We owe the development of clinical phototherapy to an astute observation made, more than 50 years ago, by Sister J. Ward, the nurse in charge of the premature baby unit at the former Rochford General Hospital in Essex, England.1 As described by Dobbs and Cremer, Sister Ward recognized the value of sunshine and fresh air to all, including premature babies, and she would take the “more delicate infants out into the courtyard, sincerely convinced that the combination of fresh air and sunshine would do them much more good than the stuffy, overheated atmosphere of the incubator.”1 During a ward round in 1956, Sister Ward showed the pediatricians a jaundiced, premature infant who appeared pale yellow except for a triangle of skin that was much yellower than the rest of the body. Apparently, a corner of the sheet had covered this part of the baby and the Sister recognized that the rest of the baby had been “bleached” by the sun. (It was only recognized more than a decade later that the mechanism for the bleaching of yellow serum and skin—photooxidation—probably plays a minor role in the reduction of the serum bilirubin concentration in newborns treated with phototherapy.)2,3
A few weeks later, in the same nursery, a tube of blood, inadvertently exposed to sunlight for several hours, showed a total serum bilirubin (TSB) level of 10 mg/dL, much lower than anticipated.1 This led to the hypothesis that visible light could affect serum bilirubin levels in vivo and the concept of using phototherapy as a clinical tool was born.4 Cremer et al. then showed that exposing jaundiced infants either to sunlight or to blue fluorescent lights effectively lowered their bilirubin levels.4 Although the observations of Cremer et al. were published in 1958 and confirmed in multiple subsequent publications in Europe and Central and South America, it was not until Lucey et al. published their findings in 19685 that this simple, apparently safe, and effective treatment for hyperbilirubinemia achieved widespread acceptance in the United States and phototherapy is now used worldwide. Although there exists a vast body of literature from human, animal, and laboratory investigation dealing with the mechanism of action, biological effects, complications, and clinical use of phototherapy, there is a need for additional information on how phototherapy works, how its dosage should be measured, and how it should be administered most effectively. Jährig et al. provide a reference source on phototherapy data up to 19936 and the recent, remarkably comprehensive and critical review by the National Institute for Health and Clinical Excellence (NICE) in the United Kingdom is an excellent resource for additional reading.7
Phototherapy is a mechanism for detoxifying bilirubin and lowering the TSB level. It achieves this by using light energy to change the shape and structure of bilirubin, converting it to molecules that can be excreted even when normal conjugation is deficient (Figures 10-1 and 10-2).3,8 Absorption of light by dermal and subcutaneous bilirubin induces a fraction of the pigment to undergo several photochemical reactions that occur at different rates. These reactions generate yellow stereoisomers of bilirubin and colorless derivates of lower molecular weight (Figure 10-2). These products are less lipophilic than bilirubin, they have less internal hydrogen bonding, and, unlike bilirubin, they can be excreted in bile or urine without the need for conjugation. The relative contributions of the various reactions to the overall elimination of bilirubin are unknown, although in vitro and in vivo studies suggest that photoisomerization is more important than photodegradation.2 Bilirubin elimination depends on the rate of bilirubin formation as well as the rates of clearance of the photoproducts. Photoisomerization occurs rapidly during phototherapy, and some isomers appear in the blood long before the level of plasma bilirubin begins to decline.8–10
Figure 10-1.

Normal bilirubin metabolism and bilirubin metabolism during phototherapy. In normal metabolism, lipophilic bilirubin, which results predominantly from the catabolism of red cells, circulates in blood mainly as a noncovalent conjugate with serum albumin. After uptake by the liver, it is converted into two isomeric monoglucuronides and a diglucuronide (direct bilirubin) by the enzyme uridine diphosphoglucuronosyltransferase 1A1 (UGT1A1). The water-soluble glucuronides are excreted in bile with the aid of a canalicular multidrug resistance-associated transport protein, MRP2. Without glucuronidation, bilirubin cannot be excreted in bile or urine. In neonates, hepatic UGT1A1 activity is deficient and the lifetime of red cells is shorter than in adults, leading to accumulation and increased formation of bilirubin, with eventual jaundice. Phototherapy converts bilirubin to yellow photoisomers and colorless oxidation products that are less lipophilic than bilirubin and do not require hepatic conjugation for excretion. Photoisomers are excreted mainly in bile, and oxidation products predominantly in urine. (Reproduced from Maisels MJ, McDonagh AF. Phototherapy for neonatal jaundice. N Engl J Med. 2008;358:920–928, with permission.)
Figure 10-2.

