Pharmacologic Treatment of Neonatal Apnea
Girija Natarajan
Jose Maria Lopes
J. V. Aranda
Methylxanthines
Since the initial report in 1973 of a decrease in the frequency of apneic episodes in neonates given theophylline rectally, numerous studies have demonstrated the usefulness of the methylated xanthines in the treatment of neonatal apnea (1). Therefore, caffeine and theophylline have gained universal acceptance in the last few decades as first-line therapy of neonatal apnea.
Mechanism of Action
Several mechanisms appear to be involved in the decrease in apnea frequency seen after methylxanthine administration. These include the following:
Respiratory center stimulation.
Improvement in respiratory muscle contraction.
Others: altered sleep states, metabolic rate, cardiac output, metabolic homeostasis, and potentiation of catecholamine effect.
Respiratory Center Stimulation
Both caffeine and theophylline produce an increase in minute ventilation, a decrease in partial pressure of arterial CO2 (PaCO2), and an increase in most indices of neural respiratory drive. Davi et al. investigated the effect of theophylline on the control of breathing in newborn infants and found a decreased CO2 threshold and increased CO2 sensitivity (2). Gerhardt et al. observed a parallel shift in the slope of the CO2 response curve after aminophylline administration (3). In both newborn infants and in cats, caffeine has a potent effect on central neural drive (4,5). Caffeine increases mean inspiratory flow (tidal volume/inspiratory time) (Vt/Ti), the pressure generated after airway occlusion (p < .01), and minute ventilation. In the cat, when isocapnic conditions were maintained, ventilation was threefold greater, suggesting an interaction between caffeine and CO2. In the newborn baby, doses as low as 2.5 mg per kg of caffeine increase tidal volume. However, the optimal ventilatory response is observed only with doses of 10 mg per kg (6). The central respirogenic effect of the xanthines is further supported by the observation that they antagonize the depressant effects of narcotics such as codeine, morphine, and meperidine (7,8,9).
Improved Respiratory Muscle Function
The effect of caffeine and theophylline on muscle contraction has been known for many years (10). However, it was only in the last decade that the effects of these drugs were investigated in relation to respiratory muscle function. Several reports in the literature have described the effects of caffeine and theophylline on diaphragmatic contraction both in vivo and in vitro (11,12,13). Theophylline improves diaphragmatic efficiency and increases force production with electrical stimulation. The drug not only affected muscle contraction but also decreased the recovery time of fatigued muscles (14). In the newborn, fatigue of the respiratory muscles has been associated with apnea, which is effectively treated by xanthine administration (15,16,17). Therefore, it is possible that part of the anti-apneic effect of these drugs is due to improvement in respiratory muscle function (15,16,17).
Other Mechanisms
In addition to the increase in respiratory drive, increased CO2 sensitivity, and improvement in respiratory muscle contraction, other factors that may facilitate the action of the xanthines include increased neuromuscular transmission, catecholamine release, improved metabolic homeostasis, and changes in sleep states (18,19,20,21,22). The increase in metabolic rate and catecholamine levels after xanthine administration may lead to improved oxygenation and increased cardiac output. Improvement in metabolic homeostasis, such as increased blood glucose, may also lessen the frequency of apneic spells. A decrease in apnea frequency has been described at low doses of theophylline that do not alter ventilation or the CO2 response curve but
may impact the sleep–wake pattern. Increased neuromuscular transmission may lead to improved muscle tone, a well-known in vitro effect of the xanthines. Improved respiratory muscle tone has been related to increased functional residual capacity and better oxygenation in the newborn (23).
may impact the sleep–wake pattern. Increased neuromuscular transmission may lead to improved muscle tone, a well-known in vitro effect of the xanthines. Improved respiratory muscle tone has been related to increased functional residual capacity and better oxygenation in the newborn (23).
