Perinatal Origins of Adult Defects in Drug Metabolism
Bernard H. Shapiro
One of the hot topics in medicine today is the so-called fetal origins of adult diseases. This intriguing topic is not so much new but rather has just begun to raise considerable interest. It has been known for decades that the fetal and early neonatal period is unique in that developmental insults during this time can result in permanent and irreversible structural, behavioral, and biochemical abnormalities. Clearly, then, any disruption in the developmental process could have repercussions throughout the life of the individual. Whereas the consequences of structural abnormalities have always been apparent, we are now starting to appreciate the effects of subtle biochemical disruptions in the developing perinate that could lead to the enhanced occurrence or early onset of certain diseases. Recent high-profile features in both biomedical and lay publications have reported that “endocrine disruptors,” that is, environmental compounds with hormonal activities, particularly estrogenic, can be teratogens, producing both permanent abnormalities in the reproductive tracts of males and females and physiologic and behavioral defects in reproductive potential and sexuality (1). Similarly, early exposure to drugs and dietary components that inadvertently disrupt endocrine differentiation could also induce malformations as well as long-lasting hormone-dependent dysfunctions (2). In this chapter, I present evidence demonstrating the plasticity of the developing drug-metabolizing enzyme system, its dependence on growth hormone, and its susceptibility to perinatal insult leading to permanent, often sexually dimorphic dysfunctions.
Delayed Teratogenic Effects
Historically, teratogens have been considered to be prenatal toxic agents that either kill the embryo or produce congenital malformations during the critical period of organogenesis. Using classical definitions, congenital malformations have been defined as anatomic abnormalities present at birth. Since its arrival as a bona fide scientific discipline, and even before that, teratology, as derived from the Greek terat-, meaning “monster,” has emphasized the study of gross anatomic anomalies. Understandably, there has been a major concern for those teratogens that produce obvious and devastating structural malformations.
However, there is now a growing awareness that many teratogens may act after the period of organogenesis, through birth and into lactation. Since a preponderance of drugs easily pass through the placental “barrier” as well as the mammary epithelium into the milk, the developing mammal remains susceptible to the teratogenic effects of maternally administered drugs from conception through fetal development and parturition and during nursing. With this awareness has come an understanding that teratogens, often at far lower doses than those needed to produce anatomic anomalies, can induce more subtle biochemical, physiologic, and behavioral defects (2). Furthermore, many of these subtle defects are not apparent at birth and may lie dormant or latent until years later. Like the classic teratogens, those compounds that interfere with normal biochemical, physiologic, or behavioral development are active at only limited critical times during development, and their adverse effects are permanent and irreversible. However, the critical fetal periods for what might be called subtle teratogens usually occur sometime after organogenesis, and, depending on the species, this may be as late as the prepubertal period. Furthermore, the effects of the teratogens are latent. That is, at the time of administration, the teratogen appears to produce a “programming” or “imprinting” defect in a developing tissue or organ. This programming defect need not be expressed until adulthood, since the “program” may not be required until that time. Consequently, in the absence of any anatomic anomalies, there is the danger that these later-onset dysfunctions will either go undiagnosed or simply be considered idiopathic.
Several alarming studies of the use of drugs by pregnant women in the 1950s, 1960s, and 1970s suggested that drug taking had become culturally fashionable. During those decades the average number of drugs taken by pregnant women in the United States was 11 (3,4,5), and their offspring now make up a large fraction of the population. Although epidemiologic surveys in the late 1980s and
1990s revealed that a disappointing 86% of women continued to consume antenatal drugs (6), the average number had declined to 4.7 (7), with the highest rate of ingestion occurring in the final trimester (8), representing the critical developmental period of biochemical differentiation (2,9). Moreover, children during the first year of life take approximately five prescription drugs per annum (10). A study by the American Psychological Association (11) reported that almost 1 million American women of childbearing age are regular, high-frequency users of psychoactive drugs (not to mention nonneuroleptics); 585,000 are regular users of barbiturates; 675,000 use prescription stimulants and appetite depressants; and about 100,000 use prescription antidepressants. The enormity of the problem is further dramatized by the fact that Americans spent $153 billion on prescription drugs during 2002, a number expected to double by the year 2010 (12). There is unlimited self-administration of over-the-counter drugs, minerals, vitamins, herbals, supplements, and metabolites to the tune of more than $2.2 billion in 2002 (13).
