Imaging modalities that are used as adjuncts for diagnosis and therapy during pregnancy include sonography, radiography, and magnetic resonance (MR) imaging. Given rapid changes in imaging technology, this chapter is not exhaustive but serves as a guide for imaging obstetric patients with perioperative needs. The focus is on safety, especially with regard to radiation exposure. Thus, detailed dosimetry is provided to help direct examination selection and patient counseling.
Of all the major advances in obstetrics, the development of sonography for study of both fetus and mother certainly is one of the greater achievements. The technique has become virtually indispensable in everyday practice (Figs. 5-1, 5-2, 5-3).
FIGURE 5-1
A 22-year-old gravida at 9 weeks’ gestation. A. Transvaginal sonography in a sagittal view demonstrates mild inhomogeneity of the endometrium and no gestational sac. B. With evaluation of the adnexa, a normal appearing right ovary (RO) is noted and contains a corpus luteum cyst (arrowheads). C. With color Doppler imaging, characteristic peripheral vascularity, often called a “ring of fire,” is seen. D. With gentle pressure from the transducer, the intraovarian position of the corpus luteum cyst is documented.

FIGURE 5-2
A 23-year-old gravida at 12 weeks’ gestation complained of lower abdominal pain. A. Transabdominal sonography in a sagittal view shows an enlarged right ovary containing a multiseptate cyst (calipers). B. Color Doppler images demonstrate minimal vascularity within the right ovary. Importantly, absent vascular flow within an ovary is not necessary for the diagnosis of torsion. Given her compelling symptoms, diagnostic laparoscopy was performed, adnexal torsion was identified, and a right salpingo-oophorectomy was completed. Histologic evaluation revealed an ovarian serous cystadenoma.

FIGURE 5-3
A 27-year-old gravida at 15 weeks’ gestation complained of vaginal bleeding. This longitudinal image taken during transvaginal sonography shows cystic and solid heterogeneous tissue filling the endometrial cavity (calipers), and no fetal parts are identified. A complete hydatidiform mole was diagnosed histologically from a dilation and curettage specimen.

Diagnostic sonography uses sound wave transmission at certain frequencies. Recall that ultrasound is a form of nonionizing radiation that transmits energy. Studies involving prolonged ultrasound exposure of animal fetuses suggest that it is possible to induce cellular alterations. For example, with at least 30 minutes of ultrasound exposure to embryonic mouse neurons, a statistically significant number of neurons were impeded from their expected migration (Ang, 2007). At this time, however, the American Institute of Ultrasound in Medicine (2010) and other organizations agree that these findings should not alter the use of ultrasound in pregnant women. Moreover, Naumburg and associates (2000) performed a case-control study of 578 children with leukemia compared with healthy controls. In each cohort, an equal number of the children had been exposed to ultrasound in utero, which implies that sonography did not induce the cancer.
Advances in technology have introduced Doppler-shift imaging coupled with gray-scale imaging to localize spectral waveforms and superimpose color mapping (Fig. 5-4). Higher energy intensities are used with this duplex Doppler imaging. Again, however, these should have no embryo or fetal effects if low-level pulses are used (Kossoff, 1997). Even so, at very high intensities of ultrasound, there is a potential for tissue damage from heat and cavitation (Callen, 2000). However, with the low-intensity range used during real-time imaging, no fetal risks have been demonstrated in more than 35 years of use (Maulik, 1997; Miller, 1998).
FIGURE 5-4
A 37-year-old gravida with two prior cesarean deliveries complained of bleeding, and her pregnancy test was positive. A. Longitudinal transvaginal sonographic view of the uterus demonstrates heterogeneous tissue and blood clot filling the cavity and focal bulging (arrowheads) in the region of the cesarean scar. B. Further evaluation of the cesarean scar with color Doppler demonstrates significant vascularity with turbulent flow within the scar and adjacent myometrium. C. She had completed childbearing, and hysterectomy was planned. With specimen examination, a failed intrauterine pregnancy and clot filling the uterus were noted. Gross (arrowheads) and histologic analysis showed an arteriovenous malformation at the prior cesarean scar site.