Adenosine Receptor Blockade
The methylxanthines appear to exert their effects by (a) blocking adenosine receptors A1 and A2a, (b) inhibition of phosphodiesterase with increased cyclic 3,5 adenosine monophosphate, and (c) translocation of intracellular calcium. Prostaglandin antagonism and upregulation of #979;-aminobutyric acid receptor A subunit expression (opposing the effect of hypoxia) have also been described (24,25). Both caffeine and theophylline are able to bind to adenosine receptors; adenosine is now recognized as a neurotransmitter or neuromodulator (21,22,26). Therefore, attention has been directed to the role of this particular neurotransmitter in the control of breathing. Adenosine and its analogues have potent inhibitory effects on respiration. Administration of L-phenylisopropyl adenosine, a stable adenosine analogue, causes respiratory depression in laboratory animals in a dose-dependent manner (27). This effect has been described in several species, including rat, rabbit, cat, and newborn piglet and in both the anesthetized (cat, rabbit, and piglet models) and the awake state (rat model) (28,29,30). The inhibition of respiration can be partially or completely reversed by the administration of theophylline and caffeine, both of which antagonize adenosine at the receptor level.
The role of intracerebral adenosine levels in the control of ventilatory response to hypoxia was explored in 15 spontaneously breathing piglets, 1 to 5 days old, sedated with chloral hydrate. Animals exposed to 12% oxygen showed a typical biphasic ventilatory response with an initial increase in ventilation followed by a late decrease. Both intravenous caffeine citrate (20 mg per kg) and inhaled CO2 separately and independently abolished or attenuated the late respiratory depression associated with hypoxia. In the same experiment, administration of dipyridamole, a competitive inhibitor of adenosine receptors, potentiated the ventilatory depression (30). These observations suggest that part of the efficacy of the xanthines in reducing apnea frequency in the neonate may be due to adenosine blockade with consequent central nervous system (CNS) stimulation.
Table 20.1 Pharmacokinetics of Theophylline and Caffeine Used in the Neonatal Period | ||||||||||||||||||||||||||||||||||||||||||||||||||||||||||||||||||
---|---|---|---|---|---|---|---|---|---|---|---|---|---|---|---|---|---|---|---|---|---|---|---|---|---|---|---|---|---|---|---|---|---|---|---|---|---|---|---|---|---|---|---|---|---|---|---|---|---|---|---|---|---|---|---|---|---|---|---|---|---|---|---|---|---|---|
|
Pharmacokinetics and Therapeutic Drug Monitoring
Several studies have shown that the plasma clearance and elimination of theophylline and caffeine are both prolonged in newborn babies compared with adults (31,32,33). The representative kinetic profiles of these two drugs are shown in Table 20.1. The obvious difference between the two drugs is the remarkably slow elimination of caffeine relative to theophylline. The plasma half-life is about 100 hours for caffeine and about 30 hours for theophylline. This difference in drug elimination indicates that caffeine can be given more sparingly (i.e., once daily), and that drug monitoring is probably not as crucial with caffeine as with theophylline. Caffeine half-life may be further prolonged in infants with cholestatic jaundice and breastfed infants (34). The recommended therapeutic plasma concentrations for theophylline and caffeine are about 5 to 15 and 5 to 20 mg per L, respectively. To achieve and maintain these
plasma concentrations, a loading dose of 4 to 8 mg per kg of theophylline (active base) followed by a maintenance dose of 2 to 4 mg per kg per day in two to four divided doses may be required. There exists substantial interindividual variability in the pharmacokinetic properties of theophylline; thus, it is necessary to monitor plasma concentrations and adjust the dose accordingly (saliva has been proposed as an alternative site for therapeutic drug monitoring in the preterm, with good correlation with blood levels) (35,36). Similarly, caffeine is recommended as a loading dose of 10 to 20 mg per kg of active base or 20 to 40 mg per kg of caffeine citrate salt, intravenously or orally. A maintenance dose of 2.5 to 4 mg per kg per day (or 5 to 8 mg per kg per day of caffeine citrate) is usually needed to maintain plasma concentrations of 5 to 20 mg per L of caffeine (Table 20.2). About 25% of theophylline is methylated to caffeine (37,38), with plasma theophylline-to-caffeine ratios sometimes reaching 0.30 to 0.40 at steady state. A small (3% to 8%) proportion of caffeine is converted to theophylline via CYP1A2. Thus, the overall methylxanthine effect has to account for the sum of the two drugs because both agents are pharmacologically active.