1990s revealed that a disappointing 86% of women continued to consume antenatal drugs (6), the average number had declined to 4.7 (7), with the highest rate of ingestion occurring in the final trimester (8), representing the critical developmental period of biochemical differentiation (2,9). Moreover, children during the first year of life take approximately five prescription drugs per annum (10). A study by the American Psychological Association (11) reported that almost 1 million American women of childbearing age are regular, high-frequency users of psychoactive drugs (not to mention nonneuroleptics); 585,000 are regular users of barbiturates; 675,000 use prescription stimulants and appetite depressants; and about 100,000 use prescription antidepressants. The enormity of the problem is further dramatized by the fact that Americans spent $153 billion on prescription drugs during 2002, a number expected to double by the year 2010 (12). There is unlimited self-administration of over-the-counter drugs, minerals, vitamins, herbals, supplements, and metabolites to the tune of more than $2.2 billion in 2002 (13).
As a possible result of its prolonged developmental period extending into early childhood (9,14), both clinical and experimental findings have shown the brain to be highly susceptible to the delayed expression of teratogens. Maternal alcohol consumption can result in retarded development, impaired learning capability (15,16), and a decreased reproductive potential (17) without obvious anatomic anomalies. Prenatal administration of morphine has been reported to alter locomotor activity (18), tolerance to the analgesic effects of morphine in adulthood (19), activity in the open-field test, pubertal onset, and the adrenal stress response (20). Moreover, the prenatal administration of D-amphetamine results in postnatal brain abnormalities in motor behavior and brain biogenic amine levels (21).
This is not to indicate that the brain is unique in its ability to exhibit latent responses to fetal insults. It has been shown from highly publicized studies in humans (22,23) and animals (24) that exposure in utero to diethylstilbestrol (DES) results in subsequent abnormalities in the reproductive structures of both female and male offspring when they become adults. In the case of female offspring, prenatal exposure to maternally administered DES (used in the 1950s through the 1970s to prevent miscarriage and premature birth) is associated with an enhanced risk of clear-cell adenocarcinoma of the vagina and cervix and a dramatic increase in abnormal vaginal cytology (22,25). Furthermore, about half of all DES daughters have less successful pregnancies (26), a greater risk of menstrual irregularities (27), and/or an increase in psychopathologies, especially depression (28), during adult life. DES sons are more likely to have testicular abnormalities, such as undescended or underdeveloped testes, gonadal cysts, and reduced sperm count (29). Some evidence indicates reproductive abnormalities in DES grandchildren (29). Two decades had to pass before the congenital defects produced by the DES teratogen were expressed. Just what DES did in utero to the approximately 2 to 4 million exposed American men and women (30) is still a matter of investigation; only the delayed consequences are now sadly clear.
Equally alarming, and perhaps more insidious, having no recognized etiology, is the dramatic increase in childhood cancers. Depending on the type, during the last 30 years there has been an 18% to 53% increase in cancers in children 4 years and younger and a 29% to 128% increase in 15- to 19-year-olds (31). In this regard, during the last 50 years we have been exposed to 75,000 new synthetic chemicals, fewer than half having been tested for potential toxicity or carcinogenicity in adults, and a fraction of which have been tested in children and pregnant women (32).
In 1979, the National Foundation–March of Dimes reported that more than 250,000 American babies, about 7% of all live births, were born with congenital defects each year (33). In agreement, the National Health Interview Survey (34) reported a 100% increase in the number of birth defects in the 25-year period starting in the late 1950s. Of relevance to this chapter is the finding that the majority of birth defects are no longer obvious malformations apparent in the newborn but represent more subtle biochemical and behavioral anomalies, such as learning disabilities, that are not diagnosed until several years after birth (34,35).
In this chapter, I discuss the latently expressed defects produced by neonatal exposure to phenobarbital and monosodium glutamate (MSG), paradigms for similar-acting compounds (2). Our laboratory is particularly interested in these compounds because of their widespread use; phenobarbital has been a commonly prescribed drug and MSG is a ubiquitous food additive (2). Moreover, neither phenobarbital nor MSG is considered a “hard” teratogen, and thus they are unlikely to produce congenital malformations (35,36). Lastly, we have reported that neonatal administration of normal exposure-like levels of both phenobarbital and MSG can produce delayed but permanent defects in hormone secretion and drug metabolism contributing to long-term, serious health consequences (2). In its broadest sense, we are investigating the unrecognized perinatal origins of adult disease.
