver enzymes such as cytochromes and catalases are a partial source.1 Production of erythrocytes is first observed in the fetal yolk sac at 2–3 weeks of gestation. RBC production in the liver is seen at about 6 weeks of gestation and in the marrow at 20 weeks.2 The mean life span of RBCs in the term newborn infant is around 45–90 days, and about 35–50 days in premature infants.3 Fetal and adult hemoglobin are structurally different. Together with a higher hemoglobin concentration, this explains the increased oxygen-carrying capacity in the fetus. Postnatally, these mechanisms are no longer needed. Production of adult hemoglobin starts in the last trimester of pregnancy, and in the absence of certain hemoglobinopathies the production of fetal hemoglobin is turned off at birth.3
Bilirubin-IXβ appears in the human fetus at 14 weeks of gestation.4 Two weeks later unconjugated bilirubin-IXα appears in bile. By 20 weeks of gestation IXα constitutes 6% of bilirubin in bile, IXβ 87%, IXγ 0.5%, and IXδ 6%.5 By 38 weeks gestation, bilirubin-IXα has replaced IXβ as the main isomer.4
In fetal hemolytic disease, such as Rhesus immunization, the concentration of total serum bilirubin (TSB) can increase from 1.5 mg/dL at 20 weeks of gestation to 4.1 mg/dL at 32 weeks. Weiner described an inverse relationship between TSB and hemoglobin concentrations.6 Thus, when fetuses became severely anemic (hematocrit <30), 82% had TSB concentrations exceeding the 97.5th percentile on the Bhutani nomogram.7 Increased TSB could be detected weeks before the development of anemia. A TSB >3 mg/dL was associated with a high likelihood of severe fetal anemia. Goodrum et al. studied the effect of repeated intrauterine transfusions and found a mean TSB value of 5.6 mg/dL (1.3–10.8 mg/dL) at the time of the third transfusion, almost all unconjugated.8 Thus, although bilirubin can pass the placenta (see below), fetal hemolytic disease is accompanied by fetal hyperbilirubinemia. It therefore appears that the placenta has a limited capacity for transfer of bilirubin, which is exceeded in fetal hemolytic disease.
The first step in the breakdown of heme is catalyzed by heme oxygenase (HO), and is the rate-limiting step in heme degradation (Figure 5-1).9,10 This enzyme is found in the reticuloendothelial system, and also in tissue macrophages and in gut mucosa.11 Formation of biliverdin is an intermediate step in bilirubin production. Because carbon monoxide (CO) is a by-product of this process, measurement of CO in exhaled breath, corrected for ambient CO (ETCOc), can be used to estimate bilirubin production (see Chapter 2). The production rate in the neonate was estimated to be about 8.5 mg/kg per day, about double the rate of 4 mg/kg per day in adults.12
Reduction of biliverdin-IXα to bilirubin-IXα takes place in the cytosol and is catalyzed by biliverdin reductase.13 Biliverdin has a blue-green color and is water soluble, while bilirubin has a strong yellow-to-orange color. Bilirubin-IXα occurs in several isomeric forms, but in the absence of skin exposure to strong light is found mostly as the (4Z,15Z) isomer in the serum of humans. This bilirubin isomer appears to be lipophilic, which may be explained by intramolecular hydrogen bonds between the side groups (Z, zusammen—German for “together”).13,14 It is this solubility characteristic that allows bilirubin to cross tissue membranes and explains its ability to enter the brain. A sketch of bilirubin metabolism is shown in Figure 5-1.
Heme catabolism releases the iron bound to heme for renewed use by the body, and CO for release through the respiration. HO is needed to convert heme to biliverdin. Therefore, it must be active when bilirubin first appears in the fetus at 14 weeks gestation.4 HO has a broad range of substrate affinities, but the oxidative degradation of heme is alpha-specific.
Three functional isoforms of HO (HO-1, HO-2, and HO-3) have been described in humans. Of these, HO-2 and HO-3 are constitutive and HO-1 is inducible. HO-1 and HO-2 have roles in heme catabolism, but the role of HO-3 has not been well elucidated.15,16 HO may be inhibited by a number of metalloporphyrins, a phenomenon which has a therapeutic potential.17
In the fetus, microsomal HO activity in the liver may be as much as eight times higher than in the adult.18 This suggests that the increased catabolism of heme in neonates may be associated with an increased transcription of the inducible HO isoform, HO-1. However, in a study of healthy mature and premature infants HO-1 was induced until day 2 or 3 after birth, possibly suggesting oxidative stress, but by the end of the first week the HO-1 mRNA level had dropped below the level at birth.19 HO-1 levels and inducibility were not dependent on gestational age, at least down to the 26 weeks gestation which was investigated. Deficiency of HO-1 has been described in one single patient who suffered from growth failure, anemia, tissue iron deposition, lymphadenopathy, leukocytosis, and increased sensitivity to oxidant injury.20
HO is expressed in human placenta, where it contributes to normal placental function. The amounts of mRNA in placenta that encode both the HO-1 and HO-2 isoforms have been measured, showing an elevated expression of HO-2 relative to HO-1.21 Furthermore, the expression of both HO-1 and HO-2 in the placenta increased with gestational age.21
Unconjugated bilirubin (UCB) is transported in plasma bound to albumin with a binding affinity of 107–108/M at the primary binding site.22 There is also a secondary binding site with lower affinity.22,23 Because of the high affinity of albumin for bilirubin, equilibrium concentrations of free (unbound) bilirubin in plasma are only in the low nanomolar range, even in significant hyperbilirubinemia.24,25 However, when the molar concentration of bilirubin exceeds that of albumin, free bilirubin concentrations may increase significantly.22,24,26 Binding of bilirubin to albumin increases with postnatal age but is reduced in sick infants and in the presence of exogenous or endogenous binding competitors.27–30 In addition to albumin, bilirubin can also bind to other proteins (e.g., α-fetoprotein and ligandin), as well as to lipoproteins and to erythrocytes.31–34
When the bilirubin–albumin complex comes in contact with hepatocytes, bilirubin is transported into the cell. Three different transport proteins may be involved.35,36 These appear to be dominant at different substrate concentrations, as well as having different driving forces. It has been suggested that the apparent receptor effect may be due to clusters of membrane proteins with high affinities for specific classes of ligands.35 These protein clusters may be located in the sinusoidal liver cell plasma membrane.
