Mithilesh Kumar Lal, Nazakat Merchant, Sunil K Sinha With contributions by, Helen Yates, Lawrence Miall, Steve Byrne • Know the definitions used in perinatal medicine and their uses and limitations • Know about pre-pregnancy and prenatal conditions which may affect the fetus • Understand how multiple births may affect the fetus • Be aware of the embryology of the human fetus and how congenital anomalies may occur • Know about teratogens and how maternal drugs, alcohol and substance misuse may affect the infant • Know about maternal medical conditions that may affect the fetus • Know about congenital infections • Understand the fetal circulation and circulatory changes after birth • Know about the effect of perinatal hypoxia on the fetus and infant Even simple definitions in perinatology can be contentious. It is traditional to calculate the baby’s due date by asking the mother about the first day of her last menstrual period and relying on this, unless there is a discrepancy with ultrasound dates of more than 10 days. However, expert opinion is divided and some would advise that when results of an ultrasound dating scan are available, the due date should be derived from ultrasound biometry alone (Perinatal Institute of Maternal and Child Health recommendations). It follows that obtaining accurate data relating to stillbirths and mortality/survival rates in extremely preterm infants is difficult. Comparing data from different countries is even more difficult. This is due to variation in legislation, regulation, and practices of registration of stillbirth, live birth and death. There is wide variation between different countries in gestational age at which fetal deaths are registered as stillbirths, which influences the decision whether extremely preterm births are registered as live birth or miscarriage. Some definitions used in perinatal medicine are listed in Box 10.1. The main causes of perinatal deaths in the UK are listed in Table 10.1. Table 10.1 Stillbirths and neonatal deaths in the UK, 2013 Maternal risk factors for poor fetal outcomes in the UK include coexisting medical conditions, parity, socio-economic status, age, nutrition, antenatal service provision and their utilization. The perinatal mortality rate has decreased from 13.3 in 1980 to 6.0 in 2013 in England and Wales. Improved maternal health and nutrition, socio-economic conditions, better antenatal and perinatal care, high antenatal steroid uptake and improved survival of preterm infants have contributed. Changes in neonatal, postneonatal and infant mortality rates are shown in Figure 10.1. Globally, almost 99% of neonatal deaths occur in low- and middle-income countries and the main causes are preterm birth (34%), infection (23%), and perinatal asphyxia (25%). The epidemiology of mortality statistics and their collection is considered in more detail in Chapter 2, Epidemiology and public health, and a global overview is considered in Chapter 33, Global child health. The placenta has three major functions: transport, immunity and metabolism. The placenta starts to develop as soon as the blastocyst implants in the uterine endometrium, forming the trophoblast (Fig. 10.2). A network of umbilical blood vessels then develop and branch through the chorionic plate to form villi. On the maternal side of the placenta, the blood supply is complete by 11–12 weeks. The uterine spiral arteries dilate and straighten and bathe the intervillous space with blood. The placenta transports nutrients from the mother to the fetus, and waste products in the other direction. This