Mechanism of phototherapy. The absorption of light by the normal form of bilirubin (4Z,15Z-bilirubin) generates transient excited-state bilirubin molecules. These fleeting intermediates can react with oxygen to produce colorless products of lower molecular weight, or they can undergo rearrangement to become structural isomers (lumirubins) or isomers in which the configuration of at least one of the two Z-configuration double bonds has changed to an E configuration. (Z and E, from the German zusammen [together] and entgegen [opposite], respectively, are prefixes used for designating the stereochemistry around a double bond. The prefixes 4 and 15 designate double-bond positions.) Only the two principal photoisomers formed in humans are shown. Configurational isomerization is reversible and much faster than structural isomerization, which is irreversible. Both occur much more quickly than photooxidation. The photoisomers are less lipophilic than the 4Z,5Z form of bilirubin and can be excreted unchanged in bile without undergoing glucuronidation. Lumirubin isomers can also be excreted in urine. Photooxidation products are excreted mainly in urine. Once in bile, configurational isomers revert spontaneously to the natural 4Z,15Z form of bilirubin. The graph, a high-performance liquid chromatogram of serum from an infant undergoing phototherapy, shows the presence of several photoisomers in addition to the 4Z,15Z isomer. Photoisomers are also detectable in the blood of healthy adults after sunbathing. (Reproduced from Maisels MJ, McDonagh AF. Phototherapy for neonatal jaundice. N Engl J Med. 2008;358:920–928, with permission.)
There are four possible configurational isomers of bilirubin (Figure 10-3). In infants receiving phototherapy the stable 4Z,15Z isomer is converted predominantly to the 4Z,15E isomer (Figure 10-2) but why this particular isomer is favored is not understood.8,11 The formation of 4Z,15E-bilirubin is spontaneously reversible in the dark and this occurs rapidly in bile. Thus, the 4Z,15E-bilirubin formed in the skin and excreted by the liver is readily converted back to unconjugated bilirubin, some of which can be reabsorbed through the gut.
When phototherapy is initiated, photoisomerization occurs almost instantaneously,8 and is detectable in the blood of newborns, within 15 minutes10 (Figure 10-4), but the clearance of the light-generated 4Z,15E isomer is slow (T1/2 ˜ 15 hours). Although the concentration of this isomer can account for 20–30% of the total unconjugated bilirubin,10,12 it might play only a minor role in lowering the serum bilirubin concentration.13 After 2 hours of phototherapy, ˜20% of the TSB is in the form of the 4Z,15E photoisomer and small amounts of 4Z,15E-bilirubin are present even before phototherapy begins, presumably as a result of exposure of the infants to ambient light. This is not surprising, as 4Z,15E-bilirubin is seen in nonjaundiced adults exposed to daylight.14 Clearance of the 4E,15Z and 4E,15E isomers, which do not accumulate appreciably in serum, is faster than that of 4Z,15E and these isomers may play a greater role in accelerating bilirubin elimination during phototherapy than currently recognized.
Figure 10-4.

Formation of 4Z,15E-bilirubin. 4Z,15E-bilirubin is shown as a percentage (mean ± SD) of TSB (4Z,15E + 4Z,15Z) in 20 jaundiced infants treated with phototherapy (F = 79.06, P < .0001, one-way ANOVA). The difference from time 0 was significant from 15 minutes onward. (Reproduced from Mreihil K, McDonagh A, Nakstad B, Hansen TWR. Early isomerization of bilirubin in phototherapy of neonatal jaundice. Pediatr Res. 2010;67:656–659, with permission.)
Because the products of photoisomerization are less lipophilic than 4Z,15Z-bilirubin, they should be less likely to cross the blood–brain barrier and the fact that phototherapy rapidly converts 20–30% of circulating bilirubin to a less lipophilic and possibly less toxic isomer suggests that a benefit of phototherapy might be the partial detoxification of bilirubin even before it is eliminated.8,10
In this reaction (Figures 10-2 and 10-3), intramolecular cyclization of the bilirubin (an irreversible process) occurs in the presence of light to form a substance known as lumirubin that also forms Z and E isomers that can be excreted in bile and in urine.13 During phototherapy, the serum concentration of lumirubin can reach about 2–6% of the TSB, much lower than the concentration of the configurational isomers that account for approximately 20–30% of the TSB10,12 but, because lumirubins are cleared from the serum much more rapidly than the 4Z,5E isomer, it has been suggested that lumirubin formation is mainly responsible for the phototherapy-induced decline in serum bilirubin in the human infant.13 However, the relative contributions of individual photoisomers are not known with any certainty.15
Bilirubin can be photooxidized to water-soluble, colorless products that can be excreted in the urine (Figure 10-2). This is a slow process and is probably only a minor contributor to the overall elimination of bilirubin during phototherapy.
The spectrum of light delivered by a phototherapy unit is determined by the type of light source and any filters used. Unconjugated bilirubin in tissues absorbs light most strongly in the blue region of the spectrum, near 460 nm, and the penetration of tissue by light increases markedly with increasing wavelength (Figure 10-5). The rate of formation of bilirubin photoproducts is highly dependent on the light intensity in the wavelengths used—only wavelengths that penetrate tissue and are absorbed by bilirubin have a phototherapeutic effect. There is a common misconception that ultraviolet (UV) light (<400 nm) is used for phototherapy. Phototherapy lights in current use do not emit significant erythemal UV radiation. In addition, the plastic cover of the lamp and, in the case of preterm infants, the incubator all filter out UV light.
Figure 10-5.