plasma concentrations, a loading dose of 4 to 8 mg per kg of theophylline (active base) followed by a maintenance dose of 2 to 4 mg per kg per day in two to four divided doses may be required. There exists substantial interindividual variability in the pharmacokinetic properties of theophylline; thus, it is necessary to monitor plasma concentrations and adjust the dose accordingly (saliva has been proposed as an alternative site for therapeutic drug monitoring in the preterm, with good correlation with blood levels) (35,36). Similarly, caffeine is recommended as a loading dose of 10 to 20 mg per kg of active base or 20 to 40 mg per kg of caffeine citrate salt, intravenously or orally. A maintenance dose of 2.5 to 4 mg per kg per day (or 5 to 8 mg per kg per day of caffeine citrate) is usually needed to maintain plasma concentrations of 5 to 20 mg per L of caffeine (Table 20.2). About 25% of theophylline is methylated to caffeine (37,38), with plasma theophylline-to-caffeine ratios sometimes reaching 0.30 to 0.40 at steady state. A small (3% to 8%) proportion of caffeine is converted to theophylline via CYP1A2. Thus, the overall methylxanthine effect has to account for the sum of the two drugs because both agents are pharmacologically active.
The methylxanthines are powerful CNS stimulants and may interact with anticonvulsants such as phenobarbital at a kinetic or a pharmacodynamic level. Babies given theophylline and phenobarbital have been shown to require higher doses of theophylline to control apnea and higher doses of phenobarbital to control seizures (39). The methylxanthines also have the potential to interact with drugs that are substrates for CYP1A2 such as cimetidine and ketoconazole.
Table 20.2 Suggested Guidelines for Respiratory Stimulants in Neonatal Apnea | ||||||||||||||||||||||||||||||||||||||||
---|---|---|---|---|---|---|---|---|---|---|---|---|---|---|---|---|---|---|---|---|---|---|---|---|---|---|---|---|---|---|---|---|---|---|---|---|---|---|---|---|
|
Choice of Methylxanthines
Caffeine and theophylline exert similar pharmacodynamic effects but may vary in their potency concerning a specific organ receptor. Moreover, the differences in their kinetic properties alter the dosing schedules and the need for therapeutic drug monitoring. Table 20.3 lists some of the differences between the two drugs. Controlled comparative trials between theophylline and caffeine indicate that although both drugs are effective in the management of apnea, more adverse effects such as tachycardia are observed with theophylline (40,41,42). Because caffeine has a more prolonged plasma half-life, the dosing schedule is less frequent and the need for therapeutic monitoring less crucial. Although frequent monitoring is advisable for theophylline, plasma caffeine measurement only if there is lack of clinical response or suspected toxicity is generally acceptable during the neonatal period (43,44). In cases of overdosing with caffeine, the prolonged drug elimination may result in sustained high plasma concentrations of caffeine for a prolonged period. However, observations suggest that caffeine plasma concentrations of up to 50 mg per L may occur with no adverse effects, whereas plasma concentrations of theophylline greater than 15 mg per L may be associated with tachycardia. Some investigators even suggest much higher doses of caffeine to achieve a therapeutic effect. This suggests that caffeine might have a wider therapeutic index relative to theophylline. In practice, caffeine has emerged as the preferred alternative in infants with apnea of prematurity.