The case for Animal Models
Imagine the difficulty of trying to identify an environmental pollutant or drug ingested for a brief critical period during pregnancy as the cause of some biochemical or behavioral abnormality expressed in adulthood in an individual seemingly free of any anatomic malformations. Who would suspect that the abnormality expressed in adulthood was actually a congenital defect? This inability even to perceive of a link between cause and effect explains why it took decades to identify in utero exposure to DES as the cause of reproductive anomalies in the exposed offspring.
Realizing, then, how profoundly important and long-lasting are the effects of development on the adult, the concept of delayed teratogenic expression seems almost intuitively irrefutable. Nevertheless, numerous drugs identified as teratogens in laboratory species appear to be harmless to the human embryo. The skeptic might recall Albert Szent-Györgyi’s comment (37) that “a drug is a substance which, if injected into a rabbit, produces a paper.” However, in defense of animal studies, it should be noted that the converse is not true. That is, all human teratogens have been shown to produce congenital defects in experimental
animals (35). Although somewhat in hindsight, we have now learned that prenatal exposure to DES produces the same reproductive defects in laboratory animals as previously reported in humans (24). Moreover, it should be noted that drugs considered as nonteratogenic in humans are so classified because they produce no observable structural defects in the newborn.
animals (35). Although somewhat in hindsight, we have now learned that prenatal exposure to DES produces the same reproductive defects in laboratory animals as previously reported in humans (24). Moreover, it should be noted that drugs considered as nonteratogenic in humans are so classified because they produce no observable structural defects in the newborn.
Our lack of knowledge regarding the causes and effects of latently expressed drug metabolism defects is more a reflection of the paucity of studies in the field than anything else. It was not until 1999 that the Food and Drug Administration (FDA) was given authority to require pharmaceutical companies to conduct target studies on pregnant women and children to learn about side effects and set proper doses. However, the FDA has relied on incentive programs to overcome the unwillingness of companies to include these targeted populations in clinical trials. By 2002, a mere 50 drugs had been reexamined, requiring that 29 be relabeled because of either adverse effects or ineffectual responses (38). Thus, under the present circumstances, assessing the long-term health consequences of perinatal exposure to drugs and environmental chemicals is almost speculative. How do we know that the increasing incidence of infertility in our population is not a delayed result of our increased in utero exposure to drugs and environmental toxins? Perhaps, certain patterns of aging that are expressed near the end of our lives are a legacy of some developmental insult. The heterogeneous influences and the time frame of our lives make it exceedingly difficult to identify latent teratogenic defects in human populations. In contrast, it seems reasonable to conduct these studies on environmentally and genetically controlled laboratory species whose life spans are sufficiently short to allow for the completion of experimental protocols. If perinatal exposure to a drug at therapeutic-like levels for that species produces long-lasting biochemical or behavioral defects in the adult offspring, it seems judicious to consider the drug as a potential threat to the unborn, requiring additional studies in alternate species and already exposed human populations.
Drug Metabolism
Two seminal discoveries in the mid to late 1970s altered our concepts of drug metabolism and rejuvenated research in the field. First, it was found that cytochrome P-450 (CYP) was not a single enzyme but a family, or, as we now know, a superfamily of hundreds of forms, having existed for several billion years and distributed across every phylum (39). It is now clear that drug-metabolizing enzymes like hexobarbital hydroxylase, benzphetamine demethylase, aniline hydroxylase, and aminopyrine demethylase are only functional descriptions, and no such enzymes exist. In reality, the activities of these monooxygenases represent the sum of activities of several forms of CYP, each with a different specificity for the substrate, which combine to contribute to the final metabolism of the drug. Thus, when we measure hexobarbital hydroxylase activity, we are actually measuring the total activities of several forms of CYP (40). This important discovery has led to now-routine investigations by pharmaceutical and academic laboratories identifying the individual CYP isoforms contributing to the metabolism of both marketed and investigational drugs.
The realization that individuals express 40 to 50 different isoforms of CYP (39) can explain the occurrence of gender differences in drug metabolism. It is not, as previously thought, that one gender may have “more” hexobarbital hydroxylase enzyme than another. Rather, male and female livers each contain many CYPs, some of which, depending on the species, may be exclusively or predominantly expressed in one sex over the other. In the case of the rat, it just so happens that the isoforms with the greatest hexobarbital hydroxylase activity are predominant in male rats (40). Although most of the studies characterizing gender differences in CYP expression have been conducted in rats, at least a half dozen sex-dependent isoforms, two identified by our laboratory (41,42), have been found in mice (39,43) and smaller numbers in other species (39,44).
