Objective
The purpose of this study was to determine whether the combined distribution of a panel of cellular messenger RNA markers can detect preeclampsia long before onset.
Study Design
We compared blood at 10-14 weeks from 11 women who ultimately experienced preeclampsia with 88 matched control subjects. After multiples of the median conversion of all the markers, logistic regression was used to calculate the risk of the development of preeclampsia.
Results
Higher multiples of the median values than expected were found for endoglin, fms-related tyrosine kinase 1, and transforming growth factor–β1. Lower multiples of the median values were found for placental growth factor and placental protein 13. Endoglin fms-related tyrosine kinase 1 and transforming growth factor–β1 had the best discriminant power. Messenger RNA species provided independent contributions to the prediction of preeclampsia. In fact, 11 women with preeclampsia scored a median risk of 50% of experiencing preeclampsia. Control subjects scored a median risk of preeclampsia of 0.18%. The detection rate at a 5% false positive rate was 72.3%.
Conclusion
The messenger RNA dosage in maternal blood would be a useful method for the calculation of the risk of the development of preeclampsia.
Hypertensive disorders in pregnancy, including preeclampsia and pregnancy-induced hypertension, are associated with significant morbidity and mortality rates for the mother and the neonate. Preeclampsia is a multisystem disorder that is unique to pregnancy and causes significant morbidity and death worldwide. It affects 2-5% of pregnancies ; the clinical features are well recognized and characteristically manifest in the second to third trimester, whereas the underlying disease and major pathogenic changes already occur at earlier stages of pregnancy because of failure of the trophoblast cells to invade, with an increase in the apoptotic and necrotic index. Because several known risk factors are not highly predictive or modifiable, attempts to prevent preeclampsia are confined mostly to those patients who are at increased risk because of their medical history. Given the morbidity that is associated with preeclampsia, an enormous variety of biomolecules have been studied to detect those that show evidence of alteration in the maternal circulation during early pregnancy, before the manifestation of clinical symptoms. The identification of reliable screening markers thus would permit major improvements in obstetric care through better targeting of antepartum surveillance. A great number of possible predictive markers have been tested, which include placental factors, abnormal fibrolytic activity, markers of endothelial dysfunction, markers of oxidative stress, and markers of abnormal trophoblast invasion. An aberrant quantitative expression of some circulating placental-specific messenger RNAs (mRNAs) in the maternal blood that was found in preeclampsia cases compared with control subjects seems to be a promising tool for the early detection of the disease. Even if there is no clear evidence that mRNAs offer some advantages over protein markers, molecular analysis has the advantage of enrolling a great number of markers at the same time and can also provide some useful information about the pathophysiologic condition of the disease. The disturbances in the expression of these molecules have led to the proposal that they may be used as early predictive markers of preeclampsia and/or intrauterine growth restriction before the onset of clinical symptoms. However, because it seems that no single test is highly sensitive or predictive, several markers might plausibly be used at the same time.
In this study, we dosed the concentration of a panel of cellular mRNAs that was comprised of fms-related tyrosine kinase 1 (FLT1), endoglin, placental growth factor (PlGF), transforming growth factor–β1 (TGFβ1), and placental protein 13 (PP13) that were circulating in maternal blood of women with preeclampsia who had been matched with appropriate control subjects, with a view to the calculation of a new posterior risk of preeclampsia for each subject in the data series.
Materials and Methods
The study population consisted of 99 women who attended the Division of Prenatal Medicine, University of Bologna, Bologna, Italy. Gestational age was calculated by ultrasound measurements at 11-14 weeks’ gestation. A second ultrasound examination was performed at 22-24 weeks’ gestation for measurement of fetal growth and examination for fetal defects. Those cases in which no major fetal defects were detected were included in the study. Eleven women with preeclampsia but who were asymptomatic at the time of blood drawing were matched with 88 control subjects (1:8 match for fetal sex and gestational age expressed in weeks + days). All women were informed and agreed to participate in the study, which was approved by the local Hospital Ethics Committee.
Preeclampsia was defined as gestational hypertension (systolic pressure of >140 mm Hg or diastolic blood pressure of >90 mm Hg on at least 2 occasions after 20 weeks’ gestation) with proteinuria (>0.3 g/d). Severe preeclampsia was defined by the presence of 1 or both of the following events: (1) severe gestational hypertension (systolic pressure of >160 mm Hg or diastolic pressure of >110 mm Hg on at least 2 occasions after 20 weeks’ gestation) and (2) severe proteinuria (≥5g protein in a 24-hour urine specimen or ≥3 g on 2 random urine samples that were collected at least 4 hours apart). Intrauterine growth restriction was defined as the estimated fetal weight being 2.0 standard deviations below the mean expected weight for the gestational age, as determined by ultrasound evaluation.