Important factors in the efficacy of phototherapy. The absorbance spectrum of bilirubin bound to human serum albumin (white line) is shown superimposed on the spectrum of visible light. Clearly, blue light is most effective for phototherapy, but because the transmittance of skin increases with increasing wavelength, the best wavelengths to use are probably in the range of 460–490 nm. Term and near-term infants should be treated in a bassinet, not an incubator, to allow the light source to be brought to within 10–15 cm of the infant (except when halogen or tungsten lights are used), increasing irradiance and efficacy. For intensive phototherapy, an auxiliary light source (light-emitting diode [LED] mattress, LED/fiber-optic pad, or special blue fluorescent tubes) can be placed below the infant or bassinet. If the infant is in an incubator, the light rays should be perpendicular to the surface of the incubator in order to minimize loss of efficacy due to reflectance. (Redrawn from Maisels MJ, McDonagh AF. Phototherapy for neonatal jaundice. N Engl J Med. 2008;358:920–928, with permission.)
Irradiance is the radiant power incident on a surface per unit area of the surface and the irradiance in a specific wavelength band is called the spectral irradiance and is expressed as μW/cm2 per nm (Table 10-1). Although there is generally a direct relationship between the efficacy of phototherapy and the irradiance used16 (Figure 10-6), the concentration of plasma bilirubin at any given time depends on a number of complex kinetic factors: the rate of formation of bilirubin, the rates of elimination of the photoisomers, the rates of migration of bilirubin and the individual photoisomers into and out of the blood, and the rate of reabsorption of bilirubin from the intestine into the blood. Clearly, some of these rates are independent of the irradiance.
Quantity | Dimensions | Usual Units of Measure |
---|---|---|
Irradiance (radiant power incident on a surface per unit area of the surface) | W/m2 | W/cm2 |
Spectral irradiance (irradiance in a certain wavelength band) | μW/m2 per nm | μW/cm2 per nm |
Spectral power (average spectral irradiance across a surface area) | W/nm | mW/nm |
Figure 10-6.

Relationship between average spectral irradiance and decrease in total serum bilirubin concentration. Full-term infants with nonhemolytic hyperbilirubinemia were exposed to special blue lights (Phillips TL 52/20W) of different intensities. Spectral irradiance was measured as the average of readings at the head, trunk, and knees. Drawn from the data of Tan.16 (Reproduced from Maisels MJ. Why use homeopathic doses of phototherapy? Pediatrics. 1996;98:283–287, with permission. Copyright 1996 by the American Academy of Pediatrics.)
The data in Figure 10-6 suggest that there is a saturation point beyond which an increase in the irradiance produces no added efficacy. However, for technical reasons, the data in Figure 10-6 were obtained not by gradually increasing the intensity of an array of identical light sources, but by switching between different combinations (and configurations?) of two different light sources that, experimentally, can introduce some uncertainty. Presently it is unknown that a saturation point exists. As the conversion of bilirubin to excretable products is partly irreversible and follows first-order kinetics, there may not be a saturation point and we do not know the maximum and safe effective dose of phototherapy.
This is the product of the skin surface irradiance and spectral irradiance across the surface area. Because both irradiance and the surface area of the infant exposed to phototherapy are key elements in determining the efficacy of phototherapy, the use of spectral power is the only meaningful way of comparing the dose of phototherapy received by infants under different phototherapy systems.17
Figure 10-7 shows that the light intensity (measured as spectral irradiance) is inversely related to the distance from the source.17 The relationship between intensity and distance is almost (but not quite) linear, indicating that these data do not obey the law of inverse squares, which states that the light intensity will decrease with the square of the distance. That law only applies to a point source of light and phototherapy units do not provide a point source of light—the light source has features of both a cylindrical and a planar source. Thus, the light intensity is a function of the distance but does not vary with the square of the distance. Figure 10-7 also demonstrates the dramatic difference in the irradiance produced within the 425- to 475-nm band by different types of fluorescent tubes. Table 10-2 lists the factors that affect the dose and efficacy of phototherapy.
Figure 10-7.

Effect of light source and distance from the light source to the infant on average spectral irradiance. Measurements were made across the 425- to 475-nm band using a commercial radiometer (Olympic Bilimeter Mark II). The phototherapy unit was fitted with eight 24-in fluorescent tubes. (■) Special blue, General Electric 20-W F20T12/BB tube; (♦) blue, General Electric 20-W F20T12/B tube; (▲) daylight blue, four General Electric 20-W F20T12/B blue tubes and four Sylvania 20-W F20T12/D daylight tubes; and (•) daylight, Sylvania 20-W F20T12/D daylight tube. Curves were plotted using linear curve fitting (True Epistat; Epistat Services, Richardson, TX). The best fit is described by the equation y = A eBx. (Reproduced from Maisels MJ. Why use homeopathic doses of phototherapy? Pediatrics. 1996;98:283–287, with permission. Copyright 1996 by the American Academy of Pediatrics.)