occurs in a number of ways, including simple diffusion (for small molecules) and active transport of larger molecules. The placenta is crucially also responsible for gaseous exchange of O2 and CO2. Oxygen diffuses from the mother (pO2 = 10–14 KPa) to the fetus (pO2 = 2–4 KPa), where it binds to fetal haemoglobin (Hb), which has a higher affinity for oxygen than maternal Hb for a given pO2. This off-loading of oxygen from maternal Hb is also facilitated by a change in maternal blood pH. The placental trophoblast prevents the maternal immune system from reacting against ‘foreign’ fetal antigens. Rejection does not occur because the trophoblastic cells appear non-antigenic, although it is known that some fetal cells do cross into the maternal circulation where they can trigger an immune reaction (e.g. Rhesus haemolytic disease). Maternal IgG antibody, the smallest of the immunoglobulins, can cross the placenta, where it provides the newborn with innate immunity to infectious diseases. IgM immunoglobulins do not cross the placental barrier. This is of particular relevance in the diagnosis of congenital infection. The placenta is metabolically active and produces hormones, including human chorionic gonadotrophin (HCG) and human chorionic thyrotrophin (HCT). It also detoxifies drugs and metabolites. Because of its metabolic activity, the placenta has very high energy demands and consumes over 50% of the total oxygen and glucose transported across it. The placenta is not solely responsible for fetal homeostasis – the fetal liver produces albumin, red cells and clotting factors and the fetal endocrine glands produce thyroid hormone, corticosteroids and insulin from about 12 weeks. The kidney excretes large volumes of dilute fetal urine from 10–11 weeks, which contributes to amniotic fluid volume. Advice for mothers includes: Avoid alcohol ingestion and drug abuse (opiates, cocaine): These may damage the fetus. Congenital rubella: Prevent by maternal immunization before pregnancy. Eating liver: Best avoided as it contains a high concentration of vitamin A. If pre-existing maternal medical conditions, e.g. diabetes and epilepsy: Optimize control. Routine prenatal screening includes maternal blood sampling, ultrasound scanning and attendance at an antenatal clinic. Maternal blood should be tested for blood group, antibodies for rhesus (D) and other red cell incompatibilities, hepatitis B (surface and e-antigen), syphilis serology, rubella, HIV serology, haemoglobin electrophoresis to identify thalassaemia and sickle cell traits. It is usual for mothers to have late first and mid-trimester scans. The initial scan allows gestational age estimation and can identify multiple pregnancy, the later scan structural abnormalities and amniotic fluid volume abnormalities. If fetal growth or other problems are identified, monitoring with serial scans may be performed. Antenatal clinical attendance allows identification of pre-existing maternal medical condition (e.g. hypertension, HIV) or obstetric risk factors for complications of pregnancy or delivery (e.g. recurrent miscarriage or previous preterm delivery). It also facilitates monitoring for pregnancy complications. Prenatal screening for disorders affecting the mother or fetus allows: Pregnancies at increased risk of genetic disorders may be identified on prenatal or antenatal screening. Trisomy 21 (Down’s syndrome) may be suspected on first trimester ultrasound screening of nuchal translucency measurement, which may be combined with maternal serum biochemical screening. Non-invasive