Ultrasound equipment must have a video display of acoustic output to safeguard against exceeding standards set by several organizations including the American College of Obstetricians and Gynecologists (2014). Acoustic outputs are displayed as an index. The thermal index (TI) is an estimate of temperature increases from acoustic output. If the index is below 1.0, then no potential risk is expected (Miller, 1998). Adverse effects reflected in thermal index changes have not been demonstrated with Doppler use in clinical applications (Maulik, 1997). The mechanical index (MI) is used to estimate the potential risk of cavitation from heat generated by real-time imaging. As long as sonographic contrast agents are not used, there is no hypothetical fetal risk of cavitation.
Ultrasound is often the initial tool in maternal evaluation given its lack of ionizing radiation, low cost, and widespread availability. In the chest, echocardiography is a sonographic tool used to assess cardiac hemodynamic function and to evaluate cardiac morphology and its adjacent structures such as the pericardium.
In the abdomen, common indications for solid organ evaluation include abdominal and flank pain (Figs. 5-5 and 5-6), jaundice, hematuria, organomegaly, or palpable mass. Abnormal blood test results, including elevated liver function tests and creatinine levels, may also be indications for abdominal ultrasound. Typically, a limited or right upper quadrant ultrasound includes the liver, gallbladder, common bile duct, pancreas, and right kidney (Fig. 5-7). A complete abdominal ultrasound also interrogates the spleen, left kidney, and upper-abdominal portions of the aorta and inferior vena cava. Ideally, a patient has fasted prior to sonographic evaluation of the abdomen to minimize bowel gas and for adequate gallbladder distention (American Institute of Ultrasound in Medicine, 2012). A renal ultrasound focuses on the kidneys, proximal collecting systems, and urinary bladder.
FIGURE 5-5
A 31-year-old gravida at 31 weeks’ gestation complained of 6 days of right lower quadrant pain. A. A curvilinear sonography probe with lower frequency is used to evaluate deeper structures transabdominally. The relationship of the dilated appendix (arrowheads) to the uterus and fetus (F) is shown. In a different patient, gray-scale transverse (B) and longitudinal (C) images of the right adnexum demonstrate a dilated tubular structure (arrowheads). The cylinder appears blind-ending and exhibits a bowel signature, that is, alternating echogenic and hypoechoic layers in the wall. Findings suggest a dilated appendix. D. With color Doppler, the wall appeared hypervascular, further supporting the diagnosis of acute appendicitis.

FIGURE 5-6
A 29-year-old gravida with a first-trimester pregnancy complains of right flank pain. A. Longitudinal gray-scale sonographic image of the right kidney shows an echogenic shadowing stone (arrowhead) in the collecting system. B. Anechoic dilated tubular structures are seen at the renal hilum. C. Application of color Doppler shows a mildly dilated collecting system (arrowhead) in addition to hilar vessels (short arrow).


FIGURE 5-7
A 25-year-old primipara who is 14 days postpartum complains of right upper quadrant abdominal pain. A. Longitudinal gray-scale sonographic image of the gallbladder (G). There are multiple small shadowing stones (arrowhead). B. A transverse image shows a round, tense gallbladder and stones (arrow). C. Longitudinal image of the common bile duct demonstrates a prominent transverse diameter but also a shadowing stone (arrow) in the distal duct consistent with choledocholithiasis. D. Axial T2-weighted magnetic resonance image of the upper abdomen in a different third-trimester patient shows the focal signal loss of an incidentally detected gallstone (arrowhead).

Outside the abdomen and pelvis, an obstetrician may select ultrasound to evaluate superficial structures, like the thyroid gland (Figs. 5-8 and 5-9) and breasts (Fig. 32-1B, p. 504). To detect deep-vein thrombosis (DVT), compression sonography, often combined with color Doppler sonography, is the initial test currently used (Fig. 32-13, p. 518). Vascular ultrasound incorporates spectral and color Doppler to assess solid organ and peripheral vasculature. However, with a pregnant uterus, structures normally visible for sonographic evaluation may become less so, such as the abdominal aorta, pancreas, and adnexa.
FIGURE 5-8
A 31-year-old gravida at 36 weeks’ gestation is noted to have thyromegaly. A. Transverse sonographic images demonstrate a diffusely enlarged thyroid gland (arrowheads) with tiny cystic spaces throughout. T = trachea. B. Longitudinal image demonstrates normal vascularity with color Doppler, which suggests against thyroiditis. C. After delivery, a radioiodine uptake study demonstrated a normal uptake of 20.4 percent. Findings are consistent with a goiter.