In liver cells about 60% of bilirubin is found in the cytosol and about 25% in microsomes.37 Ligandin, a glutathione S-transferase (GST), binds bilirubin in the hepatocytes. In fetal rat liver, the activity of GST is <5% of adult values on day 16 of pregnancy and ∼10% on day 20.38 Basic monomers of GST are present in human fetal liver cytosol at 21 weeks gestation, while near-neutral isoenzymes do not appear until 30 weeks.39
Bilirubin metabolism is different in the fetus compared with the neonate. UCB in a mammalian fetus can be disposed of either by crossing the placenta into the maternal circulation or by excretion into fetal bile. Excretion into bile causes bilirubin to accumulate in meconium, but only in an amount that corresponds to 5–10 times daily production.43 Therefore, elimination across the placenta is the major route for excretion of fetal UCB.44 Placental membranes are more or less impermeable to such polar compounds as biliverdin and bilirubin conjugates,44 but nonpolar compounds such as UCB can diffuse across.44,45 Evidence obtained using plasma membrane vesicles from human placental trophoblast46 and an in vivo experimental model of in situ perfused rat placenta at term47 point to an important role for carrier-mediated transport of UCB across the placenta. OATP8 may play an important role in this context.48
Inside trophoblast cells, bilirubin may be bound in part to lipids and proteins (e.g., GST).49 Bilirubin export across the apical pole of the trophoblast may occur via an ATP-dependent mechanism.46 Several isoforms of multidrug resistance proteins (MRPs) are expressed in human50 and rat51,52 placentas. Whether these are involved in bilirubin export is as yet not known. MRP2, possibly also MRP1, is able to transport bilirubin glucuronides.53 However, UDP-glucuronosyl transferase (UGT) activity in fetal liver is low, and biotransformation of UCB appears not to occur during transplacental transfer.54 Thus, MRP2 and MRP1 may not play an important role in bilirubin transfer across the placenta.
Comparing TSB levels in samples from umbilical artery and vein as well as from maternal serum, Rosenthal found that the bilirubin concentration in the artery was nearly double that of the vein.55 Thus, bilirubin appears to be cleared quite efficiently from fetal blood during passage through the placenta. In Rosenthal’s study the transplacental gradient of bilirubin from fetus to mother was ∼10:1, while Monte et al. found a gradient of ∼5:1.56
Bilirubin has been found in amniotic fluid from the 12th week of gestation. However, it gradually disappears as the volume of amniotic fluid increases, and normally near-term amniotic fluid does not contain measurable bilirubin.57 Bilirubin was first found in amniotic fluid from rhesus immunized women.58 The levels of amniotic fluid bilirubin correlated with the degree of fetal affection.59 Amniotic fluid is primarily a fetal product; thus, bilirubin in this fluid most likely comes from the fetus itself. Although several modes of transfer are possible, recent data support the intramembranous pathway as the only one that is compatible with the observed amniotic fluid/fetal blood ratios, and it is therefore likely to be the most important.60
Medical literature contains a few reports of infants who have been exposed to significant maternal hyperbilirubinemia in utero. An infant born to a mother with the Crigler–Najjar syndrome had a cord TSB of 24 mg/dL of which 12.7 mg/dL was unconjugated. Although neurological symptoms were absent in the newborn period, follow-up at 18 months revealed quadriplegia.61
Two infants born to women with the Crigler–Najjar syndrome received exchange transfusions soon after birth and had normal neurodevelopmental outcomes at 18 months and 4 years of age, respectively.62 Of note, a brain magnetic resonance imaging (MRI) of one of these infants performed on the fifth day of life showed a high T1 signal in the globus pallidus bilaterally, consistent with acute bilirubin encephalopathy (ABE), apparently without clinical neurological symptoms. His TSB at birth was 18.9 mg/dL. Brain MRI of the other infant was normal, although a cord TSB at birth had been 24.6 mg/dL. Evidence for reversibility of ABE has recently been published,63 and the above infant with MRI changes, but normal outcome, appears to add to such cases.