Factor | Technical Terminology | Rationale | Clinical Application |
---|---|---|---|
Type of light source | Spectrum of light (nm) | Blue-green spectrum is most effective at lowering total serum bilirubin (TSB); light at this wavelength penetrates skin well and is absorbed strongly by bilirubin | Use special blue fluorescent tubes or light-emitting diodes (LED) or another light source with output in blue-green spectrum for intensive PT |
Distance of light source from patient | Spectral irradiance (a function of both distance and light source) delivered to surface of infant | ↑ irradiance leads to ↑ rate of decline in TSB. Standard PT units deliver 8–10 μW/cm2 per nm; intensive PT delivers ≥30 μW/cm2 per nm | If special blue fluorescent tubes are used, bring tubes as close as possible to infant to increase irradiance. (Do not do this with halogen lamps because of danger of burn.) Positioning special blue tubes 10–15 cm above infant will produce an irradiance of at least 35 μW/cm2 per nm |
Surface area exposed | Spectral power (a function of spectral irradiance and surface area) | ↑ surface area exposed leads to ↑ rate of decline in TSB | For intensive PT, expose maximum surface area of infant to PT. Place lights above and belowa or aroundb the infant. For maximum exposure, line sides of bassinet, warmer bed, or incubator with aluminum foil or white material |
Cause of jaundice | PT is likely to be less effective if jaundice is caused by hemolysis or if cholestasis is present (direct bilirubin is increased) | When hemolysis is present, start PT at a lower TSB level and use intensive PT. Failure of PT suggests hemolysis is cause of the jaundice. When direct bilirubin is elevated, watch for bronze baby syndrome or blistering | |
TSB level at start of PT | The higher the TSB, the more rapid the decline in TSB with PT | Use intensive PT for higher TSB levels. Anticipate a more rapid decrease in TSB with higher TSB levels |
Since the only effective alternative to phototherapy in infants with significant hyperbilirubinemia is exchange transfusion, one measure of the efficacy of phototherapy is the dramatic reduction in the number of exchange transfusions being performed. This effect has been particularly noticeable in very low birth weight (VLBW) infants, for whom exchange transfusions, once common procedures in the neonatal intensive care unit (NICU), are now rare.18–20 In the first National Institutes of Child Health and Human Development (NICHD) randomized controlled trial of phototherapy,21 control infants did not receive phototherapy and were subject to exchange transfusions if their bilirubin levels increased sufficiently. In this group, 36% of infants with birth weights <1500 g required an exchange transfusion. When phototherapy was used in a similar population, only 2 of 833 infants (0.24%) received exchange transfusions.22 In the recent Neonatal Research Network (NRN) study, only 5/1974 (0.25%) infants with birth weights ≤1000 g required an exchange transfusion.18 In Northern California Kaiser Permanente hospitals, only 1/130 term and late preterm infants with a TSB between 25 and 29.9 mg/dL received an exchange transfusion.23
The factors that influence the dose and efficacy of phototherapy are listed in Table 10-2, and illustrated in Figure 10-5. There is a clear dose–response relationship between the irradiance (energy output of the phototherapy device) delivered to the infant and the rate of decline in the TSB (Figure 10-6).16 It is, therefore, important to perform regular measurements of the irradiance with a radiometer or spectroradiometer. Phototherapy units that deliver between 30–40 μW/cm2 per nm in the 430- to 490-nm band have been found to be effective in clinical studies.
In general, none of the light sources used for phototherapy were designed specifically for that purpose, except for special blue fluorescent bulbs for which a modified phosphor was developed. In the first phototherapy unit, the light source was commercially available standard blue fluorescent tubes.4 Over the next decade, daylight or cool-white fluorescent tubes were frequently used prior to the introduction of tungsten and halogen spotlights. Most of the radiation emitted by tungsten and halogen light sources is not therapeutically effective with respect to phototherapy. Lights that deliver irradiance predominately in the blue spectrum (460–490 nm) are desirable because at these wavelengths there is adequate skin penetration and absorption of the light by dermal and subcutaneous bilirubin (Figure 10-5). Today, there is a wide spectrum of phototherapy units including those that are built-in components of radiant warmer systems. Special blue fluorescent tubes are effective because they provide light predominantly in the blue spectrum. The imprint F20-T12/BB (General Electric, Westinghouse, Sylvania) or TL52/20W (Philips, Eindhoven, the Netherlands) is found on special blue tubes. Note that these are different from regular blue tubes (labeled F20-T12/B),13 which put out significantly less irradiance than the special blue tubes (Figure 10-7). Special blue tubes do impart a bluish tinge to an infant and there have been concerns that this can induce nausea in caregivers and that it might obscure cyanosis, although we have never encountered these problems in our nursery. Full-term or late preterm hyperbilirubinemic newborns, who are otherwise healthy and require phototherapy, are very unlikely to be cyanotic, and in the NICU, pulse oximetry identifies abnormal oxygen saturations. The blue color of the lights sometimes leads to the misconception that UV light is being used.
A wide variety of new phototherapy devices has been marketed in the last several years,24,25 and some of the commercial suppliers as well as some irradiance measurements from different devices are provided in Table 10-3 and the footnotes to Table 10-2. Halogen and tungsten lamps have the advantage of being more compact than fluorescent systems but, unlike fluorescent lamps, they cannot be brought close to the infant (to increase the irradiance) without incurring the risk of a burn. In addition, the surface area covered by most of these lamps is small25 and much of the energy output of the lamps is not in an effective region of the spectrum.