FIGURE 5-9
A 23-year-old gravida in the first trimester with a palpable left neck mass and history of multiple endocrine neoplasia type 2 (MEN2) syndrome. Transverse image of the left lobe of the thyroid demonstrates a solid hypoechoic mass (arrowheads) with internal vascularity on color Doppler evaluation. Histologic analysis following resection demonstrated medullary thyroid carcinoma.

In the setting of trauma, a FAST examination—Focused Assessment with Sonography for Trauma—may be performed to look for pathologic pericardial or intraperitoneal free fluid acutely during resuscitation. Excessive abdominal fluid (blood) can be seen in the perihepatic space (Morison pouch); the perisplenic space; and the pelvis (anterior or posterior cul-de-sacs) (Fig. 17-10, p. 287). In the setting of pregnancy, ultrasound is less sensitive (61 percent) for intraabdominal injury than in nonpregnant individuals (71 percent). Nonetheless, it remains 94-percent specific for detecting injury in pregnancy with an accuracy of 92 percent (Richards, 2004).
The fetus and placenta are also evaluated sonographically with maternal trauma. Fetal biophysical assessment is done, and the placenta is examined for a retroplacental hematoma, that is, abruption (Fig. 5-10).
FIGURE 5-10
A 17-week gestation with placental abruption. A. Transabdominal sonography demonstrates an intrauterine pregnancy (F) and a placenta measuring >5 cm (arrowheads). A focal, heterogeneous hypoechoic area was identified within the placenta (asterisk). No active vascular flow was identified within this area during color Doppler evaluation. Spontaneous labor and delivery ensued. B. Most of the placenta’s basal plate was covered with clot. C. After clot is removed, the depression in the placenta made by the clot can be seen. Arrows mark the concentric crater rims of the depressions.


Inevitably, some radiographic procedures are performed prior to recognition of early pregnancy, usually because of trauma or serious illness (Fig. 5-11). Fortunately, most diagnostic imaging procedures that use ionizing radiation are associated with minimal fetal risks. However, these procedures may lead to litigation if there is an adverse pregnancy outcome. In addition, perceptions related to medical procedures that are associated with radiation exposure may lead to a needless therapeutic abortion because of patient or physician anxiety.
FIGURE 5-11
This gravida in her third trimester was involved in a high-speed motor vehicle accident (MVA). A. Maximum intensity projection image of the fetal skull acquired for maternal indications. The fetal skull fractures (arrows) are readily identified. B. 3-D reformatted computed tomography image in a bone algorithm demonstrates the fetal skeleton from data acquired during the maternal examination. Again, the arrow marks one fracture site. (Used with permission from Dr. Travis Browning.)