Table 20.3 Theophylline and Caffeine in Neonatal Apnea | ||||||||||||||||||||||||||||||
---|---|---|---|---|---|---|---|---|---|---|---|---|---|---|---|---|---|---|---|---|---|---|---|---|---|---|---|---|---|---|
|
In 1998, Steer and Henderson-Smart reviewed three trials comparing theophylline and caffeine in reducing
recurrent apnea and the need for mechanical ventilation (45). There was no difference in the failure rate (<50% reduction in apnea/bradycardia) of treatment with caffeine or theophylline at 1 to 3 days (two studies) or 5 to 7 days (three studies). There was a higher rate of apnea in the standard caffeine group at 1 to 3 days [three studies, mean difference 0.398 (0.334 to 0.72)/100 minutes] but not at 5 to 7 days (two studies). Side effects, as indicated by tachycardia or feed intolerance leading to changed dosing, were lower with caffeine, relative risk (RR) = 0.17 (0.04 to 0.43). One additional study, which has been reported only in abstract form, found that mean rates of apnea (15 seconds or more) and episodes of oxygen desaturation (<85%) were no different in the 13 infants treated with theophylline than in the 11 infants treated with caffeine. However, mean rates of bradycardia were lower with theophylline at 1, 3, and 7 days after the initiation of therapy (46,47,48). In aggregate, the data favor the use of caffeine for neonatal apnea.
recurrent apnea and the need for mechanical ventilation (45). There was no difference in the failure rate (<50% reduction in apnea/bradycardia) of treatment with caffeine or theophylline at 1 to 3 days (two studies) or 5 to 7 days (three studies). There was a higher rate of apnea