There appears to be marked interspecies differences in fetal handling of bilirubin. Therefore, extrapolating from animal data to humans requires caution.64 For example, fetal dog and sheep livers excrete about 50% of injected radiolabeled bilirubin as conjugates, while the fetal monkey liver excretes only about 5% of the dose, and very little as conjugates.65,66 In rats monoconjugates appear in the intestines on fetal day 18, while diconjugates show up 2 days later.67 On day 18, 80% of intestinal bilirubin is unconjugated, while from day 20, the conjugated pigment predominates. Thus, in this species there appears to be a rapid development of the conjugation mechanisms in the last few days of fetal life.
An organism can excrete heme directly in bile,68 a phenomenon which is currently being exploited in the prevention and treatment of neonatal jaundice through the use of metalloporphyrins, inhibitors of HO17 (see Chapter 2). However, direct excretion of heme in the bile would result in the loss of iron, whereas conversion of heme to biliverdin conserves this valuable resource for the body. Biliverdin can also be excreted in bile,69 and although it makes sense for the fetus to reduce biliverdin to bilirubin that can cross the placenta, it is something of a biological puzzle why we continue after birth to produce and excrete potentially toxic bilirubin rather than stop the process at nontoxic biliverdin. Birds appear to lack biliverdin reductase in their reticuloendothelial system and thus excrete biliverdin rather than bilirubin.13 The discovery that bilirubin is a free radical quencher could possibly be a piece in this puzzle.70,71
The last step of the bilirubin conjugation process takes place at the cell membrane.72 Excretion of conjugated bilirubin into bile occurs against a concentration gradient, thus requiring energy. In rabbits excretion of conjugated bilirubin into bile is enhanced by the intravenous (IV) infusion of glucose.73 The Dubin–Johnson syndrome is caused by a defect in the gene encoding the hepatocyte bilirubin conjugate export pump (MRP2).74,75 Clearance of bilirubin from the hepatocyte is a saturable process, and after the first week of life appears to be the rate-limiting step in the excretion of bilirubin.76,77 The transport maximum can be increased by drugs that stimulate bile flow (e.g., barbiturates).78
When bilirubin is conjugated with glucuronic acid, the reaction is catalyzed by uridine diphosphoglucuronosyl transferase (UDPGT or UGT, EC 2.4.1.17). Conjugation makes bilirubin water soluble and permits transfer of bilirubin into bile. UGT activity is low at birth but increases to adult values by 1–2 months. As for ligandin, certain drugs (phenobarbital, dexamethasone, clofibrate) increase UGT activity (see below).
The activity of UGT in fetuses has been measured to be 0.1% at 16–32 weeks gestation, increasing to ∼1% of adult values at term.79 Conjugated bile pigments were found in the liver of fetuses with Rhesus incompatibility.80 Bilirubin-IXα glycosyl conjugates appear in human fetal bile at 20 weeks gestation, while monoglucuronide is first seen 2–3 weeks later.81 Monoconjugates of bilirubin-IXα are the predominant bilirubins in human fetal bile from around 30 weeks gestation, but only at term do bilirubin-IXα monoconjugates with glucuronic acid, that is, monoglucuronides, constitute the major pigment.81 In comparison, adult bile contains about 80% bilirubin-IXα diglucuronide and 18% monoglucuronide.82 Premature birth accelerates the development of UGT activity in human liver.79 In the first days of life, conjugated bilirubin constitutes <2% of total bile pigment in serum.83 Diconjugates make up about 20% of the total conjugated fraction in babies, less than half of the estimated >50% in adults.83
In the endoplasmic reticulum of the microsomes, UGT catalyzes the binding first of one molecule of glucuronic acid to bilirubin, forming bilirubin monoglucuronide.78 Human bilirubin UDP-glucuronosyl transferase is a tetramer with molecular weight 209 kD.84 The monomer can convert UCB to the monoglucuronide, but only the tetramer can convert the monoglucuronide to the diglucuronide.84 The importance of this process is that the essentially water-insoluble UCB is converted to a water-soluble form, which can be excreted in the bile. Conjugation of bilirubin in the liver is controlled by a gene complex on chromosome 2, at 2q37.85 Mutations and amino acid substitutions are responsible for genetic errors in bilirubin conjugation such as the Crigler–Najjar syndrome types I and II, and Gilbert syndrome (see elsewhere in this chapter as well as Chapter 1).