In 2007, the American College of Radiology began to address the growing concern of radiation doses in medicine for all patients, whether pregnant or not. One stated goal was to limit radiation exposure in any given patient through safety practices and lifelong accumulated records of exposures (Amis, 2007). Recommendations of the College task force include additional considerations for special radiosensitive populations, such as children and pregnant and potentially pregnant women. It is also suggested that the College encourage radiology groups to record all ionizing radiation times and exposures, compare them with benchmarks, and evaluate outliers as part of ongoing quality assurance programs. Currently at our institution, special recommendations are made for pregnant women. Thus, radiation exposure is recorded in high-exposure areas such as computed tomography and fluoroscopy units, with quality assurance mechanisms in place to monitor findings.
The term radiation is poorly understood. Literally, it refers to transmission of energy. Thus, it is often applied not only to x-rays, but also to microwaves, ultrasound, diathermy, and radio waves. Of these, x-rays and gamma rays have short wavelengths with very high energy and are forms of ionizing radiation. The other four energy forms have rather long wavelengths and low energy (Brent, 1999b, 2009).
Ionizing radiation refers to waves or particles—photons—of significant energy that can change the structure of molecules such as those in DNA or that can create free radicals or ions capable of causing tissue damage (Hall, 1991; National Research Council, 1990). Methods of measuring the effects of x-rays are summarized in Table 5-1. The standard terms used are exposure (in air), dose (to tissue), and relative effective dose (to tissue). In the range of energies for diagnostic x-rays, the dose is now expressed in grays (Gy), and the relative effective dose is now expressed in sieverts (Sv). These can be used interchangeably. For consistency, all doses discussed subsequently are expressed in units of gray (1 Gy = 100 rad) or sievert (1 Sv = 100 rem). To convert, 1 Sv = 100 rem = 100 rad.
Exposure | The number of ions produced by x-rays per kg of air. Unit: roentgen (R) |
Dose | The amount of energy deposited per kg of tissue. Modern unit: gray (Gy) (1 Gy = 100 rad). Traditional unit: rad |
Relative effective dose | The amount of energy deposited per kg of tissue normalized for biological effectiveness. Modern unit: sievert (Sv) (1 Sv = 100 rem). Traditional unit: rem |
The biological effects of x-rays are caused by an electrochemical reaction that can cause tissue damage. According to Brent (1999a, 2009), x-rays and gamma radiation at high doses can create biological effects and reproductive risks in the fetus. Of these actions, deterministic effects suggest that there is a threshold below which there is no risk of malformations, growth restriction, or abortions. This threshold is estimated to be less than 0.05 Gy (5 rad). That said, the true threshold for gross fetal malformations is more likely to be >0.2 Gy (20 rad), and the lower estimation is used to provide a reasonable margin of safety (Brent, 2009). A second effect, radiation’s stochastic effect, suggests that damage to a single cell may cause randomly determined probabilities of genetic diseases and carcinogenesis. In this theory, the cancer risk in radiated tissue is increased, even at very low doses.