Footprint Irradiance (μW/cm2 per nm)b | ||||||||
---|---|---|---|---|---|---|---|---|
Halogen/Fiber-Optic | Fluorescent | LED | ||||||
Wallaby (Neo)c | Sunlight @ Zenith on 8/31/05 | |||||||
Light Meter | BiliBlanketb | IIc | IIIc | PEP Bedd | Martin/Philips BBe | neoBLUEf | PortaBedg | |
(Range, Peaka) | @ Contact | @ Contact | @ 10 cm | @ 25 cm | @ 30 cm | @ 10 cm | Level Ground | |
BiliBlanket Meter IIb (400–520, 450 nm) | 34 | 28 | 34 | 40 | 69 | 34 | 76 | 144 |
Bili-Meter, Model 22f (425–475, 460 nm) | 29 | 16 | 32 | 49 | 100 | 25 | 86 | 65h |
Joey Dosimeter, JD-100c (420–550, 470 nm) | 53 | 51 | 60 | 88 | 174 | 84 | 195 | 304h |
PMA-2123 Bilirubin Detectori (400–520, 460 nm) | 24 | 24 | 37 | 35 | 70 | 38 | 73 | 81 |
GoldiLux UVA Photometer, GRP-1j,k (315–400, 365 nm) | <0.04 | <0.04 | <0.04 | <0.04 | <0.04 | <0.04 | <0.04 | 2489 |
Fiber-optic systems have found widespread use and provide a convenient way to deliver home phototherapy or double phototherapy when it is necessary to expose more of the infant’s surface area. Earlier halogen/fiber-optic systems provided relatively small pads; with a larger pad the light is distributed over a greater surface area, thus reducing the irradiance. This problem has recently been addressed by combining fiber-optic and light-emitting diode (LED) technology (currently marketed as the BiliSoft, GE Healthcare, Wauwatosa, WI, and neoBLUE Blanket, Natus Medical, Inc, San Carlos, CA) in which an LED light source is conducted down a fiber-optic bundle to a pad or blanket. The pad sizes with these newer devices are adequate to cover the full dorsal or ventral surface of a preterm or term infant and the high intensity of the LED light source provides adequate irradiance over an appropriate surface area.
LED light sources are becoming widely used. These high-intensity, gallium nitride LEDs provide a high irradiance, in whichever spectrum is chosen, with virtually no heat generation.26 A randomized controlled trial comparing LED phototherapy with special blue fluorescent lamps (at similar irradiance levels) showed that the two systems were equally effective in lowering the TSB level.27
In the United States, phototherapy was initially used in low birth weight (LBW) and term infants primarily to prevent slowly rising TSB levels from reaching levels that might require an exchange transfusion5 and this is how phototherapy is used today in the NICU. But many term and late preterm infants have been discharged and subsequently readmitted for treatment of TSB levels of 20 mg/dL or more. In these infants, therapeutic rather than prophylactic phototherapy is indicated and a full therapeutic dose of phototherapy (now termed intensive phototherapy)28 is recommended to reduce the bilirubin level as soon as possible.17,29 By choosing the appropriate light spectrum and maximizing the irradiance and the surface area of the infant exposed to phototherapy, the best therapeutic effect can be achieved. If special blue fluorescent tubes are used, the infant should be placed in a bassinette and not an incubator, because the top of the incubator prevents the light from being brought sufficiently close to the infant.17 In a bassinette it is possible to bring the fluorescent lights within about 10 cm of the infant and achieve a spectral irradiance of more than 50 μW/cm2 per nm (Figures 10-5 and 10-7). The small amount of heat produced by the fluorescent lamps maintains a normal body temperature for these infants (naked except for a diaper). One disadvantage of LED lights is that they emit almost no heat so that a naked infant in a bassinette might become cool if the only source of heat is the LED phototherapy light. Note that halogen and tungsten phototherapy lamps cannot be positioned closer to the infant than recommended by the manufacturer without incurring the risk of a burn.
Increasing the surface area exposed to phototherapy is easily achieved by placing a fiber-optic/LED blanket or mattress underneath the infant, or using a system that has special blue fluorescent tubes below the baby (Table 10-2). It is not surprising that this type of “double phototherapy” is approximately twice as effective as single phototherapy in LBW infants and almost 50% better in full-term infants.30,31 A simple way of further increasing the surface area exposed is to place some reflecting material (a white sheet or aluminum foil) around the inner surface of the bassinette or incubator. In France, the Mediprema Cradle 360 (Mediprema, Tours Cedex, France) provides 360° exposure to special blue fluorescent light and delivers highly effective phototherapy,32 but overheating may limit the duration of phototherapy that can be used with this device.
Spectral irradiance can be measured with a spectroradiometer, a precision instrument that measures the flux of light over a series of discrete wavelengths. Clinicians and the manufacturers of phototherapy units usually use standard radiometers to measure the irradiance. These radiometers are relatively inexpensive and easy to operate but, unlike spectroradiometers, they take only a single measurement across a band of wavelengths—typically 425–475 or 400–480 nm. These wavelength bands are chosen because they represent the wavelengths at which bilirubin absorbs light maximally and will therefore undergo photochemical reactions to form excretable isomers and breakdown products. Commercial radiometers measure the irradiance in a predetermined band but display the results as the spectral irradiance (μW/cm2 per nm). Examples of instruments commercially available can be found in Table 10-3.25
As can be seen in Table 10-3, measurement of irradiance from the same phototherapy system using different radiometers produces widely divergent results.25 The irradiance also varies depending on where the measurement is taken. Irradiance measured below the center of the light source can be more than double that measured at the periphery of the exposed area and this drop-off at the periphery will vary with different phototherapy units. In clinical practice, average measurements are rarely used and the spectral irradiance levels provided in this chapter refer to measurements taken at the center of the exposed area of the infant or the device. Unfortunately, there is no standardized method for reporting phototherapy dosages in the clinical literature, so it is difficult to compare published studies of efficacy.