Giving phenobarbital to pregnant women increases conjugation in the neonate.86 Therefore, phenobarbital has been used both before and after birth to prevent and/or treat neonatal jaundice.87 UGT activity is also increased by administration of a thyroid analog,88 dexamethasone,89 and clofibrate.90
The principal intracellular bilirubin binding protein in the liver is ligandin, a GST.91 Uptake of bilirubin into hepatocytes seems to increase with increasing concentrations of ligandin.37 Ligandin has two binding sites for bilirubin, one with high (Ka = 5 × 107/M) and one with lower (Ka = 3 × 105/M) affinity.97 The high-affinity site is not the same as the catalytic site, but binding to the low-affinity site is associated with competitive inhibition of the enzymatic activity of ligandin.92
The neonate is relatively deficient in ligandin, thus decreasing the ability to retain bilirubin inside the hepatocyte. This may cause bilirubin to reflux into the circulation. The concentration of ligandin in hepatocytes can be increased by pharmacological agents such as phenobarbital.37 Recent evidence points to the possibility that polymorphisms of the GST gene GSTM1 may affect ligandin functions in hepatocytes, and individuals with the GSTM1-null genotype may have higher TSB levels in the neonatal period.93
In the intestines bilirubin is reduced to colorless tetrapyrroles by microbes in the colon. The infant is born with a sterile gut, and the intestinal microbial flora normally contributes to further breakdown of bilirubin, which is established during the first days and weeks of life. Some deconjugation may occur in the proximal small intestine through β-glucuronidases located in the brush border.93 UCB can be reabsorbed into the circulation, increasing the total plasma bilirubin pool. This cycle of uptake, conjugation, excretion, deconjugation, and reabsorption is called “enterohepatic circulation” (Figure 5-1). Enterohepatic circulation may be significant in the newborn infant, in part due to limited nutrient intake in the first days of life, which delays intestinal transit. There is also a lack of a binding vehicle in the intestinal lumen to transport bilirubin conjugates through the gut.
In addition, it is thought that in some mother/infant dyads factors in breast milk, which have not been unequivocally identified, may contribute to increased enterohepatic circulation in so-called breast milk–associated jaundice. The risk of breast milk jaundice appears to be increased in infants who have genetic polymorphisms in the coding sequences of the UGT1A1 or OATP2 genes.94 The mechanisms that cause this phenomenon are still uncertain. However, it appears that supplementation with breast milk substitutes may reduce the degree of breast milk jaundice.95
Meconium contains a significant amount of bilirubin, estimated to be about 5–10 times the daily production.78 Half of this is UCB and thus capable of being reabsorbed. Bilirubin-IXβ is the predominant bile pigment in the first excreted batch of meconium. It decreases subsequently, and is a biochemical marker of meconium, as is zinc coproporphyrin.96
Enterohepatic circulation is increased by any condition that causes delayed or interrupted passage of intestinal contents. This is evident in intestinal atresias,97 in infants kept off oral feeds for reasons of severe illness, and in infants receiving inadequate nutrition due to difficulties in establishing lactation (“lack-of-breast milk jaundice”). Conversely, enterohepatic circulation is reduced by establishment of enteral nutrition, by increasing the frequency of feeding,98 by oral feeding of agar, which binds bilirubin in the gut,99 and by oral administration of bilirubin oxidase.100 It should be noted that the latter two treatments must be considered “off-label” and have not been subjected to any approval process of which we are aware.
Delayed passage of meconium has been believed to be associated with hyperbilirubinemia. In a study, 84 healthy term neonates were randomly assigned to a suppository group and a nonsuppository group.101 The suppositories resulted in earlier evacuation of meconium. However, no significant effect was noted on TSB levels in the first 3 days of life. In another study, early evacuation of meconium through glycerin enemas given within 30 minutes after birth, and 12 hours after birth, had no effect on peak TSB levels or on TSB concentrations in the first 7 days of life.102 Thus, routine use of suppositories or enemas to decrease TSB levels is unlikely to be effective and cannot be recommended for term healthy neonates.
Neonatal hyperbilirubinemia affects nearly all newborns, in the sense that TSB levels above the range considered as normal during the remainder of an individual’s life cycle are typically present in neonates. Thus, almost all newborn infants will develop a TSB >1.8 mg/dL during the first week of life.103 Neonatal jaundice occurs when TSB increases to a level where the accumulation of bilirubin in skin becomes visible to the unaided eye in daylight conditions (or similar quality artificial light). Such visual detection is possible when TSB exceeds 5–6 mg/dL, but actual detection will vary between observers and depend on lighting conditions. The type of feeding also influences the expected course of jaundice in the neonate. Some infants affected by breast milk jaundice may remain visibly jaundiced for weeks after birth (“breast milk jaundice”).
Jaundice typically first becomes visible on the forehead (Figure 5-2A). With time, jaundice moves further down the body.104 This may be clinically useful because visible jaundice in the hands or feet may suggest a significantly elevated TSB level that one should consider checking by appropriate tests (Figure 5-2B). In most infants, yellow color is the only finding on physical examination, although infants who have pronounced jaundice may be drowsy.
Figure 5-2.


Zones of dermal jaundice. A. Location of dermal jaundice zones 1–5. B. Box and whisker plots of relation between TSB values and dermal jaundice zones 1–5. (Based on recalculated data abstracted from Kramer.104)
Textbooks may refer to neonatal jaundice as either physiological or nonphysiological (or pathologic). Physiological jaundice is due to the normal occurrences of increased breakdown of RBCs in the presence of a low capacity for uptake, conjugation, and excretion of bilirubin in the liver. Reabsorption of bilirubin from the bowel because of relatively low nutrient intake and reduced intestinal transit in the first days of life must be said to be part of this normal phenomenon. Nonphysiological or pathologic jaundice refers to situations where hyperbilirubinemia is accentuated by diseases or conditions such as genetic variations, leading, for example, to increased hemolysis or reduced excretion of bilirubin (or both combined). It is important to note that there is often not a clear-cut distinction between physiological and pathologic jaundice in newborns. Each of the normal mechanisms that produce hyperbilirubinemia may be affected. A priori an infant with a very high serum bilirubin level is more likely to have a pathologic cause contributing to his or her jaundice than an infant with a low serum bilirubin level, but a simple measurement of serum bilirubin is not enough to ascertain this. From a didactic perspective we have found it helpful to compare this situation with measuring the height of a mountain that is topped by an ice cap. The measurement obtained at the summit will not tell you how many meters (or feet) is contributed by the rock (i.e., “physiology”) and how many by ice (i.e., “pathology”).