Blood samples were taken at the time of first examination (11-14 weeks’ gestation) from subjects who had been scheduled for chorionic villous sampling or amniocentesis. The blood samples (2.5 mL) were collected in PAXgene blood RNA tubes (PreAnalytic, Hombrechtikon, Switzerland), kept at room temperature for 3 hours, and then stored at –20°C until being transported to Japan. Molecular analysis was performed in the Department of Obstetrics and Gynecology at Showa University School of Medicine, Tokyo, Japan. RNA extraction and real-time polymerase chain reaction (PCR) were performed according to protocols described elsewhere. In brief, cellular component samples were centrifuged twice at 4000 g for 10 minutes at room temperature to remove the entire supernatant and any mRNA that was present in the residual plasma. The pellet was then washed, resuspended, and incubated in optimized buffer solution that contained proteinase K to digest protein. A second round of centrifugation was performed to remove any residual cell debris, and the resulting supernatant was transferred to a fresh microcentrifuge tube. We added 100% ethanol to the supernatant to adjust the binding conditions; the resultant lysate was then applied to a PAXgene spin column (PreAnalytiX; PreAnalytic), which resulted in selective binding of RNA to the silica-gel membrane of the spin column. After the column was washed 3 times, pure RNA was eluted in 80 μL of RNase-free water.
Reverse transcription of the mRNA was performed using an Omniscript RT Kit (Qiagen, Valencia, CA) according to the manufacturer’s instructions. Complementary DNA products were amplified by real-time quantitative PCR according to the manufacturer’s instructions (QuantiTect Probe PCR kit; Qiagen) with a 2-μL aliquot of complementary DNA and the kit’s components in a reaction volume of 20 μL. QuantiTect PCR analyses for vascular endothelial growth factor, FLT1, endoglin, PlGF, and TGFβ1 were performed with predeveloped and commercially available primers and probe sets (catalog no. Hs00900054_m1 for vascular endothelial growth factor; catalog no. Hs01052936_m1 for FLT1; catalog no. Hs00923997_g1 for endoglin; catalog no. Hs00182176_m1 for PlGF; and catalog no. Hs0000171257_m1 for TGFβ1; Applied Biosystems, Foster City, CA). The following thermal cycling protocol was used for PCR: initial denaturation at 95°C for 15 minutes, 40 cycles of denaturation at 94°C for 15 seconds, and annealing at 60°C for 1 minute. As an initial step, we verified that each PCR assay was specific to mRNA and not to genomic DNA. Amplification data were collected and analyzed with an ABI Prism 7900T Sequence Detector (Applied Biosystems). Each sample was analyzed in duplicate, and multiple negative water blanks were included in every analysis. Quantification of gene expression was performed with investigators blinded to the outcome of pregnancy. Amounts of mRNA samples were expressed in term of copies per milliliter. To quantify mRNA concentrations, we prepared plasmid DNA for calibration curves as previously described.
Data analysis was performed by nonparametric statistics because of the small sample size. A 1:8 match (1 case with 8 control subjects) for gestational age expressed in days, and fetal sex was determined. Such a match would guarantee a proper comparison, even in the presence of a relatively low number of cases. The median mRNA concentration of each available marker (FLT1, endoglin, PlGF, TGFβ, and PP13) as a function of increasing gestational age was measured initially and calculated by weighted log10–linear regression. All data were expressed as multiples of the median. We used a logistic regression to calculate the posterior risk of preeclampsia in both control subjects and affected cases, taking the panel of available mRNA values that were expressed in multiples of the median and parity as predictors of the disease. The detection and false-positive rates were calculated for each available marker with the use of a univariable receiver operating characteristic (ROC) curve. Multivariable analysis was performed with logistic regression to calculate the risk for each patient for classification as a control or preeclampsia case. The logistic output was adjusted for the incidence of preeclampsia in the general population (2%) by the calculation of the sampling fraction as described by Collett. Again, quantitative data were transformed into an ordinal scale (in 4 categories <25th percentile, 25-50th percentile, 50-75th percentile, and >75th percentile).
To obtain a more robust risk estimation, a multivariable ROC curve for the calculation of multivariable detection rate was built with the use of the calculated risk for preeclampsia by logistic regression analysis as the test variable, for each patient in the series.