in the standard caffeine group at 1 to 3 days [three studies, mean difference 0.398 (0.334 to 0.72)/100 minutes] but not at 5 to 7 days (two studies). Side effects, as indicated by tachycardia or feed intolerance leading to changed dosing, were lower with caffeine, relative risk (RR) = 0.17 (0.04 to 0.43). One additional study, which has been reported only in abstract form, found that mean rates of apnea (15 seconds or more) and episodes of oxygen desaturation (<85%) were no different in the 13 infants treated with theophylline than in the 11 infants treated with caffeine. However, mean rates of bradycardia were lower with theophylline at 1, 3, and 7 days after the initiation of therapy (46,47,48). In aggregate, the data favor the use of caffeine for neonatal apnea.
Clinical Effects
Efficacy of Methylxanthines in Neonatal Apnea and weaning from Mechanical Ventilation
Table 20.4 Clinical Studies and Drug Regimens of Methylxanthines in Neonatal Apnea | ||||||||||||||||||||||||||||||||||||||||||||||||||||||||||||||||||||||||||||||||||||||||||||||||
---|---|---|---|---|---|---|---|---|---|---|---|---|---|---|---|---|---|---|---|---|---|---|---|---|---|---|---|---|---|---|---|---|---|---|---|---|---|---|---|---|---|---|---|---|---|---|---|---|---|---|---|---|---|---|---|---|---|---|---|---|---|---|---|---|---|---|---|---|---|---|---|---|---|---|---|---|---|---|---|---|---|---|---|---|---|---|---|---|---|---|---|---|---|---|---|---|
|
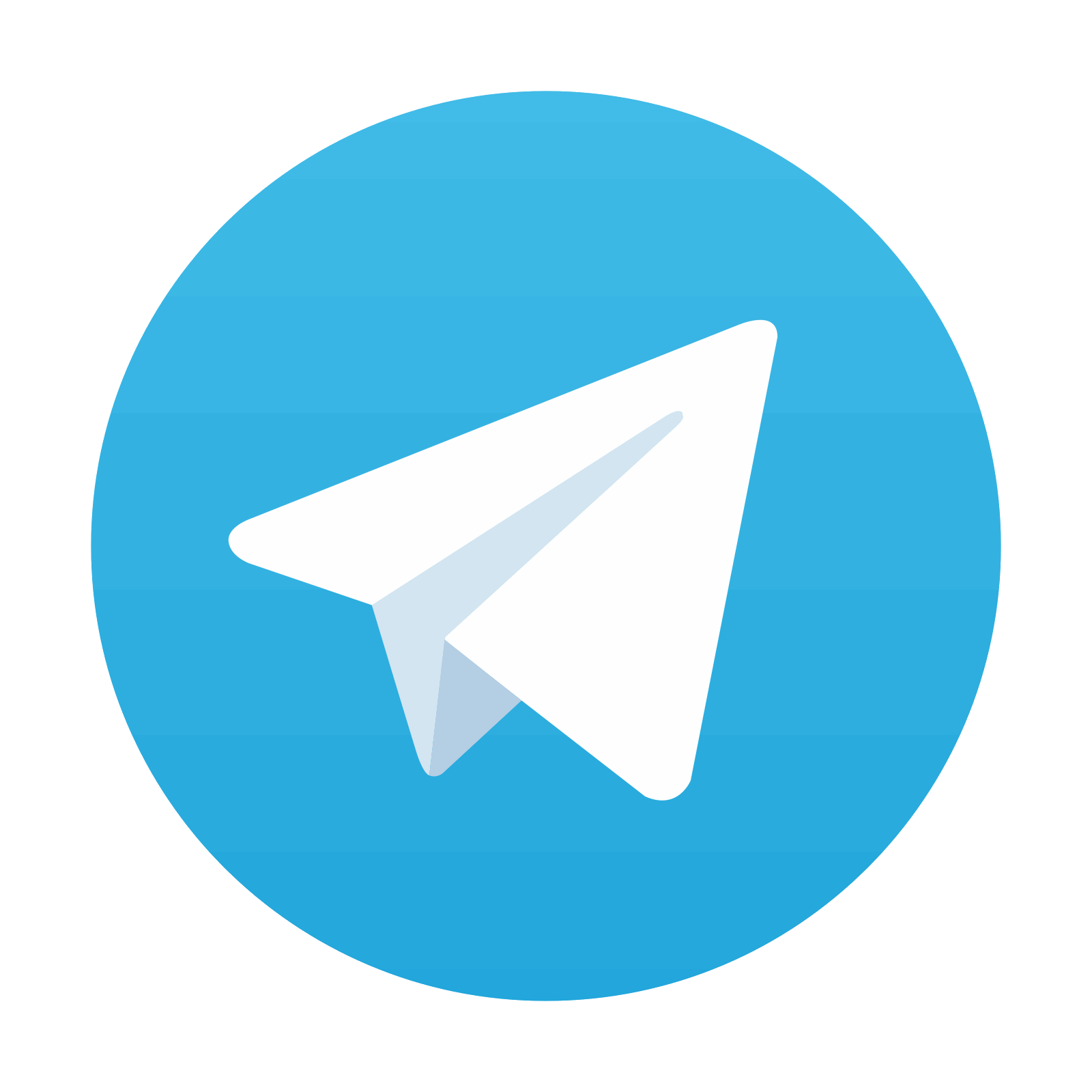
Stay updated, free articles. Join our Telegram channel

Full access? Get Clinical Tree
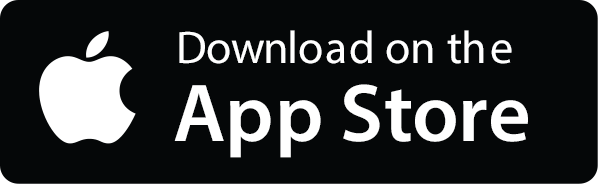
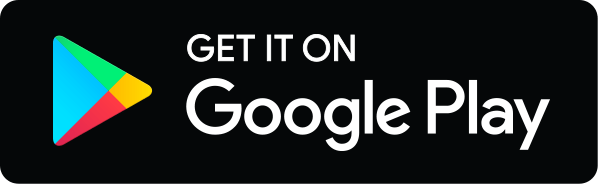