As mentioned, jaundice is a very common phenomenon in neonates, and a frequent reason for clinical concern and investigation. In a study of 490 term and near-term infants from a racially diverse background, 80% of infants were found to have TSB concentrations greater than 5 mg/dL.105 This is approximately the value at which the human eye can detect jaundice due to unconjugated hyperbilirubinemia by looking at the skin. In contrast, as shown in Figure 5-3, conjugated hyperbilirubinemia, such as in cholestatic jaundice in the adult, is readily detected at levels as low as 1.8 mg/dL.106
Figure 5-3.

Visual identification of jaundice. Box and whisker plots of relationship between visible (V), uncertain (U), and not visible (N-V) dermal jaundice and TSB values in adults and infants. (Recalculated from data from With.106)
Most surveys of the range of normal TSB levels in North American newborns identify peak TSB in normal newborns to be 14–15 mg/dL at the third to fifth days of life. It has been estimated that bilirubin production in the neonate is about 8.5 mg/kg per day,12 equaling 30 mg per day (50 μmol per day) in a 3.5-kg term infant. Thus, if no bilirubin conjugation and excretion took place, 90 mg (150 μmol) of bilirubin would have accumulated in the body at day 3 of life. If we assume that albumin-bound UCB distributes only in the plasma volume, peak TSB levels in these infants would readily reach 64 mg/dL on average instead of the usual normal peak value of 15 mg/dL. This suggests that the liver of the newborn infant, in spite of decreased ability to take up, conjugate, and excrete bilirubin, is still able to dispose most of the bilirubin produced in the early neonatal period.
When presentation of jaundice occurs during the first 24 hours of life or later than days 3–4 of life, it is likely that factors in addition to those involved in the normal physiological adaptation of the newborn may contribute. This is discussed further in section “Jaundice as a Sign of Disease.”
The diagnosis of jaundice in a newborn typically begins with the mother or caregivers recognizing the yellow color of the infant’s skin. Targeted screening has, however, become more common, and has been recommended in guidelines.107–109 Jaundice in the neonate is first visible in the face and forehead, but can be difficult to assess in polycythemic infants or in infants with a reddish or dark hue. Pressure on the skin helps to reveal the underlying color. However, visual inspection might not be reliable for assessing the degree of jaundice as pointed out in several studies.110–112
In most infants, yellow color is the only finding on physical examination, although infants who have pronounced jaundice may also appear drowsy and feed poorly. When called upon to assess a newborn infant for jaundice, the physician should consider whether: (i) the infant looks generally healthy; (ii) it is necessary to measure a TSB level; (iii) treatment is indicated, and (iv) elements in the history or physical examination suggest that nonphysiological factors may contribute to jaundice (see section “Jaundice as a Sign of Disease”).
If an infant appears more than mildly jaundiced by visual inspection, an objective measurement of a TSB level should be measured. In most infants who develop a moderate jaundice on second or third day of life, and without any history and/or physical findings suggestive of a pathologic process, a TSB measurement is the only necessary test. Bilirubin can be measured noninvasively in the skin by transcutaneous bilirubinometry (TcB). This technique is commonly used as a screening instrument to decide whether there is a need to measure TSB.113
Measurement of TSB is necessary for determining whether therapy should be instituted since therapeutic guidelines mostly refer to TSB values. Measurement of TcB using handheld devices performs better than visual assessment. In the presence of apparently mild jaundice, this may be sufficient to assure that TSB levels are safely below intervention levels. In a recent study of a 1-year cohort of infants in a normal newborn nursery, blood tests for TSB determinations were performed in 598 (16.4%) of 3648 infants,114 and phototherapy was instituted in 45% of infants tested.
It is well known that measurement of TSB in a routine clinical setting represents a challenge. The test results can be significantly affected by the technique for blood sampling, transport time, and conditions, as well as the analytical method.115 Recent studies indicate, however, that variation in analytical quality of TSB measurements within and between hospitals is acceptable, and does not represent a critical factor in the diagnosis and treatment of jaundiced newborn infants.116
Although it has been shown that TcB correlates relatively well with TSB, the difference between these two measurements can be significant in individual infants109 (Figure 5-4). It has therefore been recommended that infants with a TcB within 2.9 mg/dL of treatment indication or with a TcB >14.6 mg/dL should have TSB measured before treatment is evaluated.117 Since it has been shown that TcB measurements can be inaccurate in infants with darkly pigmented skin, it is also recommended that TSB should be measured routinely in such infants around 24 hours of age. It should also be emphasized that TcB is not useful during and in the first hours after phototherapy has been discontinued due to fading of the skin.