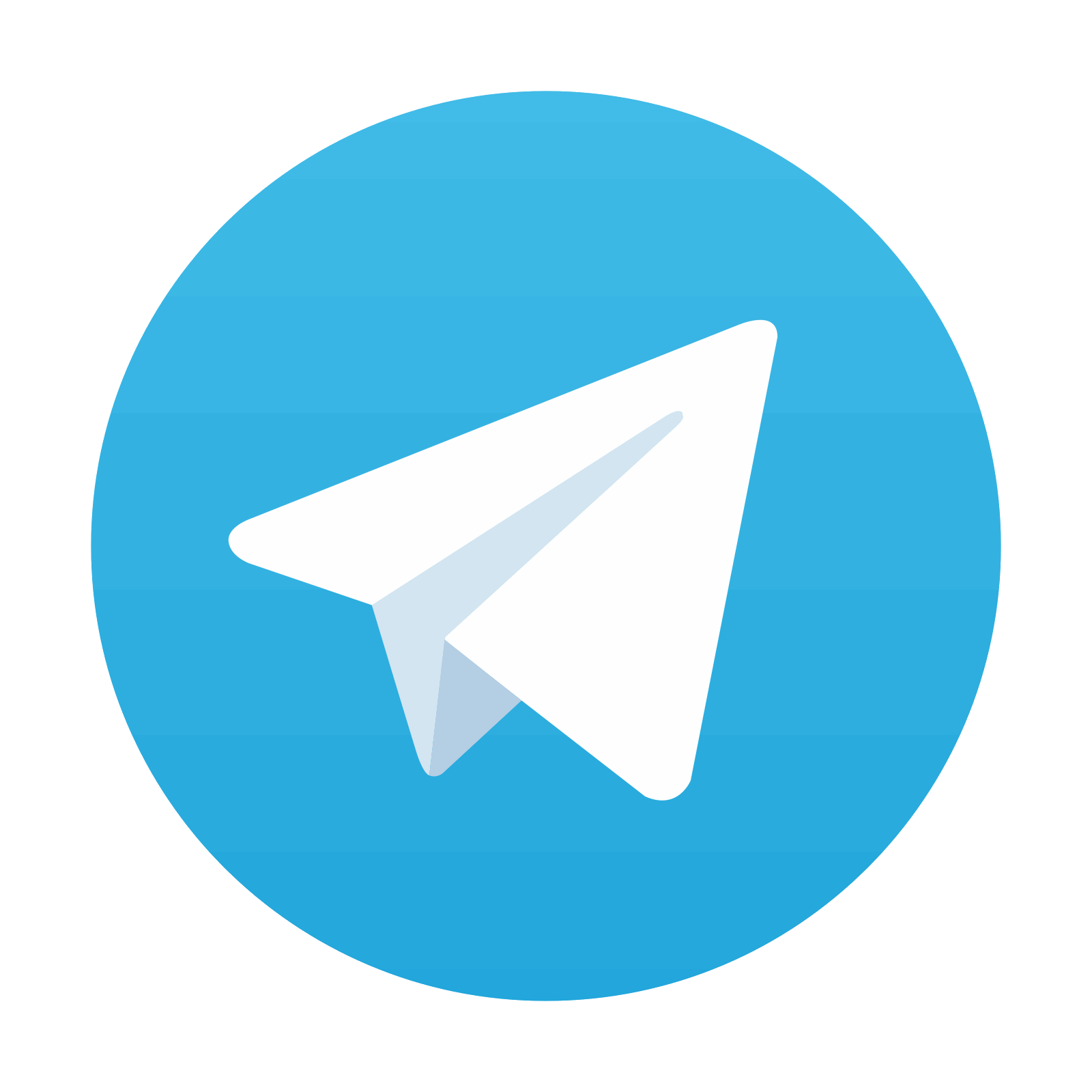
Stay updated, free articles. Join our Telegram channel

Full access? Get Clinical Tree
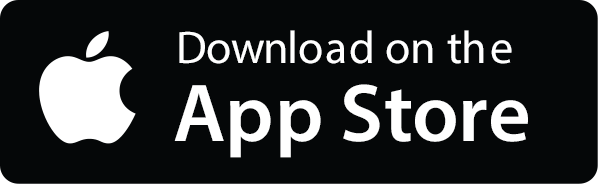
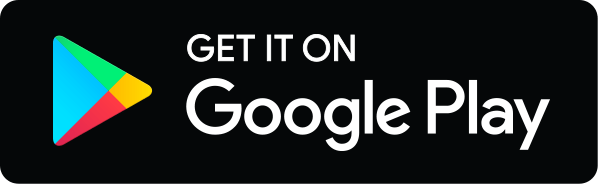