Clinical studies comparing intermittent with continuous phototherapy have produced conflicting results.33–35 In practice, trying to administer phototherapy with defined on and off times is probably more trouble than it is worth. Yet, it is rarely necessary for phototherapy to be continuous. It can, and certainly should, be interrupted during feeding or brief parental visits when eye patches are removed and normal parental bonding can occur. When TSB levels are very high, however, intensive phototherapy should be administered continuously until a satisfactory decline in the TSB has occurred.28
Because phototherapy acts on bilirubin that is present in the extravascular space as well as in the superficial capillaries, it has become a common practice to turn the infant at intervals supine to prone and back. Two randomized studies36,37 and one controlled trial38 found that turning infants every few hours did not improve the efficacy of phototherapy. For this and other reasons (back to sleep), it seems preferable for the infant to be supine during phototherapy.
Breastfed infants who are readmitted to the hospital with hyperbilirubinemia frequently also have excessive weight loss, largely as a result of poor caloric intake, although some of these infants are also dehydrated. In those in whom there is excessive weight loss and/or dehydration, it makes sense to provide supplemental calories and fluids using expressed breast milk, if available, or a milk-based formula and, if necessary, intravenous (IV) fluids. Because lumirubin photoisomers are excreted in the urine, maintaining adequate hydration and good urine output should help to improve the efficacy of phototherapy.39 In a randomized controlled trial, 74 Indian infants with TSB levels >18 mg/dL and no documented hemolysis received special blue light phototherapy.40 In the group that received supplemental IV fluids 6/37 (16%) required an exchange transfusion versus 20/37 (54%) in the group that received breastfeeding or formula only (relative risk [RR]: 0.30; 95% confidence interval [CI]: 0.14–0.66). But in a Malaysian randomized trial,41 no difference was found in the number of infants requiring an exchange transfusion between groups that received oral or IV supplementation. The above reported incidence of exchange transfusions in an Indian population40 is much higher than currently experienced in western countries. In Northern California Kaiser Permanente hospitals, only 1/130 (0.8%) infants with TSB levels 25–29.9 mg/dL received an exchange transfusion.23 In our experience, if the infant is taking oral fluids and is not significantly dehydrated, IV therapy is not required and phototherapy with oral fluid supplementation is sufficient.
A detailed guideline for the use of phototherapy in infants of 35 or more weeks of gestation has been published by the American Academy of Pediatrics (AAP) (Figure 10-8) and has been widely adopted in the United States and elsewhere.46–48 Similar, consensus-based guidelines have been published recently in Canada, Israel, Norway, and the United Kingdomch10rf#7,ch10rf#46,ch10rf#49,ch10rf#50 and two of these guidelines include the management of infants ≤34 weeks of gestation.7,49Figure 10-9 shows the recommendations from Norway, which are based on birth weight. In the UK guideline,7 graphs are provided for each gestational age. Because solid data on which to base recommendations are lacking, all of these guidelines are, of necessity, consensus based. Most recently, Maisels et al. have offered suggestions for the management of hyperbilirubinemia in the preterm infant <35 weeks gestation51 (Table 10-4). The recommended treatment levels in this report are based on operational thresholds or therapeutic normal levels (a level beyond which a specific therapy is likely to do more good than harm).52 It would be ideal if all of these guidelines were the product of evidence-based estimates of when the benefit of intervention exceeds the risks and costs. Such estimates should come from randomized trials or high-quality, systematic observational studies, but such studies are rare.53,54 Treatment guidelines must, therefore, rely on relatively uncertain estimates of risks and benefits as well as the recognition that using a single TSB to predict long-term behavioral and developmental outcomes is not reliable and will lead to conflicting results.53,54
Figure 10-8.

Guidelines for phototherapy in hospitalized infants of 35 or more weeks gestation.
These guidelines refer to the use of intensive phototherapy, which should be used when the TSB exceeds the line indicated for each category. Infants are designated as “higher risk” because of the potential negative effects of the conditions listed on albumin binding of bilirubin,42–44 the blood–brain barrier,45 and the susceptibility of the brain cells to damage by bilirubin.45
Intensive phototherapy implies irradiance in the blue-green spectrum (wavelengths of approximately 430–490 nm) of at least 30 μW/cm2 per nm (measured at the infant’s skin directly below the center of the phototherapy unit) and delivered to as much of the infant’s surface area as possible. Note that irradiance measured below the center of the light source is much greater than that measured at the periphery. Measurements should be made with a radiometer specified by the manufacturer of the phototherapy system.