An excellent review of ionizing radiation exposure during pregnancy was performed in exposed persons associated with the Fukushima nuclear plant disaster (Groen, 2012). This study reinforced the concept that high-level exposure is unlikely to occur with diagnostic procedures but that considerations should be made during pregnancy.
According to Wagner (1997), when calculating the dose of ionizing radiation from medical imaging, several factors are considered: (1) type of study, (2) type and age of equipment, (3) distance of the target organ from the radiation source, (4) thickness of the body part penetrated, and (5) method or technique used for the study.
Estimates of the dose to the uterus and embryo for various commonly used radiographic examinations are summarized in Table 5-2. Studies of maternal body parts farthest from the uterus, such as the head, result in a very small dose of radiation scatter to the embryo or fetus. Notably, the size of the woman, radiographic technique, and equipment performance are variable factors. Thus, data in the table serve only as a guide. When calculation of the radiation dose for a specific individual is required, a medical physicist should be consulted. In his most recent review, Brent (2009) recommends consulting the Health Physics Society website at www.hps.org to view some examples of questions and answers posed by patients exposed to radiation in the “ask the expert” section.
Study | View | Dosea/View (mGy) | Films/Studyb (No.) | Dose/Study (mGy) |
---|---|---|---|---|
Skullc | AP, PA Lat | <0.0001 | 4.1 | <0.0005 |
Chest | AP, PAc, Latd | <0.0001–0.0008 | 1.5 | 0.0002–0.0007 |
Mammogramd | CC, Lat | <0.0003–0.0005 | 4.0 | 0.0007–0.002 |
Lumbosacral spinee | AP, Lat | 1.14–2.2 | 3.4 | 1.76–3.6 |
Abdomene | AP | 1.0 | 0.8–1.63 | |
IVPe | 3 views | 5.5 | 6.9–14 | |
Hipb (single) | AP | 0.7–1.4 | 2.0 | 1–2 |
Lat | 0.18–0.51 |
As discussed, one potential harmful effect of radiation exposure is deterministic, which may result in abortion, growth restriction, congenital malformations, microcephaly, or mental retardation. These deterministic effects are threshold effects, and the level below which they are not induced is the NOAEL—the no-adverse-effect level (Brent, 2009).
The harmful deterministic effects of ionizing radiation have been extensively studied to identify cell damage that leads to embryo dysgenesis. These have been assessed in animal models. Human data stem from Japanese atomic bomb survivors and the Oxford Survey of Childhood Cancers (Sorahan, 1995). Additional sources have confirmed previous observations and provide more information. One is the 2003 International Commission on Radiological Protection (ICRP) publication of the biologic fetal effects from prenatal irradiation (Streffer, 2003). Another is the BEIR VII Phase 2 report of the National Research Council (2006) that discusses health risks from exposure to low levels of ionizing radiation.
In the mouse model, the risk of lethality is highest during the preimplantation period, namely, up to 10 days postconception (Hall, 1991). This is likely due to blastomere destruction caused by chromosomal damage. The NOAEL for lethality is 0.15 to 0.2 Gy. In some mouse models, genomic instability can be induced at levels of 0.5 Gy (50 rad), which greatly exceeds levels from diagnostic studies (Streffer, 2003).