prenatal testing (NIPT) is where cell-free fetal DNA is obtained from maternal blood. This can now be performed for identification of fetal gender (for X-linked disorders), fetal genotyping (Rhesus) and exclusion of common aneuploidy (especially trisomy 21). It is likely to become increasingly refined and available in clinical settings. It is debated if this should be offered alone or with nuchal translucency ultrasound screening to identify trisomy 21. The advantage is that it avoids the risk of miscarriage with invasive testing procedures, however there are technical, ethical and financial issues. Invasive prenatal testing (see Fig. 10.3) requires an invasive test in pregnancy with the aim of collecting fetal cells. These can be used for: • Cytogenetic analysis to look for chromosomal abnormalities. • Molecular analysis in the case of a known genetic mutation. • Biochemical analysis in the case of a defined disorder with a suitable prenatal biochemical test. In families already affected by a genetic condition, a prenatal test is only possible where the genetic mutation in the family is known. In a small number of disorders, mainly metabolic, prenatal biochemical or enzyme testing may be possible if the diagnosis has been confirmed in an affected family member. The techniques involved in fetal medicine and their indications are shown in Figure 10.3. Examples of fetal therapy include: • Glucocorticoid therapy before preterm delivery to accelerate lung maturity and surfactant production • Digoxin or flecainide given to the mother to treat fetal supraventricular tachycardia This is performed in a few specialist perinatal centres, although outcomes have been highly variable. Some examples include: An infant’s gestation and birth weight influence the nature of the medical problems likely to be encountered in the neonatal period. In the UK, 7% of babies are of low birth weight (<2.5 kg). However, they account for about 70% of neonatal deaths. Babies with a birth weight below the 10th centile for their gestational age are called small for gestational age (SGA) or small-for-dates (SFD). The majority of these infants are normal, but constitutionally small. An infant’s birth weight may also be low because of preterm birth, or because the infant is both preterm and small for gestational age. SGA infants may have grown normally but are small, or they may have experienced intrauterine growth restriction (IUGR), i.e. they have failed to reach their full genetically determined growth potential and appear thin and malnourished. Babies with a birth weight above the 10th centile may also be growth restricted, e.g. a fetus growing along the 80th centile who develops growth failure and whose weight falls to the 20th centile. As the incidence of congenital abnormalities and neonatal problems is higher in those whose birth weight falls below the second centile (two standard deviations (SD) below the mean), some restrict the term SGA to this group of babies. In obstetric care, fetal centiles may be customized for maternal characteristics (weight, height, parity, and ethnicity) as well as gestation and gender; this is more predictive of morbidity and mortality. Intrauterine growth restriction implies a pathological restriction of genetic growth potential; this may be identified or monitored by evidence of fetal compromise, such as reduced liquor volume and abnormal Doppler waveforms. It has traditionally been classified as symmetrical or asymmetrical. In the more common asymmetrical growth restriction, the weight or abdominal circumference lies on a