Measurement of conjugated bilirubin in serum is not necessary in most infants. However, in the presence of an enlarged liver or spleen, petechiae, thrombocytopenia, or other findings suggesting liver or biliary disease, metabolic disease, or congenital infection, bilirubin fractions should be measured. Measurement of the conjugated bilirubin fraction is also indicated in infants who remain jaundiced beyond the first 2 weeks of life and infants whose TSB levels repeatedly rebound following treatment.
From a methodological point of view, conjugated bilirubin is not a precise term. This is partly due to the fact that both monoglucuronides and diglucuronides as well as other conjugates are produced.118 There are also several different methods available for bilirubin determinations, which include or exclude different bilirubin conjugates.118,119 However, in clinical use determination of so-called direct-reacting bilirubin is often used as a substitute for conjugated bilirubin. When obtaining separate values for UCB and conjugated bilirubin is desirable, the value for direct-reacting bilirubin (conjugated bilirubin) is subtracted from the total bilirubin to give the value for UCB. However, this is also not very precise, since the delta fraction of UCB will be included in the measurement of direct-reacting bilirubin.120 Thus, even in a strictly unconjugated hyperbilirubinemia, a significant fraction of the TSB will be determined as conjugated bilirubin. Studies in newborn infants with erythroblastosis indicate that a direct-reacting fraction of 5–10% of the TSB should be considered normal, and not indicative of any increase in conjugated bilirubin in the sample.118 Situations in which additional laboratory studies may be indicated are further discussed in section “Jaundice as a Sign of Disease.”
Bilirubin toxicity is related to the nonalbumin-bound or “free” bilirubin. Since several physiological substances and conditions as well as drugs might displace bilirubin from albumin,33,34,121 the concentration of unbound bilirubin often does not correspond to TSB.28,122,123 The concentration of unbound bilirubin is, however, rarely measured in the clinical setting. This is mainly due to methodology not suited for routine analysis. An important step in improving treatment indications and the prevention of bilirubin toxicity in the newborn would be to develop a methodology suitable for routine clinical use.
When an infant is about to be discharged, the American Academy of Pediatrics (AAP) recommends a risk assessment as far as development of jaundice.107 This may consist of a measurement of TcB or TSB, with plotting of the TcB/TSB value on a nomogram for hour-specific values. The nomogram recommended by the AAP is shown in Figure 2-8. An infant whose hour-specific bilirubin value is in the low-risk zone of the nomogram is at low risk of developing severe jaundice after discharge. Recently published Norwegian guidelines recommend that all infants discharged before 24–48 hours of age should be screened with measurement of TcB or TSB.117 Infants with a TSB less than 1.8 mg/dL below treatment indication should be seen again. In Norway this is usually done in connection with return to the hospital for the routine metabolic screen at 60–72 hours of age.124 Parents of infants with identified risk factors for hyperbilirubinemia (large cephalhematoma, previous sibling treated for jaundice, late preterm infants, Asian ethnicity) should be given written information about jaundice in the newborn as well as oral and written information to return to the maternity ward if the infant becomes more jaundiced and/or feeds poorly. When parents call on the phone with such problems, the situation should not be discussed over the phone, but be told that the infant needs to be seen at the hospital immediately.
The most common cause of prolonged jaundice in newborn infants is probably so-called breast milk–associated jaundice.125 In the absence of specific diagnostic tests, the diagnosis of breast milk–associated jaundice can only be presumptive, and more serious conditions need to be ruled out, as discussed in section “Jaundice as a Sign of Disease.” Follow-up must be close with careful instruction of the parents. Interruption of breastfeeding for 24–48 hours and/or feeding with breast milk substitutes may help to reduce the TSB level. Supplementing breast milk feeds with 5 mL of a breast milk substitute also reduces the level and duration of jaundice in breast milk–fed infants.95 Because this latter intervention causes less interference with the establishment of the breastfeeding dyad, this approach tends to be our first step.
When jaundice continues beyond the first 2 weeks of life, obtaining the lactation/nutrition history is a first priority since hypogalactia and dehydration of the infant may play a role. The infant’s weight curve should be evaluated. Delay to regain birth weight may be associated with prolongation of jaundice through increased enterohepatic circulation of bilirubin. The mother’s impressions as far as adequacy of her breastfeeding may be erroneous, particularly in primiparous women.
The family history should also be explored. The risk of breast milk jaundice appears to be increased in infants who have genetic polymorphisms in the coding sequences of the UGT1A1 or OATP2 genes; prolonged and/or pronounced jaundice in older siblings may also be significant, and may suggest Gilbert syndrome.126 The result of the newborn metabolic screening for congenital hypothyroidism and galactosemia, if that condition is included in the screening program, should be checked. A gray/whitish stool may suggest intrahepatic or extrahepatic biliary atresia. The threshold should be low for ordering supplementary studies as outlined in section “Jaundice as a Sign of Disease.”
An epidemic of kernicterus, which occurred in the 1950s when sulfonamides were used for infection prophylaxis in newborn nurseries, led to the recognition that kernicterus in these infants was caused by the drug displacing bilirubin from its binding to albumin.127 Since then, several other drugs have also been shown to compete with bilirubin for the binding site on albumin.128,129 Some stabilizers used with IV drugs may also cause bilirubin displacement.130,131 Therefore, all drugs to be used in sick infants should be tested for their ability to displace bilirubin from its binding to albumin; any drug that has this characteristic must be avoided, at least as long as TSB levels remain significantly elevated.