In spite of the discovery of sexually dimorphic expression of CYPs in almost every species examined, it is commonly assumed that these gender differences are operative only in the rat, and similar investigations in humans would be unproductive and of little clinical value. However, such assumptions contradict a growing body of literature demonstrating gender differences in human hepatic isoforms CYP1A2, 2C19, 2D6 and the predominant 3A4 (45,46,47,48,49) and their association with gender differences in the incidence of some cancers (50,51,52), adverse drug effects (53,54,55), and even longevity (56).
The identification of endogenous regulators of drug metabolism is the second important advance in the field. Compared to other major metabolic pathways, surprisingly little is known about endogenous factors maintaining CYP homeostasis. The generally accepted role of androgens in regulating sex differences in rat hepatic monooxygenases came into question when it was reported that hypophysectomy abolished the gender differences in drug metabolism (i.e., enzymatic activities declined to female levels) and testosterone was completely ineffective in restoring the dimorphism (57,58). After almost 10 more years of investigations, growth hormone was identified as the pituitary factor responsible for maintaining the sexual dimorphisms of hepatic monooxygenases in the rat (59). More specifically, it was determined that the sexually dimorphic ultradian rhythms in circulating growth hormone maintained gender-dependent expression levels of rat CYPs (60,61). Male rats secrete growth hormone in episodic bursts every 3.5 to 4 hours. Between the peaks, growth hormone levels are undetectable. In female rats the hormone pulses are more frequent and irregular and are of lower magnitude than in male rats, whereas the interpeak concentration of growth hormone is always measurable. Exposure to the “continuous” feminine secretory profile of growth hormone produces the characteristic pattern of CYPs expressed in female rats. Conversely, the “episodic” rhythm of growth hormone secretion characterized as masculine is responsible for the expression of CYP isoforms observed in male rats and resulting in a faster rate of drug metabolism. In fact, growth hormone regulation of drug metabolism is far more exquisite. Using laboratory species, we have reported (62,63,64) that the expression or suppression of each isoform of CYP is regulated by a different “signal,” or, perhaps, a differential sensitivity
to the “signal” in the sexually dimorphic growth hormone profile. These signals are recognized by the hepatocyte in the frequencies and/or durations of the pulse or interpulse periods. Alternatively, the hepatocyte can monitor the mean plasma concentration of the hormone (62,63,64). We have observed that expression of some rat CYP isoforms is regulated by the duration of the growth hormone–devoid interpulse period, with the amount of hormone secreted in the pulses of no importance (63,64), while expression levels of other CYPs are proportional to the amplitudes of the growth hormone pulse (63), and other isoforms of CYP are regulated by the mean concentration of growth hormone (62).
to the “signal” in the sexually dimorphic growth hormone profile. These signals are recognized by the hepatocyte in the frequencies and/or durations of the pulse or interpulse periods. Alternatively, the hepatocyte can monitor the mean plasma concentration of the hormone (62,63,64). We have observed that expression of some rat CYP isoforms is regulated by the duration of the growth hormone–devoid interpulse period, with the amount of hormone secreted in the pulses of no importance (63,64), while expression levels of other CYPs are proportional to the amplitudes of the growth hormone pulse (63), and other isoforms of CYP are regulated by the mean concentration of growth hormone (62).
Just as many investigators consider gender differences in drug metabolism a “rat phenomenon,” sexually dimorphic growth hormone secretion and its regulation of drug metabolism is likewise considered to be limited to the rat. Actually, the contrary is true. Sexually dimorphic growth hormone secretory patterns have been reported in turkeys (65), sheep (66), and horses (67). We have measured and analyzed the plasma growth hormone profiles in mice and chickens (68,69) and reported that, like the female rat, female mice, and hens secrete hormone profiles characterized by more frequent peaks separated by shorter interpulse periods than do males animals of the species. Moreover, like the rat, these sexually dimorphic growth hormone profiles are responsible for the gender differences in drug metabolism and CYP expression observed in mice and chickens (70,71,72,73). Not surprisingly then, there are many reports describing sexually dimorphic growth hormone profiles in humans (74,75,76,77,78,79). In agreement with observations in other species, secretory growth hormone profiles in women compared to men are characterized by more frequent pulses, separated by elevated and briefer interpulse periods.