If the total serum bilirubin does not decrease or continues to rise in an infant who is receiving intensive phototherapy, this strongly suggests the presence of hemolysis.
(Reproduced from Maisels MJ. Why use homeopathic doses of phototherapy? Pediatrics. 1996;98:283–287. Copyright 1996 by the American Academy of Pediatrics.)
Gestational Age (Weeks) | Initiate Phototherapy (mg/dL) | Exchange Transfusion |
---|---|---|
<28 0/7 weeks | 5 to 6 | An exchange transfusion is recommended for infants <30 0/7 weeks if the TSB exceeds 12–13 mg/dL. For those ≥30 0/7 weeks, an exchange transfusion is recommended if, in spite of intensive phototherapy, TSB levels continue to rise and are 5 mg/dL above the levels listed. For all infants, an exchange transfusion is recommended if the infant shows signs of acute bilirubin encephalopathy (hypertonia, arching, retrocollis, opisthotonos, high-pitched cry), although it is recognized that these signs rarely occur in VLBW infants. |
28 0/7–29 6/7 weeks | 6 to 8 | |
30 0/7–31 6/7 weeks | 8 to 10 | |
32 0/7–33 6/7 weeks | 10 to 12 | |
34 0/7–34 6/7 weeks | 12 to 14 |
Although it is clear that phototherapy has played an important role in the dramatic reduction in the need for exchange transfusion, it has not been clearly established how many late preterm and term infants we need to treat with phototherapy (the “number needed to treat” [NNT]) in order to prevent one infant from requiring an exchange transfusion. In the published randomized trials,55–57 among infants without hemolysis, 6–10 infants needed to be treated with phototherapy to prevent 1 from developing a TSB ≥20 mg/dL.53 In those studies, however, the type of phototherapy used was much less effective than that used today,17,28 so the NNT could be lower. As the primary function of phototherapy is to prevent the need for an exchange transfusion, Newman et al. estimated the NNT58 among term and late preterm newborns in order to prevent one infant from reaching a TSB level that meets the AAP criteria for exchange transfusion.28 There was a remarkable range in the NNT among infants who did not have a positive direct antiglobulin test (DAT, Coombs’ test) (Table 10-5). For 36-week gestation boys, <24 hours old, the NNT was 10 (95% CI: 6–19) while for 41-week gestation girls who were ≥3 days old, it was 3041 (95% CI: 888–11,096). Phototherapy was less effective for infants with a positive DAT. For a 40-week gestation male infant who met criteria for phototherapy between 48 and 72 hours the NNT was 682 (95% CI: 367–1294). These numbers suggest that for infants ≥38 weeks who qualify for phototherapy between 48 and 72 hours a different approach might avoid the need for phototherapy entirely. Instead of routinely admitting these infants for treatment, other options could be considered, such as improved lactation support, providing formula supplementation,56 the use of home phototherapy (see below), or simply repeating the TSB after several hours when, in many cases, it might have decreased. If we need to treat 3000 infants with phototherapy to prevent one exchange transfusion, it is reasonable to ask whether, in many of these infants, phototherapy could have been avoided.
Gestational Age (Weeks) | Age at Qualifying TSB: <24 h | Age at Qualifying TSB: 24 to <48 h | Age at Qualifying TSB: 48 to <72 h | Age at Qualifying TSB ≥72 h |
---|---|---|---|---|
Boys | ||||
35 | 14 (7–40) | 26 (14–57) | 83 (36–190) | 171 (70–426) |
36 | 10 (6–19) | 19 (12–39) | 59 (31–101) | 122 (68–236) |
37 | 16 (10–28) | 29 (20–58) | 95 (52–168) | 196 (100–407) |
38 | 35 (14–100) | 67 (31–215) | 222 (107–502) | 460 (196–1,352) |
39 | 74 (31–244) | 142 (62–554) | 476 (197–1,385) | 989 (373–3,607) |
40 | 106 (44–256) | 204 (98–487) | 682 (367–1,294) | 1,419 (634–3,755) |
≥41 | 148 (54–428) | 284 (127–780) | 953 (366–3,017) | 1,983 (676–8,408) |
Girls | ||||
35 | 21 (12–49) | 40 (21–86) | 126 (50–267) | 261 (105–585) |
36 | 15 (11–26) | 28 (20–51) | 90 (43–146) | 186 (102–347) |
37 | 23 (16–39) | 44 (31–75) | 145 (73–243) | 300 (146–671) |
38 | 53 (23–134) | 102 (43–236) | 339 (154–730) | 705 (314–2,016) |
39 | 113 (58–342) | 217 (103–713) | 729 (272–1,730) | 1,516 (614–4,520) |
40 | 162 (75–400) | 312 (164–704) | 1,046 (491–2,136) | 2,176 (922–6,107) |
≥41 | 226 (92–702) | 435 (183–1140) | 1,461 (510–4,842) | 3,041 (888–11,096) |
As noted by Newman et al., the TSB level at which phototherapy should be done “depends on the NNT one is willing to accept.”58 But to determine this number, we need much more information regarding both the costs and potential adverse effects of phototherapy as well as a better way of knowing which TSB levels (or some other measurement) represent a threat to the infant’s well-being. “Unless we can quantify how bad an outcome is, it is hard to know how many people it is worth treating to prevent it.”58 In the NICHD NRN study of ELBW infants,18 the rate of neurodevelopmental impairment (NDI) was lower with aggressive phototherapy than with conservative therapy (26% vs. 30%, RR: 0.86; 95% CI: 0.74–0.99) (see below). Based on these data, the NNT with aggressive phototherapy, on average, to prevent one case of developmental impairment in infants with birth weights <1000 g was 25.