lower centile than that of the head. This occurs when the placenta fails to provide adequate nutrition late in pregnancy but brain growth is relatively spared at the expense of liver glycogen and skin fat. This form of growth restriction is associated with uteroplacental dysfunction secondary to maternal pre-eclampsia, multiple pregnancy, maternal smoking or may be idiopathic. These infants rapidly put on weight after birth. In symmetrical growth restriction, the head circumference is equally reduced. It suggests a prolonged period of poor intrauterine growth starting in early pregnancy. It is usually due to a small but normal fetus, but may be due to a fetal chromosomal disorder or syndrome, a congenital infection, maternal drug and alcohol abuse or a chronic medical condition or malnutrition. These infants are more likely to remain small permanently. In practice, distinction between symmetrical and symmetrical growth restriction often cannot be made. The fetus with IUGR is at risk from intrauterine hypoxia/intrauterine death and asphyxia during labour and delivery. The growth-restricted fetus should be monitored closely to determine the optimal time for delivery. Progressive uteroplacental failure results in: • Reduced growth in femur length and abdominal circumference (<10th centile) • Reduced amniotic fluid volume • Abnormal ductus venosus Doppler waveform denoting diastolic cardiac dysfunction • Abnormal CTG (cardiotocography) Risks and complications after birth include: In the healthy fetus, there is forward flow in the umbilical artery throughout systole and diastole. In IUGR due to placental disease, the resistance in the placenta increases (e.g. due to loss of placental villi or pre-eclampsia) resulting in reduced, then absent or even reversed flow during diastole (Fig. 10.4). If end-diastolic flow is absent, detailed Doppler studies of the middle cerebral artery (MCA) and ductus venosus are indicated. As IUGR becomes increasingly severe, there is redistribution of fetal blood flow, with an increase in flow to the brain, and increased end-diastolic velocity. Evidence of cerebral redistribution should trigger intensive regular monitoring. This reflects the physiological state of the right heart. In the second trimester growth-restricted fetus, reversed flow during atrial contraction represents cardiac decompensation. It is a better predictor of stillbirth than umbilical artery Doppler alone. Timing of delivery will be based on Doppler findings, gestation and estimated fetal weight.
Perinatal medicine
Perinatal definitions and epidemiology
Stillbirths (4.2 per 1000 total births)
Neonatal deaths (1.8 per 1000 live births)
Unexplained antepartum fetal death 52%
Immaturity 44%
Intrapartum 9%
Congenital malformation 22%
Congenital malformation 6%
Intrapartum causes 6%
Infection 3%
Infection 6%
Placenta 19%
Fetal 5%
Other 11%
Other 17%
Placental physiology and early embryology
Placental function
Transport
Immunity
Metabolism and homeostasis
Pre-pregnancy care
Prenatal care
Maternal blood
Ultrasound
Antenatal clinic attendance
Screening
Genetic disorders
Non-invasive prenatal testing
Invasive prenatal testing
Fetal medicine
Fetal surgery
Intrauterine growth restriction
Patterns of growth restriction
Monitoring the growth-restricted fetus
Umbilical artery Doppler
Middle cerebral artery Doppler
Ductus venosus Doppler
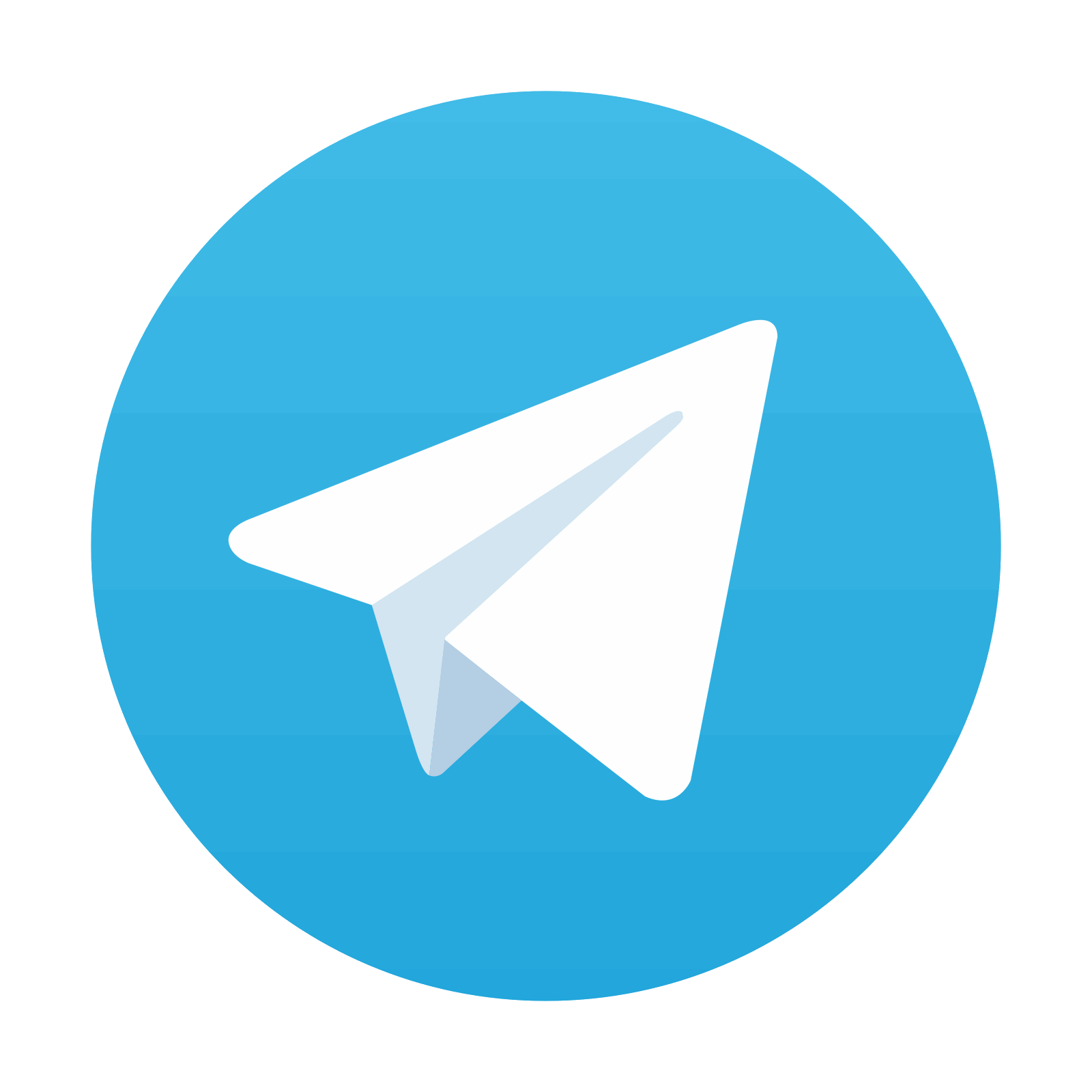
Stay updated, free articles. Join our Telegram channel

Full access? Get Clinical Tree
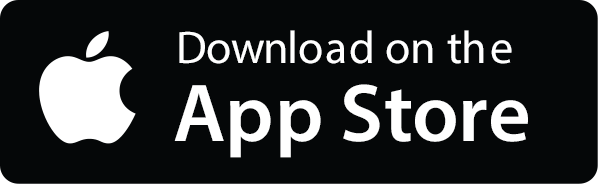
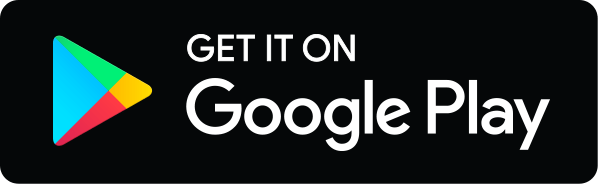