However, does growth hormone regulate CYP expression in humans as it does in other species? A search of the literature indicates that this question has received little attention. There are a few studies with growth hormone-deficient individuals, before and after hormone replacement therapy, which clearly show that growth hormone alone can restore drug-metabolizing enzymes to normal levels (80,81,82). Recently, we have reported, using primary human hepatocytes (82), that like other species examined, (1) growth hormone can regulate expression of human isoforms of CYP; (2) the sex-dependent secretory profiles of growth hormone can differentially regulate expression levels of some isoforms; (3) there are intrinsic sexual differences in hepatocytes of men nd women, resulting in both different levels of CYP isoforms (e.g., CYP3A4, 1A2 and 2D6) and their responsiveness to growth hormone; and (4) in agreement with most species but not the rat, growth hormone effects on CYP, although real, can be subtle and easily concealed by the heterogeneous background of human populations. Accordingly, these findings suggest, small developmentally induced defects in the endogenous human growth hormone profiles could permanently alter expression levels of CYP isoforms metabolizing cholesterol, corticosteroids, sex hormones, thyroid hormone, and prostaglandins, not to mention consumed drugs and environmental compounds. Small changes in the metabolism of these endogenous and exogenous bioactive chemicals over a lifetime could seriously affect the long-term health of the affected individual. We have demonstrated this scenario in experimental animals (discussed later) and propose that it is just as likely to occur in humans.
Anticonvulsants
For the physician, there is a particular moral dilemma of treating pregnant and nursing women whose medical conditions require drug therapy. Moreover, we are all concerned about the teratogenic effects of environmental pollutants. However, what can the individual—physician included—do? Surely, this is a societal problem requiring political remedy. The excessive use of over-the-counter medications, alcoholic beverages, cigarettes, recreational drugs, and so on during pregnancy is a serious threat to the unborn, and physicians have unequivocally condemned their excessive use. In contrast, how do you treat women whose medical conditions require drug therapy but who incidentally happen to be pregnant? Who would want to restore the health of the mother at the cost of compromising her child’s long-term well-being?
In this regard, there are drugs whose widespread use and teratogenic potential make them likely candidates for long-term birth defects studies. Approximately 1 of every 200 women is epileptic (83), and 95.7% of them are on anticonvulsant therapy (84), which is invariably continued throughout pregnancy and lactation (85,86). Moreover, approximately 1% of children and adolescents, still susceptible to developmental disruptors, are epileptic (87). In other words, about 50 million people globally are epileptic, contributing to an aggregate burden of approximately 0.5% of total world disease (88). In this regard, the evidence indicates that anticonvulsant drugs are responsible for producing a two to three times greater incidence of malformations in the children of epileptic mothers (89,90). With the exception of the fetal alcohol syndrome, anticonvulsant-induced malformations, specifically the fetal hydantoin syndrome, represent the most commonly recognized teratogen-induced malformations (91).
Phenytoin (diphenylhydantoin) is one of the most efficacious and widely used anticonvulsants in North America (92). Considering that phenytoin easily passes through the placenta into the fetal tissues and through the mammary epithelium into the milk (86,93), there has been a justifiable concern that the drug could be teratogenic. In this regard, animal studies have demonstrated clearly that prepartum administration of phenytoin can produce congenital malformations (4,35,89,92). Studies in humans (84,85,90,91) have indicated that in utero exposure to phenytoin may be responsible for inducing such anatomic malformations as cleft lip and palate, microcephaly or trigonocephaly, and various anomalies of the face and fingers, often referred to as the fetal hydantoin syndrome. In addition, there is evidence to suggest that peripartum exposure to phenytoin can produce more subtle long-term biochemical and behavioral defects at doses too low to induce structural malformations (94,95). It has been reported that in the absence of anatomic malformations, adult rats exposed perinatally to maternally administered phenytoin have several behavioral and neurologic deficits resulting in delayed motor development, persistent locomotor dysfunction, and abnormal maze learning (94,96)
as well as reduced fertility (97,98). Our findings of normal developmental profiles of serum androstenedione, testosterone, and dihydrotestosterone in phenytoin-exposed male offspring (99) suggest that the delayed reproductive dysfunctions found by others (97,98) are like the behavioral defects (94,96), a result of phenytoin’s teratogenic action on the developing brain.
as well as reduced fertility (97,98). Our findings of normal developmental profiles of serum androstenedione, testosterone, and dihydrotestosterone in phenytoin-exposed male offspring (99) suggest that the delayed reproductive dysfunctions found by others (97,98) are like the behavioral defects (94,96), a result of phenytoin’s teratogenic action on the developing brain.