Recent data on ibuprofen, a drug used to close the ductus arteriosus in preterm infants and approved as an orphan drug on the European market for this purpose, provide worrisome evidence that bilirubin displacement is not taken seriously enough at present. Thus, ibuprofen may be a competitive displacer of bilirubin in vitro and should therefore be used with caution in premature infants with significant hyperbilirubinemia.132–134 Soligard et al. found that ibuprofen showed a competitive displacement of bilirubin from albumin and increased the dissociation constant of the bilirubin–albumin complex from 3.9 × 10-8 to 9.9 × 10-8 M.134
Theoretically, free fatty acids have the ability to displace bilirubin from albumin.135 For this reason, there has been some concern regarding the use of lipid solutions in total parental nutrition. When lipid doses are less than 1 g/kg, the effect is not significant,136 and it may even enhance the effect of phototherapy.137
More recent data have further suggested that some drugs used in jaundiced infants, and which do not affect bilirubin binding, may nevertheless increase the risk of bilirubin neurotoxicity. Posphoglycoprotein P (PGP) may play a role in restricting the entry of bilirubin into the brain.138,139 PGP, when expressed in cancer cells, limits the entry of several cytostatic drugs into these cells, and seems to confer increased resistance to such drugs.140–142 The fact that PGP function may be inhibited or downregulated by a number of commonly used drugs has therefore excited interest among oncologists. Similarly, drugs that are used in the neonate, which are tested for bilirubin displacement, may also need to be tested for their effect on PGP. One important example is the drug ceftriaxone, which is both a PGP inhibitor and a bilirubin displacer; it is therefore particularly potent for increasing bilirubin entry into the brain (Figure 5-5).138 This issue clearly requires further research.
Figure 5-5.

Brain bilirubin and posphoglycoprotein P. Brain bilirubin values from rats given an IV bolus of bilirubin after administration of verapamil (a PGP inhibitor) or ceftriaxone (both a PGP inhibitor and a bilirubin displacer). (*) P < .05 compared with controls; (#) P < .05 compared with verapamil. (Based on data recalculated from Hankø.138)
Herbal medicines are commonly used in some countries and population groups. Yin et al. studied the impact of yin zhi huang, a decoction of four plants, which is widely used in Asia to treat neonatal jaundice, on induction of hepatic drug and bilirubin-metabolizing enzymes in rats.145 The effect of the individual constituents of the decoction was compared with that of phenobarbital, a well-known inducer. Artemisia, Rheum, and phenobarbital increased UGT activity. Phenobarbital was the most effective inducer of GST activity. Both phenobarbital and gardenia induced delta 5-3-ketosteroid isomerase activity, a marker for the Ya subunit of GST, responsible for intracellular bilirubin transport in liver. Thus, the possibility that there may be some benefits in treating neonatal jaundice with these plant extracts cannot be ruled out, although safety studies would clearly be required.
On the other hand, Yeung et al.146 studied Chinese goldthread (Coptis chinensis), also commonly given to newborn infants in China. This herb, which is taken as a tea, was shown to have a significant effect in displacing bilirubin from its albumin binding. The authors strongly caution against the use of this herb in southern Chinese neonates, where neonatal jaundice is very common. Other Chinese herbal drugs (purified constituents LZX-A, QTJ, YHS, and SQZG) were, however, without displacing properties.147
While it is well established that drug therapy in jaundiced infants requires caution because of the risk of both increased brain bilirubin entry secondary to binding competition and inhibition of membrane pumps, the question of whether jaundice can affect drug therapy has not been well studied. Although it has been shown that significant jaundice (TSB >11.7 mg/dL) can delay gastric emptying,148 whether this has any impact on the absorption or metabolism of drugs administered enterally can at present only be speculated. Similarly, the possible effect on drug absorption of the secretory diarrhea observed in jaundiced infants receiving phototherapy149 appears not to have been studied. The normal increase in blood flow to the bowel seen postprandially in infants is blunted during phototherapy for neonatal jaundice.150 This might also theoretically influence the absorption of drugs given by mouth, but no studies addressing this question appear to be on record. Finally, when drugs and bilirubin compete for binding to serum albumin, depending on the specificity and competitive nature of binding, conceivably free drug concentrations might be increased.
After more than 50 years of research into the mechanisms of bilirubin-induced neurological dysfunction (BIND), the specific nature of this phenomenon remains elusive.151 Experimentally, bilirubin has diverse toxic, intracellular effects, which in vitro have appeared to be mainly inhibitory. It has been suggested that the diversity of effects might be understood in terms of inhibition of a common regulatory mechanism such as protein phosphorylation.151 However, McDonagh has recently proposed that bilirubin may be a so-called promiscuous inhibitor, cautioning that effects observed in vitro may be nonspecific and not related to in vivo mechanism(s) of toxicity.152 “Promiscuous inhibitors” have high hydrophobicity, high molecular flexibility, and the ability to form microaggregates, properties also shared by bilirubin. For a more detailed discussion of these vexing problems, please see Chapter 7.