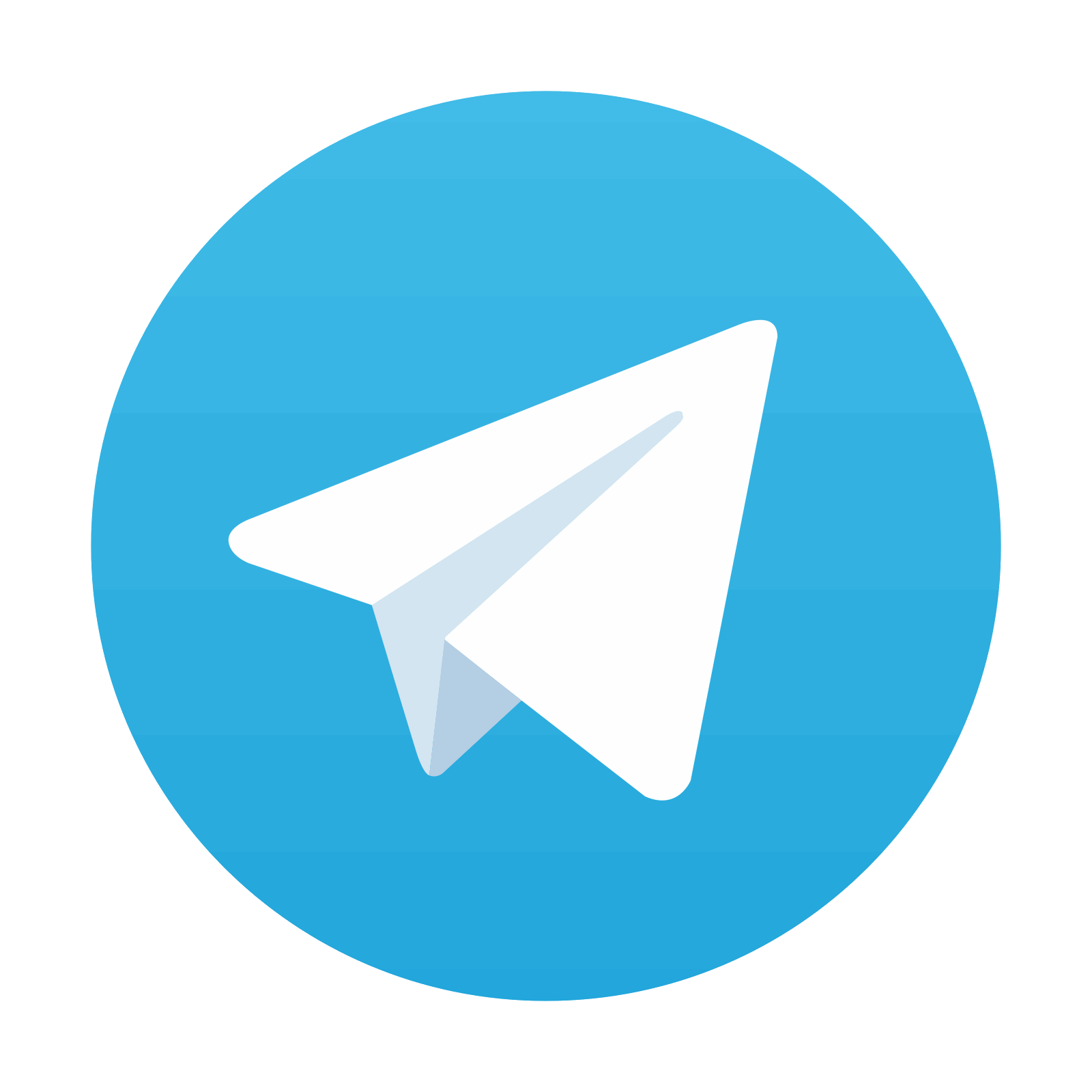
Stay updated, free articles. Join our Telegram channel

Full access? Get Clinical Tree
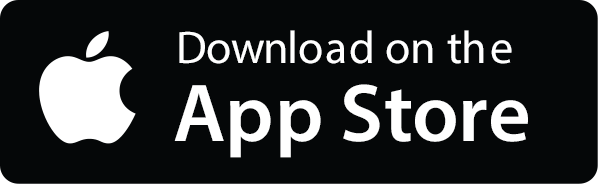
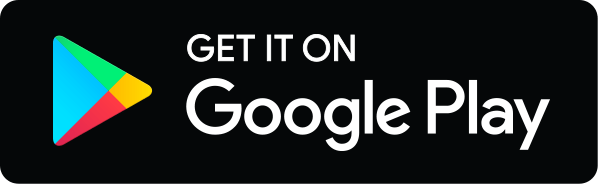