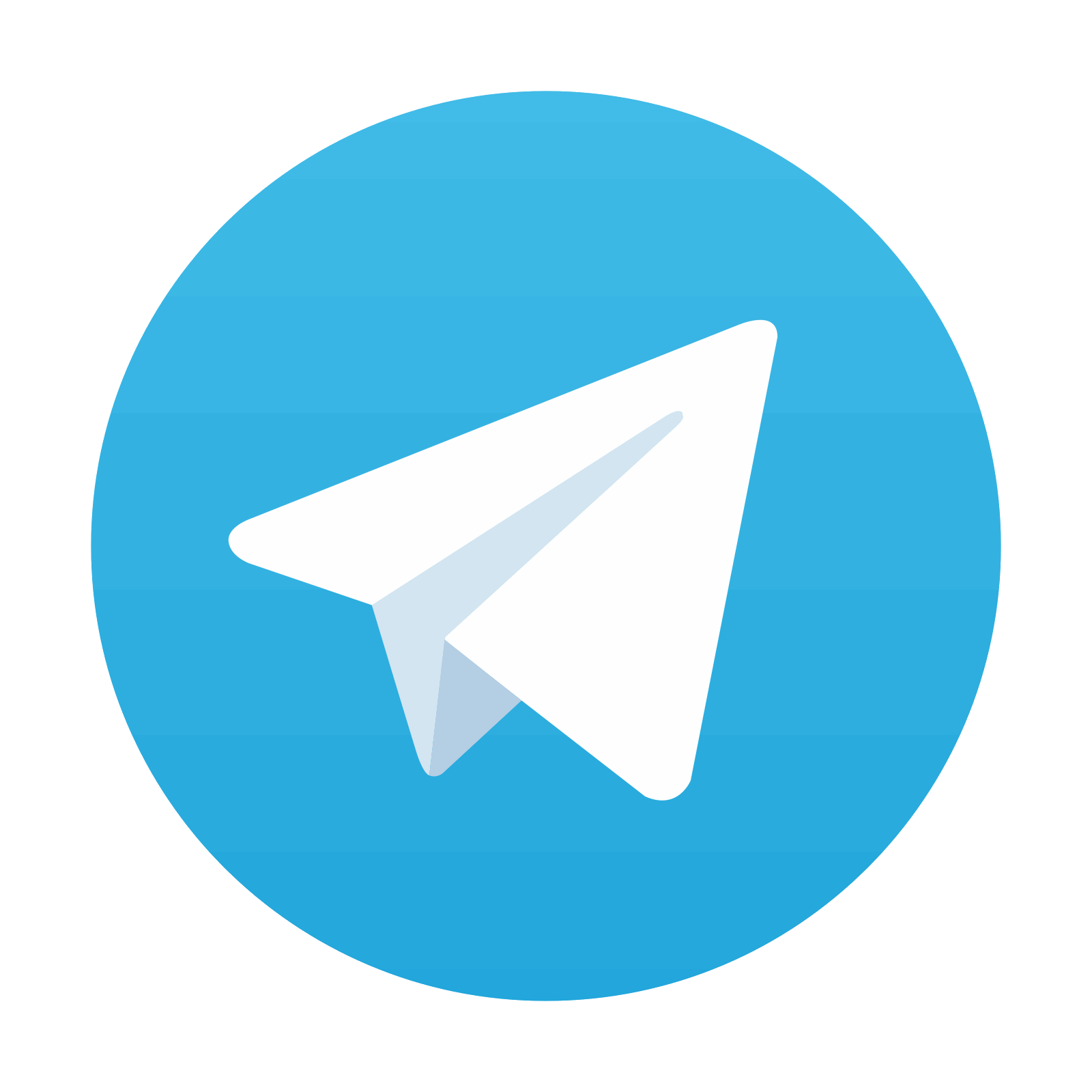
Stay updated, free articles. Join our Telegram channel

Full access? Get Clinical Tree
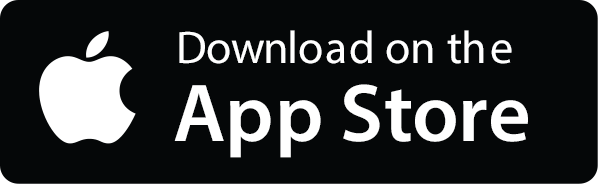
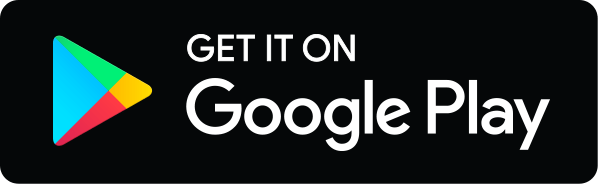