During organogenesis in the mouse, high-dose radiation—1 Gy or 100 rad—is more likely to cause malformations and growth restriction and less likely to have lethal effects. Studies of brain development suggest that radiation affects neuronal development. Specifically, a “window of cortical sensitivity” is purported to exist in early and midfetal periods, and critical thresholds range from 0.1 to 0.3 Gy or 10 to 30 rad (Streffer, 2003).
Adverse human effects of high-dose ionizing radiation are most often quoted from atomic bomb survivors from Hiroshima and Nagasaki (Greskovich, 2000; Otake, 1987). The International Commission on Radiological Protection confirmed initial studies showing that the increased risk of severe mental retardation was greatest between 8 and 15 weeks’ gestation (Streffer, 2003). During this time, there may be a lower threshold dose of 0.3 Gy, that is, a range or “window of cortical sensitivity” similar to that in the mouse model discussed in the last section. The mean decrease in intelligence quotient (IQ) scores in humans exposed was 25 points per Gy. There appears to be a linear dose response, but it is not clear whether there is a threshold dose. Most estimates err on the conservative side by assuming a linear no-threshold (LNT) hypothesis. From their review, Strzelczyk and associates (2007) conclude that limitations of epidemiologic studies at exposures less than 0.1 Gy along with recent radiobiologic findings challenge the hypothesis that low-level radiation exposure causes adverse effects.
Finally, an increased risk of mental retardation in humans <8 weeks or >25 weeks’ gestation, even with doses exceeding 0.5 Gy or 50 rad, has not been documented (Committee on Biological Effects, BEIR V, 1990; Streffer, 2003). There are reports that describe high-dose radiation given to treat women for malignancy, heavy menstrual bleeding, and uterine myomas. In one, Dekaban (1968) described 22 infants with microcephaly, mental retardation, or both, following exposure in the first half of pregnancy to an estimated 2.5 Gy or 250 rad. Malformations in other organs were not found unless they were accompanied by microcephaly, eye abnormalities, or growth restriction (Brent, 1999b).
The implications of these findings seem straightforward. From 8 to 15 weeks, the embryo is most susceptible to radiation-induced mental retardation. It has not been resolved whether there is a threshold dose at which abnormalities occur or if a no-threshold linear model is more accurate. The Committee on Biological Effects (1990) estimates the risk of severe mental retardation to be as low as 4 percent for 0.1 Gy (10 rad) and as high as 60 percent for 1.5 Gy (150 rad). But recall that these doses are 2 to 100 times higher than those from diagnostic radiation. Importantly, cumulative doses from multiple procedures may reach the harmful range, especially at 8 to 15 weeks’ gestation. At 16 to 25 weeks, the risk is less. And again, there is no proven risk before 8 weeks or after 25 weeks.
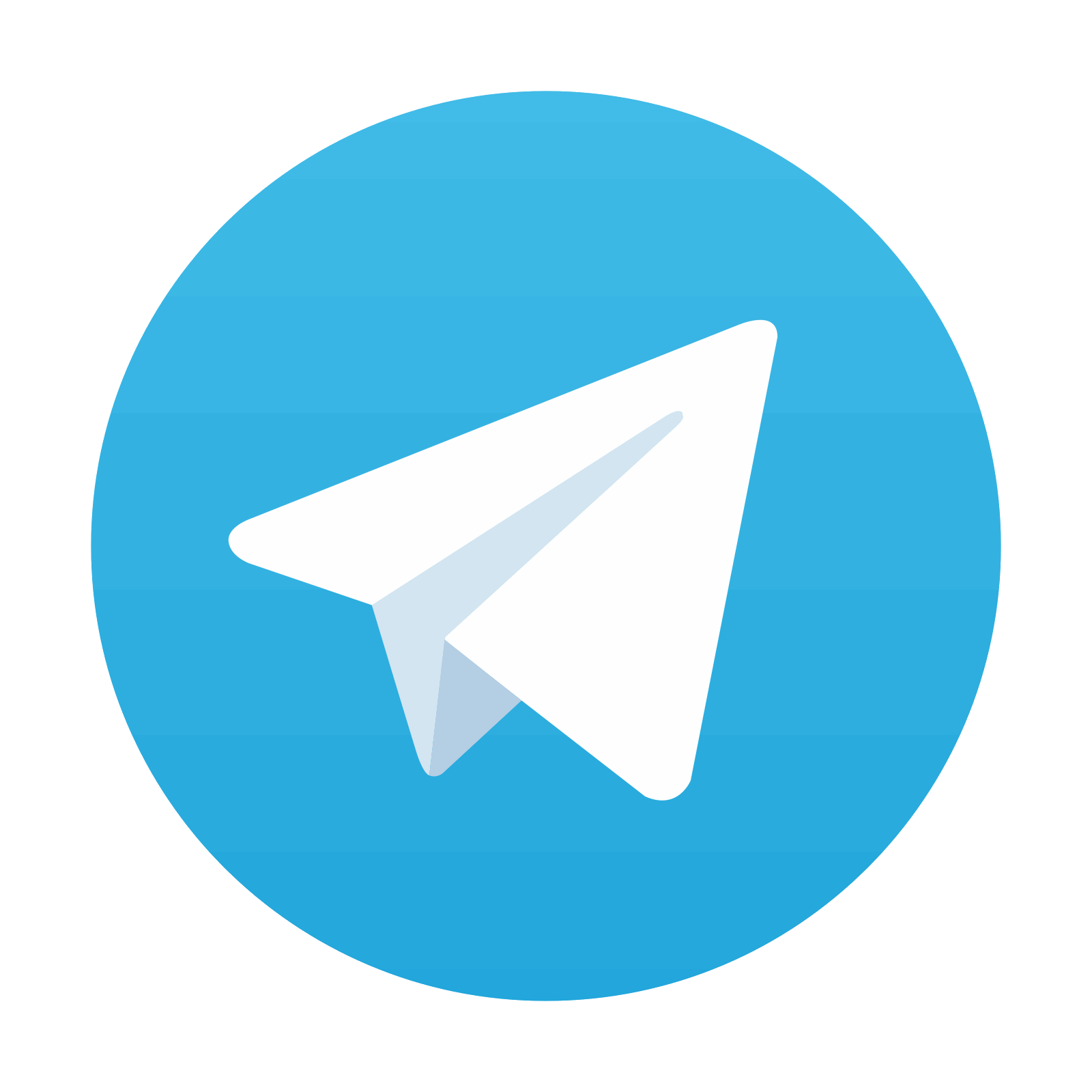
Stay updated, free articles. Join our Telegram channel

Full access? Get Clinical Tree
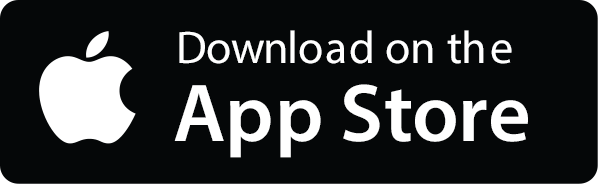
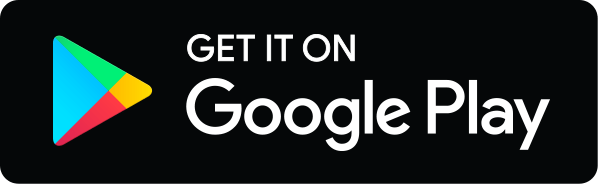