For bilirubin to affect the brain, it must gain entry. Different conditions and mechanisms for bilirubin entry into the brain are shown in Figure 5-6. The chemistry and solubility characteristics of bilirubin may to some extent explain how this happens. The main bilirubin isomer, bilirubin-IXα (Z,Z), may occur as a charged dianion or as bilirubin acid. The dianion has some water solubility at neutral pH, whereas the acid, due to intramolecular hydrogen bonds, is nearly insoluble in water.14,23 It has been believed that the hydrophobic isomer is responsible for the toxic effects, while the water-soluble isomers are thought to be nontoxic.153,154 However, there are weaknesses in the experimental approaches to these questions, and the issue cannot be said to be fully resolved.
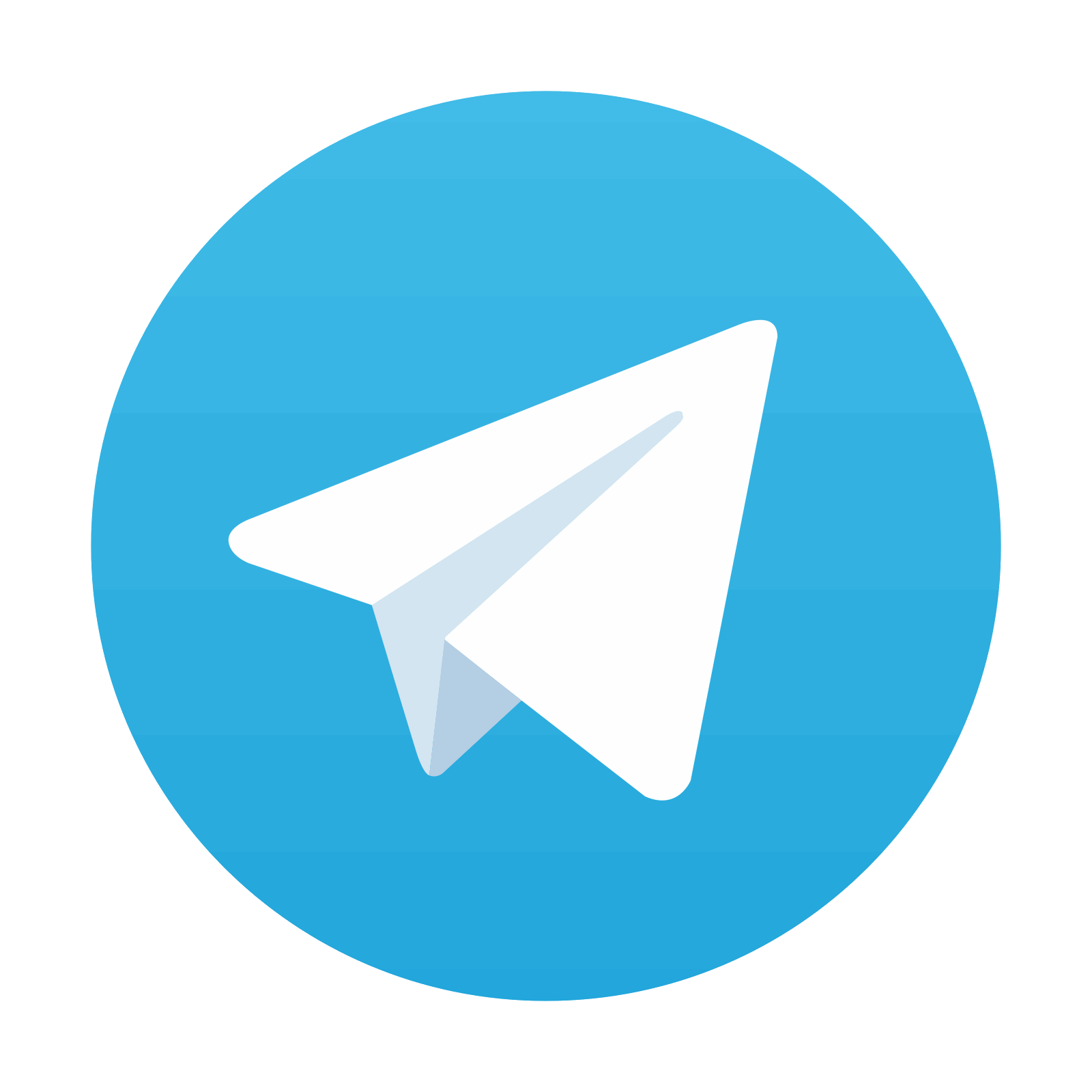
Stay updated, free articles. Join our Telegram channel

Full access? Get Clinical Tree
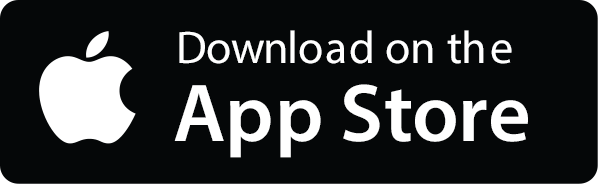
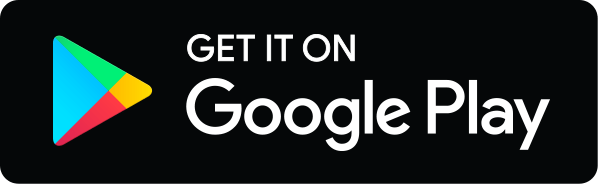